Muscle and Nervous Tissues PDF
Document Details
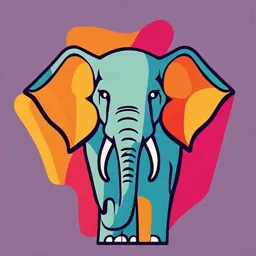
Uploaded by BestSellingAnemone
Tags
Summary
This document provides an overview of muscle tissue types, including skeletal, cardiac, and smooth muscles. It covers their characteristics, structure, location, and function. The document also details the sliding filament mechanism and components of sarcomeres, with accompanying diagrams and illustrations.
Full Transcript
Cells & Tissues Lect 4: Learning objectives Describe the general features of muscle tissue Muscular tissues consist of elongated cells called muscle fibres (fibres = cells) or myocytes (muscle cells). The muscle tissue uses energy from the hydrolysis of ATP to generate force. The force is a contract...
Cells & Tissues Lect 4: Learning objectives Describe the general features of muscle tissue Muscular tissues consist of elongated cells called muscle fibres (fibres = cells) or myocytes (muscle cells). The muscle tissue uses energy from the hydrolysis of ATP to generate force. The force is a contraction that causes the muscle tissue to produce body movements, maintains posture, provides protection and generates heat. Understanding of the three types of muscle, their location, structure and functional control Three types of muscle - skeletal, cardiac and smooth muscle. Skeletal muscles The tissues of skeletal muscles Location Usually attached to bones by tendons Structure The fibres are long, cylindrical, striated. - Long cells (1mm - 30cm+) - Multinucleate (many peripheral nuclei pushed to the side) Control Voluntary - Usually considered voluntary because it can be made to contract or relax by conscious control. 2 types of skeletal muscle tissue Smallest skeletal muscle - stapedius (1.25mm), controls the tympanic function - controls the tension on the bone, thus transmits external sound vibrations. Largest skeletal muscle - sartorius (60cm), goes from the hip to the top of the tibia (bone above the knee) - this muscle is responsible for use to look at the bottom of our foot. Why are the muscle fibres striated? The striations of the skeletal muscle fibres are due to the highly organised arrangements of myofibrils within the cells. Myofibrils (2 um diam) more or less fill the cytoplasm (sarcoplasm) of the muscle fibre and extend its entire length within the cell. What are the myofibrils composed of? 1. Myofibrils: These are the contractile elements within muscle cells. They consist of two types of filaments: ○ Thin filaments: Primarily composed of actin, these filaments have a diameter of approximately 8 nm and a length of 1-2 μm. ○ Thick filaments: Composed of myosin, these filaments are larger, with a diameter of approximately 16 nm and a similar length of 1-2 μm. 2. Myofilaments: These filaments do not extend the entire length of the muscle fibre. Instead, they are organised into compartments called sarcomeres. Each sarcomere is bounded by Z discs (Z lines). 3. Sarcomeres: The sarcomere is the fundamental functional unit of a myofibril. It consists of overlapping thin and thick filaments. During muscle contraction, these filaments slide past each other, leading to muscle shortening. Why do we see the striations? The thin and thick myofilaments overlap to produce the striations in the myofibril: 1. 2. 3. 4. 5. 6. A Band: ○ What it is: The dark, middle part of the myofibril. ○ Contents: Contains all the thick filaments (made of myosin). ○ Visualise: Imagine it as the chunky centre of a chocolate bar. I Band: ○ What it is: The lighter region between A bands. ○ Contents: Contains only thin filaments (primarily actin). ○ Visualise: Picture it as the vanilla cream filling in an Oreo cookie. H Zone: ○ What it is: The part within the A band where there are no thin filaments. ○ Contents: Consists of thick filaments (myosin) without interference from thin filaments. ○ Visualise: Think of it as the empty space in the middle of the chocolate bar. M Line: ○ What it is: The middle of a sarcomere (the repeating unit of a myofibril). ○ Role: Hold the thick filaments together. ○ Visualise: Imagine it as the sturdy seam running down the centre of a sandwich. Z Disc: ○ What it is: Passes through the centre of the I band, between sarcomeres. ○ Composition: Made up of proteins called actinins. ○ Function: Links filaments of adjacent sarcomeres. ○ Visualise: Picture it as the zipper that connects neighbouring Oreo cookies. Titin: ○ What it is: A giant protein. ○ ○ ○ Role: Links the Z disc to the M line. Importance: Provides resting tension in the I band and acts like a molecular spring. Visualise: Think of it as a stretchy bungee cord within the sandwich. What is the sliding filament mechanism? This mechanism helps understand how muscles contract - the arm is just an example like the leg (don’t get it confused). 1. Sarcomeres: When we examine muscle cells under a microscope, we observe a striped pattern called striations. These striations are formed by sarcomeres, which are basic units stacked throughout muscle tissue. Each muscle cell can contain thousands of sarcomeres. Within an individual sarcomere, there are parallel actin (thin) and myosin (thick) filaments. 2. Sliding Interaction: The core of sarcomere shortening lies in the interaction between myosin and actin proteins. Imagine these filaments as sliding past each other. Here’s how it works: ○ Myosin Heads: Myosin heads attach to the thin filaments (actin) and “walk” along them. ○ Progressive Pulling: As myosin heads move, they progressively pull the thin filaments toward the M line (located at the centre of the sarcomere). ○ Filament Sliding: The thin filaments slide inward, meeting at the centre of the sarcomere. In some cases, they even overlap. ○ I Band and H Zone: As the thin filaments slide inward, the I band (lighter region) and H zone (central region) narrow and eventually disappear when the muscle is maximally contracted. ○ A Band: The A band (dark region) remains relatively constant in length during contraction. It contains thick myosin filaments. ○ Z Discs: Thin filaments on each side of the sarcomere are attached to Z discs. When thin filaments slide inward, the Z discs come closer together, resulting in sarcomere shortening. ○ Muscle Fibre Shortening: Shortening of sarcomeres leads to the shortening of the entire muscle fibre, which, in turn, causes the entire muscle to shorten. Skeletal muscles also contain connective tissues surrounding muscle fibres, and blood vessels and nerves. In order to understand how contraction of skeletal muscle can generate tension, we need to understand microscopic anatomy. 1. Epimysium: ○ What it does: Surrounds the entire muscle (like a tough outer coat). ○ Function: Provides support and protection. ○ Visualise: Imagine it as the muscle’s sturdy overcoat. 2. Perimysium: ○ What it does: Wraps around bundles of muscle fibres (called fascicles). ○ Role: Contains blood vessels, nerves, and collagen fibres. ○ Visualise: Picture it as a protective casing around a bundle of straws (representing fascicles) within the muscle. 3. Endomysium: ○ What it does: Encircles individual muscle fibres (often called muscle cells). ○ Role: Supports muscle fibres and houses capillaries and nerves. ○ Visualise: Think of it as a fine mesh around each straw (representing muscle fibres) within the fascicle. 4. Sarcoplasm: ○ What it is: The cytoplasm of a muscle cell. ○ Contents: Contains organelles like mitochondria and myofibrils. ○ Function: Houses machinery for muscle function. ○ Visualise: Like a busy factory floor inside the muscle cell. 5. Sarcolemma: ○ What it is: The muscle cell’s plasma membrane. ○ Role: Separates the inside from the outside. ○ Visualise: Imagine it as the protective outer skin of the muscle cell. Need to be able to identify the elements from the diagram Cardiac muscle The tissue of the cardiac muscle Location Heart wall Structure Striated Branched Single central nucleus Intercalated discs - where the fibres (cells) join end-to-end. Control Involuntary What are the intercalated discs made up of? Two types of epithelial tissues - desmosomes and gap junctions 1) Gap Junctions: Within intercalated discs, gap junctions facilitate rapid electrical communication between neighbouring cells. These tiny channels allow ions (such as calcium and sodium) to flow, ensuring coordinated contractions. 2) Desmosomes: Intercalated discs also contain desmosomes, which are protein complexes that bind intermediate filaments. Desmosomes provide adhesion between adjacent cells during contraction. Understanding the concept of Purkinje fibres 1. What Are Purkinje Fibres? ○ Think of Purkinje fibres as the heart’s electrical messengers. They’re like tiny couriers that deliver important messages within the heart. ○ These specialised muscle cells are found in the ventricles (the lower chambers of the heart). 2. Job Description: Electrical Conductors ○ Purkinje fibres have a crucial job: they conduct electrical signals. ○ Imagine them as the heart’s wiring system—connecting different parts and ensuring smooth communication. 3. Less Myofibrils, More Connexins ○ Purkinje fibres are a bit different from regular muscle cells: They have fewer myofibrils (those contractile units responsible for muscle movement). Instead, they’re loaded with something called “connexins”. ○ These connexins create tiny gap junctions—like secret passages—between neighbouring cells. ○ Through these gaps, electrical signals zip around, making sure everyone’s on the same page. 4. Why They Matter ○ Purkinje fibres help orchestrate the heart’s rhythm. ○ When your heart beats, it’s thanks to these little messengers doing their job. ○ They ensure that the ventricles contract in sync, pushing blood where it needs to go. Smooth Muscle tissue Location Walls of hollow internal organs Structure Function Intestines: It helps with peristalsis, the coordinated squeezing that pushes food along. Blood Vessels: Here, it’s responsible for constricting or narrowing blood vessels. Iris of the Eye: Yep, even our eyes have some smooth muscle action going on! Reproductive, Digestive, Respiratory, Urinary Systems: Basically, it’s everywhere. Skin: Ever get goosebumps? That’s smooth muscle at work (thanks, erector pili muscles)! Smooth muscle cells are short, small, and spindle-shaped. Picture them as little warriors—only about 30-200 micrometres long (that’s super tiny!). They’re thickest in the middle, like mini sausages Smooth muscle doesn’t follow our conscious commands. It’s involuntary—doing its thing without us even thinking about it. Unlike the stripy look of skeletal muscle, smooth muscle is non-striated (no stripes) 1. What Is a Smooth Muscle? ○ Imagine smooth muscle as the quiet achiever in our body—it’s everywhere, from our intestines to blood vessels. ○ Unlike the showy stripy muscles, smooth muscle is non-striated (no stripes). 2. The Smooth Muscle Cell Story: ○ Each smooth muscle cell is like a tiny worker with a single central nucleus (its control centre). ○ Inside, there are bundles of thin (actin) and thick (myosin) filaments. ○ These thin filaments attach to something called “dense bodies” (like anchors). ○ Intermediate filaments (non-contractile elements) also connect to these dense bodies. 3. How It Works: Twist and Shout (Contract) ○ When smooth muscle contracts, it’s like a gentle twist. ○ Tension travels to the intermediate filaments (they don’t contract themselves). ○ The cell twists around these stable “rods,” doing its job without fuss. Describe the general features of nervous tissue The nervous tissue is the essential component of the nervous system. There are two type of nervous system - central nervous system (CNS) and Peripheral nervous system (PNS) 1. Central Nervous System (CNS): ○ Imagine the CNS as the command centre of our nervous system. ○ It includes two main parts: Brain: The epicentre of thinking, memory, and coordination. Spinal Cord: The information highway connecting the brain to the rest of the body. ○ Bonus: The optic nerve (for vision) is also part of the CNS. 2. Peripheral Nervous System (PNS): ○ Think of the PNS as the messengers—they carry info between the CNS and the rest of the body. ○ The PNS has two key jobs: Sensory/Afferent Division: These nerves bring info to the CNS. For example, when you touch something hot, sensory nerves tell your brain, “Ouch!” Motor/Efferent Division: These nerves take instructions from the CNS to organs (like muscles and glands). When you want to move your hand, motor nerves make it happen. What is the importance of the nervous system? Maintain homeostasis (along with the endocrine system), initiates voluntary movements and is responsible for perception, behaviour and memory. What are the major functions of the nervous system? (grouped into three major functions) 1. Sensory Function: ○ Think of this as the detective work of our nervous system. ○ What it does: Detects both internal (like hunger or pain) and external (like touching something hot) stimuli. Sends this information to the Central Nervous System (CNS)—our brain and spinal cord. ○ So, when you feel a tickle or hear a loud noise, your sensory nerves are on the case! 2. Integrative Function: ○ Imagine this as the brain’s think tank. ○ What it does: Analyses and processes all the info coming in. Stores memories, thoughts, and experiences. Basically, it’s where the magic happens—decisions, problem-solving, and understanding. ○ So, when you remember your friend’s birthday or figure out a puzzle, that’s your integrative function at work! 3. Motor Function: ○ This is like the action hero of the nervous system. ○ What it does: Takes instructions from the CNS (like a command from the brain). Sends these instructions to effectors—that’s just a fancy word for muscles and glands. So, when you move your hand, blink, or even sweat, your motor nerves are the ones making it happen! What is the nervous tissue made up of? Neurons (nerve cells) and neuroglia (collective cells that support the neurons), Neurons are the longest cells in the body. Neurons (the building blocks of our nervous system) 1. Neuron Basics: ○ Neurons are like the messengers of our nervous system. ○ They have a few key parts: Cell Body (Soma): Think of this as the neuron’s headquarters. It contains the nucleus and other essential components. Dendrites: These are like tiny branches reaching out from the cell body. They receive incoming nerve impulses (also called action potentials). Axon: Picture the axon as a long, slender cable. It carries the nerve impulse away from the neuron. It’s the neuron’s output part. 2. Special Traits of Neurons: ○ Non-Dividing: Neurons don’t play the cell division game. Once they’re formed, they stick around. ○ High Metabolic Rate: Neurons are like the energy guzzlers of our body—they need lots of oxygen and nutrients to function. ○ Sensitive to Oxygen: Without oxygen, neurons don’t last long. They’re like delicate flowers in need of fresh air. 3. Dendrites and Axons in Action: ○ Dendrites: Imagine them as the neuron’s receptionists. They listen for incoming messages from other neurons. ○ Axon: This is the neuron’s delivery guy. It takes the nerve impulse and delivers it to another neuron or even to muscle or gland tissue. Have an understanding of the function of the different components The neurons come in multiple shapes - there are 4 major once that we focus on Multipolar Neurons - ‘Social butterflies’ of the nervous system Key Features: ○ Multiple Dendrites: They have two or more dendrites branching out from the cell body. ○ Single Axon: There’s one long axon that carries messages away. Where to Find Them: ○ They’re the most common type of neurons in the Central Nervous System (CNS). ○ Bonus: All motor neurons (the ones controlling our skeletal muscles) belong to this class. Super Long Travellers: ○ Some multipolar neurons are like the long-haul travellers: They stretch from the spinal cord all the way down to our toe muscles! Imagine the journey they make—delivering commands for movement and coordination. Bipolar Neurons - special agents Key Features: Two Distinct Processes: - - - Dendritic Process: It can branch at the tip but not at the cell body. Think of it as the neuron’s receiving antenna. Axon: This is the neuron’s transmitter cable. It carries messages away. Cell Body (Soma): It sits between the axon and dendrite, coordinating the neuron’s activities. - Small and Rare: Bipolar neurons are tiny (about 30 micrometres) and not commonly found. - Special Sense Organs: They play a crucial role in relaying information from sensory receptors (like those for sight, smell, and hearing) to other neurons. 1. Where to Find Them: ○ Bipolar neurons are like the silent operators behind our senses: In the retina of our eyes, they help with vision. In the olfactory epithelium (that’s your nose), they’re involved in smell. And in the cochlea of the inner ear, they handle hearing. Unipolar neurons - long-distance runners Key Features: - Continuous Dendrites and Axon: Imagine them as a single, uninterrupted cable. - Cell Body (Soma): It’s off to one side, like a quiet observer. - Axon: The whole thing—from where the dendrites converge—is called the axon. - Mostly Sensory Nerves: Unipolar neurons are often sensory specialists. - Super Long: Some unipolar neurons stretch up to 1 meter! That’s like going from your CNS (central nervous system) all the way down to your toe tip. 1. Where to Find Them: ○ Unipolar neurons are like the information superhighways: They’re involved in relaying sensory info—like touch, temperature, and pain—from our skin, muscles, and organs to the CNS. So when you feel a gentle breeze or a pinch, thank your unipolar neurons! Anaxonic neuron 1. Rare and Mysterious: ○ Anaxonic neurons are like the shy introverts at the neuron party. ○ We don’t see them often, and their exact function remains a bit of a mystery. 2. Dendrites and Axons? Who Knows!: ○ Their anatomy is like a puzzle: you can’t easily tell their dendrites apart from their axons. ○ Imagine them as the shape-shifters of the neuron world. 3. Where to Find Them: ○ Anaxonic neurons hang out in the brain (the ultimate think tank) and in special sense organs. ○ They’re like the backstage crew, quietly doing their thing. Neuroglia 1. Where They Hang Out: ○ Neuroglia are like the support crew for neurons. You’ll find them both in the Central Nervous System (CNS) and the Peripheral Nervous System (PNS). 2. Volume and Numbers: ○ They make up about 50% of the CNS volume—like the glue holding everything together. ○ While neurons get the spotlight, neuroglia are smaller but more numerous (like a bustling crowd). 3. What They Don’t Do: ○ Unlike neurons, neuroglia don’t play the action potential game (those electric impulses). ○ But they can still communicate with each other. 4. Superpowers of Neuroglia: ○ Physical Structure: They provide the framework for nervous tissue. ○ Repair Crew: When neurons need fixing, neuroglia step in. ○ Phagocytosis: They’re like the clean-up crew, gobbling up debris. ○ Nutrient Delivery: Neurons get their snacks from neuroglia. ○ Fluid Regulation: They keep the interstitial fluid (the brain’s juice) in check. ○ Types of neuroglia CNS neuroglia Astrocytes, the multitasking stars of our nervous system! Let’s explore their role in simpler terms: Astrocytes are like the support crew for neurons. Key Features: Star-Shaped: They’re the largest and most numerous neuroglia, resembling little stars. Syncytium Network: Imagine them as a connected web, communicating with each other. Support and Repair: Astrocytes provide structural support and help repair brain tissue (scar formation). Gliotransmitters: They chat with neurons using special molecules like glutamate. Environment Managers: Astrocytes maintain the brain’s environment by regulating ions (like sodium and potassium). Blood-Brain Barrier Heroes: They wrap around blood vessels, influencing their permeability and maintaining the blood-brain barrier. Oligodendrocytes These cells are like the insulators for our neurons. Key Features: Myelin Sheath: Oligodendrocytes wrap around CNS axons, creating a multilayered myelin sheath (a mix of proteins and lipids). Speed Boosters: Myelin sheaths help accelerate action potentials (those electric signals) along axons. One Oligodendrocyte, Many Axons: Unlike in the PNS (where Schwann cells myelinate one axon), an oligodendrocyte can myelinate multiple axons. Microglia These cells are like the bodyguards of our nervous system. Key Features: Phagocytic Heroes: Microglia are the resident macrophages—they gobble up debris, damaged cells, and foreign invaders. Protection Squad: They’re always on the lookout, ensuring the brain stays safe and healthy. Ependymal Cells Ependymal cells are like the fluid engineers of our nervous system. Key Features: CSF Production: They’re the ones responsible for producing cerebrospinal fluid (CSF). Lining Ventricles and Central Canal: Imagine them as the interior decorators—they line the CSF-filled ventricles in the brain and the central canal of the spinal cord. Cuboidal Shape: Ependymal cells form a single layer and are mostly cuboidal in shape. Cilia and Microvilli: They have tiny hair-like structures called cilia (for flow) and microvilli (for sampling). Locations: You’ll find them in the ventricles and other spots where CSF hangs out. 1. CSF’s Superpowers: Mechanical Buffer: CSF acts like a cushion, protecting our brain and spinal cord from bumps and jolts. Nutrient Transport: It’s like a nutrient-delivery service, moving goodies to neurons. Waste Removal: CSF also helps clear away waste products. Peripheral Nervous System Neuroglia 1. Schwann Cells: ○ These cells are like the personal bodyguards for PNS axons. ○ Key Features: Myelin Sheath: Schwann cells wrap around PNS axons, creating an insulating myelin sheath. One Axon, One Schwann Cell: Each axon gets its own Schwann cell for myelination. Support Crew: Schwann cells also support and surround several non-myelinated axons. 2. Satellite Cells: ○ These cells are like the neighbors of our neuron cell bodies. ○ Key Features: Cell Body Guardians: Satellite cells surround neuron cell bodies, providing support. Fluid Exchange: They’re like the caretakers, ensuring nutrients and waste products move smoothly around neurons. Equivalent to Astrocytes: In the PNS, satellite cells play a role similar to astrocytes in the CNS.