PHM2113 Module 1 (Introduction to Genetics) PDF
Document Details
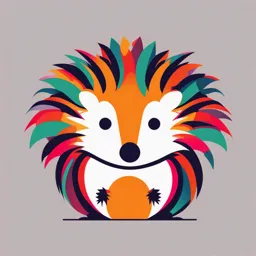
Uploaded by CleanlyBiography
University of Guyana
Tags
Summary
This document provides an introduction to genetics, covering topics such as adaptation, evolution, and Mendel's work. It discusses Darwin's theory of evolution and explores the concept of natural selection.
Full Transcript
Table of Contents Introduction to Genetics........................................................................................................ 1 Adaptation and Evolution........................................................................................................ 1 Darwin’s...
Table of Contents Introduction to Genetics........................................................................................................ 1 Adaptation and Evolution........................................................................................................ 1 Darwin’s Theory of Evolution................................................................................................. 1 Environmental Factors affecting Natural Selection............................................................... 2 Mechanisms of Natural Selection........................................................................................... 3 Gregor Mendel— A Foundation for Genetics........................................................................ 4 Mendel’s Work with Peas........................................................................................................ 5 Monohybrid Crosses................................................................................................................. 7 Principle of Dominance.......................................................................................................... 7 Principle of Segregation......................................................................................................... 8 Dihybrid Crosses....................................................................................................................... 9 Complex Inheritance Patterns................................................................................................11 Incomplete Dominance.......................................................................................................... 11 Codominance......................................................................................................................... 11 Incomplete Penetrance:......................................................................................................... 11 Complications in Inheritance................................................................................................. 12 Complex Genetic Interactions............................................................................................... 12 Introduction to Genetics The foundations of genetics trace back to ancient civilizations, including Babylonian and Greek interpretations of inheritance. However, true scientific inquiry began with discoveries related to sperm and ova by Leeuwenhoek and de Graaf in the 17th century, marking the understanding that both males and females contribute to inheritance. Charles Darwin's theory of natural selection laid the groundwork for understanding evolution, but it lacked genetic insight. Gregor Mendel's work on inheritance in peas filled this gap, establishing the foundation of modern genetics. By the 20th century, the chromosome theory of inheritance linked genetics to evolution, explaining how traits are passed on and evolve over time. Adaptation and Evolution Adaptation refers to traits that enhance an organism's survival and reproductive success. These traits are inherited and shaped by the environment. Evolution is a gradual genetic change within a population that results in phenotypic variation. Darwin’s Theory of Evolution Charles Darwin introduced the theory of evolution by natural selection in his book “On the Origin of Species by Means of Natural Selection” in 1859. Darwin made the following points in his theory of evolution: Overproduction. Organisms produce more offspring than the environment can support. This leads to a struggle for survival because resources (food, space, etc.) are limited. Competition. Individuals in a population must compete for limited resources. Only those with traits that give them an advantage in survival and reproduction are likely to succeed. Variation. Within a population, individuals have variations in traits (e.g., size, colour, speed). Some of these traits provide an advantage in the competition for survival and reproduction. Survival of the Fittest. The individuals with the most advantageous traits (those best suited to their environment) are more likely to survive and reproduce. This concept is called natural selection. "Fitness" refers to an individual's ability to survive and reproduce in a given environment. 1 Inheritance. Beneficial traits are inherited by offspring. Over generations, these advantageous traits become more common in the population, while less advantageous traits are phased out. Descent with Modification. Over time, as populations continue to adapt to their environments through natural selection, species undergo gradual changes. These changes may accumulate to the point where new species arise from common ancestors— Evolution! Variation in the gene pool (the total number of alleles in a population), consisting of different alleles (variant form of a gene on a chromosome), provides the basis for evolutionary change. Evolution occurs through changes in allele frequency (relative proportion of specific alleles in a population) in a gene pool. Fitness refers to the reproductive success of an organism’s genotype or phenotype, relative to others in the population. Higher fitness increases the likelihood of passing genes to future generations. Therefore, fitness contributes to allele frequency, thus influencing evolution. Environmental Factors affecting Natural Selection Environmental factors drive natural selection, affecting the reproductive success of individuals based on their traits. Gene Flow occurs when individuals migrate and breed in new locations, introducing new alleles into the gene pool. This causes changes in allele frequency. Genetic Drift is an evolutionary agent where certain factors reduce genetic diversity in small populations, potentially losing advantageous alleles and fixing harmful ones. o Population Bottleneck is a phenomenon where only a few individuals contribute to the gene pool, reducing genetic diversity. o Founder Effect is when a small group colonizes a new area and their limited genetic variation defines the new population. 2 Mechanisms of Natural Selection Natural selection may favour particular traits (phenotypes) resulting in an increased proportion of that selected trait in a population. 1. Stabilizing Selection: Stabilizing selection acts like a filter, promoting traits in the middle and eliminating extremes; reducing variation. The population stays centred around a specific, "optimal" trait value. Example: Human birth weight (3-4 kg) is optimal for survival, since those out of the optimal range risk complications after birth. 2. Directional Selection: Directional selection "pushes" the population in a specific direction, favouring one extreme trait, leading to gradual but noticeable change in that trait over time. Example: As humans migrated from Africa to colder, less sunny regions, darker skin was less beneficial for absorbing sunlight (needed to produce vitamin D). Lighter skin, which absorbs more sunlight, became favoured because it helped with vitamin D production in areas with lower sunlight. Over generations, people in those regions developed lighter skin due to the advantage it provided, shifting the population's average skin colour toward the lighter extreme. 3. Disruptive Selection: Favors individuals at both extremes, leading to two distinct phenotypes. Example: In a population of birds, small-billed birds are good at eating soft seeds, while large-billed birds are good at eating hard seeds. Birds with medium-sized bills are less efficient at eating either type of seed, so they have a lower survival rate. Over time, the population ends up with mostly small-billed and large-billed birds, with very few birds having medium-sized bills. When a population becomes reproductively isolated (due to geographic, behavioural, or other barriers), natural selection can lead to the formation of new species as different traits become dominant in each isolated population— speciation. Darwin’s theory had a significant weakness; he lacked a scientific understanding of the mechanism of inheritance. Darwin could not explain how traits were passed from parents to offspring, which left his theory open to critique— Mendel to the rescue! 3 Gregor Mendel— A Foundation for Genetics Key terms: Allele- An allele is one of the versions of a gene. Dominant Allele- One that produces the phenotypic character. Filial generation (Fn)- The line of progeny after the P generation. (n indicates the number of lines after the P generation. Gene- An inherited factor that determines a trait, often a segment of DNA that provides instructions for making a specific protein. Genotype- The genetic constitution of an individual. Heterozygotes- One dominant and one recessive allele. Homozygotes- Both alleles the same. Locus- Location at specific position on chromosomes. Parental generation (P)- The two individuals which produce progeny (offspring). Phenotype- The physical expression of a trait. Recessive Allele- One that is masked in the presence of its dominant partner. Known as the father of modern genetics, Mendel conducted his groundbreaking experiments on Pisum sativum (garden peas) in the 1850s, which laid the foundation for understanding how traits are inherited. His work went largely unnoticed until rediscovered in 1900. Mendel established that traits are inherited through units (now known as genes) that follow specific patterns. His three fundamental laws of inheritance are the basis of classical genetics: 1. Law of Uniformity: When two homozygotes with different alleles are crossed, all offspring in the first filial generation (F1) will be identical heterozygotes. Traits do not blend, and dominant traits are expressed in the F1 generation. 2. Law of Segregation: Each individual carries two alleles for a trait, but only one allele is passed on to offspring during reproduction. This law is foundational for understanding how single-gene inheritance works. Rare exceptions, such as chromosome nondisjunction, can cause deviations from this pattern. 3. Law of Independent Assortment: Different gene pairs segregate independently of each other when forming gametes. This applies when genes are on different chromosomes or 4 far apart on the same chromosome. However, genetic linkage can sometimes breach this rule when genes are located close together on the same chromosome. Mendel’s Work with Peas Gregor Mendel was an Austrian monk and botanist with a keen interest in how traits were passed from one generation to the next. His experiments on pea plants provided the first scientific insights into the principles of inheritance. Mendel noticed variations in traits among pea plants, which led him to conduct systematic breeding experiments. He selected seven key traits in pea plants to study, focusing on their inheritance patterns over multiple generations. Mendel's experiments primarily dealt with traits controlled by a single gene, which made the inheritance patterns easier to predict and understand. This approach is also referred to as Mendelian inheritance. Mendel conducted controlled breeding experiments (crossing) to determine how traits were passed from parents to offspring. He was able to carefully control which plants mated since pea plants have male and female parts. Some plants, like peas, can fertilize their own eggs (self- pollination); this allowed Mendel to analyse inheritance patterns when traits were passed from a single parent. Adding to which, he made several strategic choices in his experimental design that simplified the understanding of inheritance patterns: Mendel used true-breeding plants, meaning these plants consistently produced offspring with the same phenotype over successive generations when self-fertilized. 5 He focused on traits that had only two distinct phenotypes, such as tall vs. short or yellow vs. green seeds. This binary system made it easier to analyse inheritance without the complexities of intermediate or multiple phenotypes. Mendel ensured that the traits he studied were inherited the same way in both males and females. This allowed him to avoid the complications arising from sex-linked genes, simplifying his observations of inheritance patterns. A road trip backwards— Reproduction in plants Pollination is the transfer of pollen from the male parts (stamen) to the female parts (stigma) of a flower. Fertilization occurs when the sperm from pollen reaches and joins the egg inside the ovule, forming a zygote—the first cell of a new plant. Mendel Techniques Mendel used manual techniques to ensure controlled pollination: o Removing male parts. To prevent a flower from self-pollinating, Mendel removed the male parts (stamen) from certain flowers before they produced pollen. o Hand pollination. He transferred pollen manually using a tiny brush, ensuring that specific traits could be observed in offspring. Mendel Findings Mendel's findings were revolutionary but largely unnoticed during his lifetime. It was only 34 years after his death that his work became widely recognized as the foundation of genetics. His experiments laid the groundwork for the laws of inheritance, including the concepts of dominant and recessive traits. 6 Monohybrid Crosses Mendel crossed true-breeding plants that exhibited distinct traits, such as round vs. wrinkled seeds, or tall vs. short plants. In a monohybrid cross, only one gene and its two alleles are considered. The following are examples of monohybrid crosses, where A represents a dominant allele and a represents a recessive allele: AA × AA: All offspring are homozygous dominant (AA). Aa × AA: Half of the offspring are heterozygous (Aa) and half are homozygous dominant (AA). Aa × Aa: This cross produces offspring in a 1:2:1 ratio (1 AA, 2 Aa, 1 aa), with a 3:1 phenotypic ratio for the dominant trait. AA × aa: All offspring are heterozygous (Aa), showing the dominant phenotype. Aa × aa: Half of the offspring are heterozygous (Aa) and half are homozygous recessive (aa). aa × aa: All offspring are homozygous recessive (aa). Principle of Dominance Mendel’s experiments led to the concept of dominance, where one allele can mask the expression of another. For example: Tall (T) is dominant over short (t). Round (R) seeds are dominant over wrinkled (r) seeds. Yellow (Y) seed colour is dominant over green (y). Dominance means that in a heterozygous organism (e.g., Tt), only the dominant trait (tall) is expressed, while the recessive trait (short) is hidden; recessive traits only appear in homozygous recessive individuals. The Punnett square is a grid used in genetics to predict the possible genotypes of offspring based on the parental genotypes. Male gametes (sperm) are listed across the top, and female gametes (egg) down the side. Each box is filled by combining the alleles from both parents, showing the potential diploid genotype of the offspring. 7 Mendel found that traits did not blend; instead, they remained distinct across generations. In the F2 generation after breeding parents of different homozygous allele, both the dominant and recessive traits reappeared, disproving the theory of blending inheritance. Principle of Segregation Segregation refers to the separation of the two alleles (one from each parent) that determine an organism's phenotype during gamete formation. Diploid organisms (like pea plants) have two alleles of one gene. Gametes, however, are haploid, meaning they only carry one allele (e.g., A or a). During meiosis, homologous chromosomes (and the alleles they carry) separate, ensuring each gamete receives only one allele per locus. This creates equal chances for the dominant and recessive alleles to combine in offspring. 8 Test Cross (Back Cross) Test Crosses are used to determine whether an individual showing a dominant trait is homozygous (SS) or heterozygous (Ss). The individual showing the dominant phenotype is crossed with a homozygous recessive individual. If the tested individual is homozygous dominant (SS), all offspring will display the dominant trait (e.g., Ss offspring). If the tested individual is heterozygous (Ss), approximately half the offspring will display the dominant trait (e.g., Ss), and the other half will display the recessive trait (e.g., ss). Mendel studied traits controlled by single gene pairs at specific loci (e.g., plant height, seed colour). These gene pairs behave independently (leading to genetic diversity), and each gene controls a distinct trait— law of segregation. Dihybrid Crosses This is a genetic cross considering two different genes, each with two alleles. Possibilities of dihybrid crosses with the alleles A, a, B, and b include: AABB × AAbb Aabb × aaBB AaBB × AaBb Aabb × AABB Gregor Mendel’s dihybrid crosses with seed shape and seed colour in pea plants provided foundational principles for understanding how traits are inherited. Seed shape: Smooth seeds (W) are dominant over wrinkled seeds (w). Seed colour: Yellow seeds (G) are dominant over green seeds (g). 9 A true-breeding pea strain with smooth yellow seeds has the genotype WWGG. A strain with the recessive phenotype of wrinkled green seeds has the genotype wwgg. A cross between these two strains (WWGG × wwgg) results in the F1 generation, where all offspring have smooth yellow seeds with the genotype WwGg (double heterozygotes). Mendel’s dihybrid cross between F1 plants with the WwGg genotype resulted in the following: Four types of gametes are produced in equal proportions: WG, Wg, wG, wg. These gametes combine randomly, producing nine different genotypes in the F2 generation. However, there are only four phenotypes: 1. Smooth yellow 2. Smooth green 3. Wrinkled yellow 4. Wrinkled green Wrinkled yellow and wrinkled green are considered recombinant phenotypes because they represent new combinations of traits not seen in the parental generation. 10 Complex Inheritance Patterns Mendel's initial studies used traits exhibiting simple dominance (e.g., yellow vs. green seeds). Dominant alleles completely mask the expression of recessive alleles (e.g., yellow (Y) is dominant over green (y)). Incomplete Dominance This occurs when the phenotype of heterozygotes is intermediate between the two homozygous phenotypes. For example, light purple eggplants from dark purple (PP) and white (pp) parents. In the F1 generation (Pp) shows light purple fruit, and the F2 generation results in a 1:2:1 ratio of phenotypes (1 dark purple, 2 light purple, 1 white); there is a mix of colours! Codominance In codominance, both alleles are fully expressed. Human blood types are a classic example: Type A (dominant A allele) and Type B (dominant B allele) can coexist in Type AB blood (heterozygous). Type O blood is recessive and only expressed when homozygous (OO). Incomplete Penetrance: Some dominant alleles may not always manifest in the phenotype, leading to incomplete penetrance. For example, mutations in the BRCA1 gene increase the risk of breast and ovarian cancer but do not guarantee disease development (70% penetrance). 11 Complications in Inheritance 1. Multiple Alleles. Traits can be influenced by multiple alleles at a single locus, complicating inheritance patterns. For example, rabbit coat colour is determined by the C gene, which has four alleles: dark grey (C) (wild-type), albino (c), chinchilla (cch), and Himalayan (ch). This gene follows Mendelian principles but exhibits a dominance hierarchy, with wild- type being completely dominant. 2. Lethal Alleles. Some alleles can cause lethality, impacting inheritance ratios. For instance, the yellow coat colour in mice is dominant, but homozygous yellow (YY) mice do not survive, leading to a 2:1 ratio of surviving offspring (Yy). Huntington’s disease is a rare example of an autosomal dominant lethal condition where one copy of the allele leads to a fatal disorder. Complex Genetic Interactions 1. Gene Interactions and Phenotypes. Many phenotypes result from the interaction of multiple genes, complicating inheritance patterns. For example, the colour of bell pepper is determined by two genes, R and C. A cross between homozygous red (RRCC) and homozygous green (rrcc) yields all heterozygous red offspring (F1). F2 generation displays a 9:3:3:1 phenotypic ratio for colour (red, brown, yellow, green), illustrating independent assortment and dihybrid inheritance. 12 2. Epistasis. One gene masks the expression of another gene at a different locus, with both genes inherited independently. For example, mice coat colour. The normal fur colour, characterized by grey or brindled fur due to banding on individual hairs. A (dominant) produces banded hairs (agouti phenotype), aa (homozygous recessive) results in un-banded black fur, B (dominant) allows normal pigment production, and bb (homozygous recessive) blocks all pigment production, leading to albino mice regardless of the A locus genotype. 3. Pleiotropy. A single gene can influence multiple, seemingly unrelated phenotypes. For example, Phenylketonuria (PKU). A defect in one gene leads to intellectual disability and other traits like light hair and skin issues. 13