Biology Evolution Chapter 8: Gene Pool, Genetic Drift, and Adaptation - PDF
Document Details
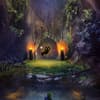
Uploaded by iiScholar
Arizona State University
Tags
Related
- Chapter 23: The Evolution of Populations PDF
- Lecture 11 Mechanisms of Evolution & Sexual Selection PDF
- OCR (A) Biology A-level Genetics and Evolution Notes PDF
- Lecture 10: Reproductive & Genetic Species Concepts 2022 PDF
- Lab 16: Population Genetics and Evolution (MK Fall 2024) PDF
- Biology SN2 Lecture 3: Microevolution PDF
Summary
This biology document delves into the principles of evolution, exploring concepts such as gene pools, genetic drift, and the Hardy-Weinberg principle. Various factors influencing these processes are examined, providing a foundational understanding of how populations evolve.
Full Transcript
Introduction The genetic makeup of a given population of organisms can change over time. This change in genetic makeup can be driven by [various factors](javascript:void(0)) and results in **evolution** of the population. This lesson covers conditions in which a population's genetic makeup (ie, ge...
Introduction The genetic makeup of a given population of organisms can change over time. This change in genetic makeup can be driven by [various factors](javascript:void(0)) and results in **evolution** of the population. This lesson covers conditions in which a population's genetic makeup (ie, gene pool) would remain unchanged over time as well as factors that would cause a population to evolve. The concept of evolutionary fitness is also addressed. 8.1.01 Gene Pool A biological [population](javascript:void(0)) is made up of all members of a given [species](javascript:void(0)) that live within the same geographical area. For example, all largemouth bass fish (*Micropterus salmoides*) living in a single lake represent a population. The members of a population have the potential to successfully interbreed; therefore, the population shares a common collection of genetic information, which is referred to as the population\'s **gene pool**. The [gene pool](javascript:void(0)) is composed of all [alleles](javascript:void(0)) of all genes present in the individuals that make up the population. Populations with more genetically diverse gene pools are typically more resilient and can adapt to environmental changes more successfully than populations with less genetic diversity, as shown in Figure 8.1. Chapter 8: Evolution 300 **Figure 8.1** Effect of genetic diversity on a population\'s resiliency to environmental changes. 8.1.02 Hardy-Weinberg Principle **Evolution** occurs in a population when the frequencies (ie, relative abundances) of alleles in the population\'s gene pool change over time. However, according to the **Hardy-Weinberg principle**, allele and genotype frequencies of a population will remain *constant* over time if certain conditions are met (Figure 8.2). Populations that meet these conditions are said to be in **Hardy-Weinberg equilibrium** and are *not* evolving. For a population to be in Hardy-Weinberg equilibrium, the following conditions must be met: Matings within the population must occur randomly. A diagram of different colors of pills AI-generated content may be incorrect. Chapter 8: Evolution 301 No net mutation can occur (ie, mutation cannot create or destroy alleles). No migration can occur into or out of the population. The population must be very large. No natural selection can occur (ie, all genotypes must have equal reproductive success). **Figure 8.2** Conditions for a population to be in Hardy-Weinberg equilibrium.  Chapter 8: Evolution 302 When a population is in Hardy-Weinberg equilibrium, two equations can be used to predict the population\'s allele and genotype frequencies: *p* + *q* = 1: Applies to a gene with two alleles (eg, A and a), where *p* represents the frequency of one allele (A), and *q* represents the frequency of the other allele (a). *p*2 + 2*pq* + *q*2 = 1: Can be used to calculate genotype frequencies (ie, frequencies of AA, Aa, and aa) from the allele frequencies. Here, *p*2 and *q*2 represent the frequencies of the two [homozygous](javascript:void(0)) genotypes AA and aa, respectively. The heterozygous genotype (Aa) frequency is represented by 2*pq*. **Concept Check 8.1** In an isolated population of 100,000 largemouth bass (*Micropterus salmoides*), 5,000 are homozygous for a mutant recessive allele. Assuming the population is in Hardy-Weinberg equilibrium, what percentage of largemouth bass in the population are heterozygous for this mutant allele? [**Solution**](javascript:void(0)) 8.1.03 Genetic Drift **Genetic drift** is the random fluctuation in allele frequencies that occurs in a population due to **chance events** (Figure 8.3). Such events (eg, earthquakes, floods, fires) can result in the death of organisms regardless of their genetic makeup, causing random loss of alleles from the gene pool. In general, small populations are [much more susceptible](javascript:void(0)) to genetic drift than large populations because small populations typically contain fewer unique alleles in their gene pools. Genetic drift reduces genetic diversity in small populations by randomly eliminating alleles from their already small collection of alleles. Therefore, rare alleles are much more likely to be completely lost from a gene pool via genetic drift in a small population than in a large population. A blue check mark in a square AI-generated content may be incorrect. Chapter 8: Evolution 303 **Figure 8.3** Genetic drift. The **bottleneck effect** (Figure 8.4) is observed in a population when genetic drift occurs following a drastic reduction in the size of the population due to a sudden, unpredictable event (eg, flood, earthquake, human-induced catastrophe). During a bottleneck event, members of a population are eliminated randomly because no particular genotype or phenotype typically confers greater survivability to an organism. Because the effect of genetic drift on a population is random, a bottleneck event does not necessarily cause a population to become better adapted to its environment. For example, a *beneficial* allele can be completely eliminated from a population\'s gene pool if no members carrying this allele happen to survive the bottleneck. Furthermore, rare harmful alleles can become *more* prevalent in a population if most members carrying a harmful allele randomly survive the bottleneck and most members carrying a wild-type allele do not.  Chapter 8: Evolution 304 **Figure 8.4** Bottleneck effect. 8.1.04 Gene Flow Movement of individuals (or gametes) among different populations of a species can alter the allele frequencies of these different populations. Such movement of alleles into or out of a population via migration is called **gene flow** (see Figure 8.5). Individuals that migrate into a population can bring new alleles and therefore increase the genetic diversity of the receiving population. However, if migration occurs in both directions between two populations, gene flow tends to cause the gene pools of the two populations to mix and the genetic makeup of the two populations to become more similar. **Figure 8.5** Gene flow. A diagram of a graph showing different types of frogs AI-generated content may be incorrect.  Chapter 8: Evolution 305 8.1.05 Natural Selection According to the theory of **natural selection** (see Figure 8.6), organisms with traits that make them well suited to their environment are more likely to survive and reproduce (ie, pass on their alleles) than organisms with less favorable traits. This tendency causes beneficial alleles to [become more common](javascript:void(0)) and detrimental alleles to become less common in a population over time. As a result, traits that increase an organism\'s likelihood of survival and reproduction prevail in a population over time, causing the population to become better adapted to its environment. However, patterns of evolution often vary depending on the [selective pressures](javascript:void(0)) faced by different species. If two species inhabit similar environments, they often evolve analogous (comparable) characteristics in response to facing comparable selective pressures (eg, habitat, food availability, predation). In contrast, if two species inhabit distinct environments with different selective pressures, they are likely to evolve unique traits best suited to their different environments. **Figure 8.6** Natural selection. A group of frogs in water AI-generated content may be incorrect. Chapter 8: Evolution 306 8.1.06 Evolutionary Fitness [Charles Darwin\'s](javascript:void(0)) theory of natural selection is often summarized by the phrase \"the survival of the fittest,\" which can lead to the incorrect conclusion that evolutionary fitness is primarily exhibited in organisms that possess physical strength or superior fighting ability. Rather than being limited to characteristics such as size and strength, **evolutionary fitness** is measured in terms of an organism\'s **reproductive success**, as shown in Figure 8.7. *Any* characteristic that promotes an organism\'s ability to survive and successfully reproduce contributes to its evolutionary fitness, and alleles responsible for such characteristics increase in frequency over time in a gene pool. **Figure 8.7** Evolutionary fitness.  **Evolution of Species** Introduction A [biological species](javascript:void(0)) can be defined as a group of organisms whose members have the potential to successfully interbreed (ie, [reproduce sexually](javascript:void(0))) in nature to produce fertile offspring. In general, members of different species cannot mate with each other and produce viable, fertile offspring. Consequently, biological species are typically marked by [reproductive isolation](javascript:void(0)). This lesson covers factors that influence the extent to which populations are reproductively isolated, factors that influence the adaptation of species, and the concept of evolutionary time. 8.2.01 Species Breeding Members of all [populations](javascript:void(0)) of a given species have the potential to interbreed. However, different populations can be more or less isolated from each other, which affects the likelihood of mating between members of those populations. **Inbreeding**, which involves mating of individuals with shared ancestry, typically occurs in small, isolated populations. Inbreeding causes an increase in [homozygosity](javascript:void(0)) of alleles in a population, which can result in inbreeding depression (ie, reduced [fitness](javascript:void(0))) due to the expression of harmful recessive alleles in homozygotes. Conversely, **outbreeding** (ie, mating of unrelated individuals) tends to increase genetic diversity in populations and is facilitated by large population size and [gene flow](javascript:void(0)) between populations, as shown in Figure 8.8. **Figure 8.8** Effects of inbreeding and outbreeding. A diagram of a dog AI-generated content may be incorrect. Chapter 8: Evolution 308 8.2.02 Species Hybridization Mating between two different species may result in **hybrid offspring**, some of which may be [inviable](javascript:void(0)) (ie, unable to survive) or infertile. When two different species cannot produce viable or fertile offspring by interbreeding, they are considered reproductively isolated. Reproductive isolation typically results from barriers that fall into two main categories: **prezygotic barriers** and **postzygotic barriers**. [Prezygotic barriers](javascript:void(0)) prevent mating or, if mating does occur, interfere with successful [fertilization](javascript:void(0)), thus preventing the formation of hybrid zygotes. Table 8.1 summarizes the prezygotic mechanisms that contribute to species\' reproductive isolation. **Table 8.1** Prezygotic isolating mechanisms. **Mechanism** **Description** Temporal isolation Species mate during different years, in different seasons, or at different times of the day Habitat isolation Species live in the same geographical area but occupy different habitats and do not interact Behavioral isolation Species engage in particular courtship rituals or produce specific signals to attract mates Mechanical isolation Anatomical differences between species prevent successful mating Gametic isolation Sperm and eggs of different species cannot successfully fuse to accomplish fertilization [Postzygotic barriers](javascript:void(0)) do not function until *after* hybrid zygotes have been successfully produced. Postzygotic reproductive isolation occurs when species produce hybrid offspring that are inviable or infertile. Table 8.2 summarizes the postzygotic mechanisms that contribute to reproductive isolation. **Table 8.2** Postzygotic isolating mechanisms. **Mechanism** **Description** Hybrid inviability The genetic makeup of hybrid offspring causes impaired development and death Hybrid sterility Hybrid offspring may develop normally but are unable to reproduce successfully Hybrid breakdown First-generation hybrids are viable and fertile, but second-generation hybrids are inviable or sterile If hybrid offspring successfully mate with members of either parental species, **genetic leakage** may occur (see Figure 8.9). Genetic leakage is the transfer of genetic information (ie, [genes](javascript:void(0))) between different species. Chapter 8: Evolution 309 **Figure 8.9** Genetic leakage. 8.2.03 Species Adaptation [Natural selection](javascript:void(0)) is an [evolutionary mechanism](javascript:void(0)) in which **adaptive traits** that increase the fitness (ie, reproductive success) of an organism are more likely to be passed to the next generation than less favorable traits. This tendency causes beneficial traits (those encoded by adaptive [alleles](javascript:void(0))) to become more common and detrimental traits to become less common over time. There are multiple types of natural selection. One type, [disruptive selection](javascript:void(0)), favors phenotypic extremes and tends to eliminate intermediate phenotypes from a population. Disruptive selection can result in **polymorphism** within a species, or when two or more different phenotypic forms (ie, morphs) are maintained in a population, as depicted in Figure 8.10.  Chapter 8: Evolution 310 **Figure 8.10** Different phenotypic forms of *Neochromis omnicaeruleus*. **Adaptive radiation** occurs when natural selection results in rapid diversification of a single species into multiple forms adapted to exploit different environmental resources. As shown in Figure 8.11, adaptive radiation reduces intraspecific competition (competition for resources by members of a single species). Reduced intraspecific competition improves fitness for the entire species because each subgroup has a role within an ecological community (ie, [niche](javascript:void(0))) that is different from the rest of the subgroups. Adaptive radiation can eventually lead to [speciation](javascript:void(0)), or the formation of new species, if the subgroups diverge enough to become reproductively isolated from each other. A group of fish with different colors AI-generated content may be incorrect. Chapter 8: Evolution 311 **Figure 8.11** Adaptative radiation. 8.2.04 Evolutionary Time The **neutral theory of molecular evolution** states that most genetic [mutations](javascript:void(0)) are [neutral](javascript:void(0)), which means they do not affect the fitness of an organism. Within species, neutral mutations are randomly fixed or lost due to [genetic drift](javascript:void(0)) and occur at fairly constant rates over time. After one species diverges to form two separate species, the accumulation rate of neutral mutations in a region of homologous DNA is mostly similar in both species. Because the number of neutral mutations tends to increase at a constant rate, accumulation of these mutations can serve as a molecular clock of evolution. The **molecular clock model** (see Figure 8.12) allows researchers to measure **evolutionary time** by analyzing random changes in the genome over time. The molecular clock model assumes that species that diverged more recently from a common ancestor have accumulated fewer mutations over a shorter time and are more genetically similar to one another (ie, more [closely related](javascript:void(0))). In contrast, species that diverged less recently (ie, longer ago) from a common ancestor have accumulated more mutations across a longer period and are less genetically similar to one another (ie, less closely related).  Chapter 8: Evolution 312 **Figure 8.12** Molecular clock model. A graph showing different animals and fish AI-generated content may be incorrect. **Have you mastered this Lesson?** Mark this lesson as complete and continue to the next. Mark as Complete