MSOP1015 Pharmaceutical Analysis Lecture 1: Intermolecular Interactions 2024-2025 PDF
Document Details
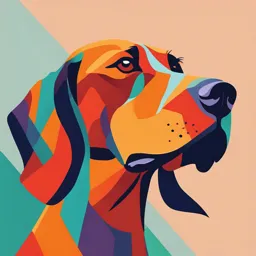
Uploaded by ProductiveSerpentine6303
Medway School of Pharmacy
2024
Andrew J Hall
Tags
Summary
These are lecture notes related to intermolecular interactions in pharmaceutical analysis. The lecture is for MSOP1015 and is suitable for undergraduate students.
Full Transcript
MSOP1015 Pharmaceutical Analysis Lecture 1: Intermolecular interactions Dr. Andrew J Hall Senior Lecturer in Chemistry Room A120, Anson Building [email protected] Recommended texts An introduction to Medicinal...
MSOP1015 Pharmaceutical Analysis Lecture 1: Intermolecular interactions Dr. Andrew J Hall Senior Lecturer in Chemistry Room A120, Anson Building [email protected] Recommended texts An introduction to Medicinal Chemistry Graham L. Patrick (2017) (2013) (2009) The Organic Chemistry of Drug Design and Drug Action Richard B. Silverman (& Mark W. Holladay) Learning outcomes At the end of the lecture, you should: Understand why and how molecules interact with each other recognise and understand the different types of non-covalent interactions that may occur between molecules understand how the nature and strength of intermolecular interactions may be measured appreciate how this knowledge can help us in many areas relating to drugs and medicines DNA secondary structure Protein secondary structure – the -helix Each step contains 3.6 amino acid residues which form hydrogen bonds between each other Protein secondary structure – the -sheet Formed by hydrogen bonds between N-H and C=O groups of neighbouring chains anti-parallel parallel Protein tertiary structure – the -sheet Formed by attraction between -helices and -sheets by a range on covalent and non-covalent interactions How enzymes work What about the detail? YOU need an appreciation of intermolecular interactions Function/action of biological macromolecules Mechanisms of drug action Drug metabolism Drug/excipient interactions Excipient/environment interactions Chromatographic separation mechanisms Supramolecular chemistry Study of systems involving aggregates of molecules/ions held together by non-covalent interactions:- electrostatic effects ion-dipole/dipole-dipole interactions hydrogen bonding charge-transfer interactions the hydrophobic effect − interactions cation- interactions halogen bonding London dispersion forces and van de Waals interactions A brief backwards step Covalent bonding Bonds where one or more electron pairs is shared by two atoms They are short, strong and highly dependent on orientation Ionic (electrostatic) interactions Formed between ions bearing opposite charges Consider protein receptors at physiological pH Arginine, lysine, histidine: positively charged side chains Aspartic acid, glutamic acid: negatively charged side chains Pivagabine (anti-depressant) Ionic (electrostatic) interactions Strength and properties Comparable in strength to covalent bonds: 200 – 300 kJ/mol Non-directional Operate over reasonable long distances (compared to other non-covalent interactions) Bond strength is proportional to 1/r2 (r is the distance between the two interacting charges) Ion-dipole/dipole-dipole interactions Consider distribution of electrons in C-X bonds Where X = O, N, S, halogen Non-symmetrical electron distribution ➔ electronic dipoles These can be attracted to ions or other dipoles Zalepon dipole-dipole (anti-insomnia) ion-dipole Ion-dipole/dipole-dipole interactions Strength and properties Weaker than ionic interactions: ion-dipole: 250 - 200 kJ/mol dipole-dipole: 5– 50 kJ/mol Directional There is a requirement for complementarity Hydrogen bonding This is the MOST IMPORTANT non-covalent interaction a special type of dipole-dipole interaction Examples: DNA, proteins, the unusual properties of water Formed between proton of a X-H group (where X is electronegative) and one or more other electronegative atoms (Y) possessing lone pairs (can be inter- or intramolecular) Hydrogen bonding Strength and properties Weaker than ionic and ion-dipole interactions: Typical strengths: 4 – 120 kJ/mol Directional Additive Complementarity required Operate over a short range: 2.5 – 3.5 Å (ångström) (0.25 – 0.35 nm) Hydrogen bonding Directionality and geometry Strength depends on nature of D–H----A distance and on bond angle RO-H---O=C distance 2.75 Å R2N-H---O=C distance 2.90 Å Hydrogen bonding Additive effects While single hydrogen bonds are rather weak, multiple cooperative hydrogen bonds lead to an increase in interactions strength Kd = 2 x 10-3 M Kd = 1 x 10-4 M Kd = 5 x 10-6 M Hall et al., Anal. Chem. 2006, 78, 8362-8367 Zimmerman et al., J. Am. Chem. Soc. 1992, 114, 4011-4013 Meijer et al., Angew. Chem. Int. Ed. 1998, 37, 75-78 Hydrogen bonding Primary and secondary H bonds Primary: direct interaction between donor (D) and acceptor (A) groups Secondary: interactions with neighbouring groups These can be either attractive or repulsive Kd = 10-5 – 10-6 M Kd = 10-2 – 10-3 M Note: each system has 3 primary hydrogen bonds Hydrogen bonding Intramolecular Stability depends on Ring size: 6-membered >> 5-membered > 7-membered Acceptor strength: carbonyl > heterocyclic N > sulfoxide Salicylic acid intramolecular intermolecular Hydrogen bonding Effect of intramolecular hydrogen bonding Masking of pharmacophoric groups Methyl salicylate Methyl 4-hydroxybenzoate (Methyl 2-hydroxybenzoate) (Methyl paraben) Production of biosteric structures Increase a compound’s lipophilicity Some intramolecular H bonds can persist in water Charge-transfer complexes Interaction between a good electron donor and a good electron acceptor Effectively a molecular dipole-dipole interaction Strength is proportional to difference in ionisation potentials between the donor and acceptor species Good acceptor: group with -electrons or lone pair electrons Good donor: group with electron deficient -orbitals Interaction strength: 5 – 35 kJ/mol Charge-transfer complexes Example Chloroquine (anti-malarial) Chlorothalonil (fungicide) The hydrophobic effect Origin lies in the fact that water interacts much more strongly with itself than it does with non-polar groups This leads to the exclusion of non-polar groups from aqueous solution Non-polar groups/molecules tend to aggregate https://youtu.be/nfZL0tmcIPM Hydrophobic interactions Creation and maintenance of macromolecular structures and assemblies of cells Protein folding Formation of amphiphilic structures Hydrophobic interactions Liposomes − interactions Different orientations Can be viewed as a type of hydrophobic interaction Layered structure of graphite Base pair stacking in DNA (forms shape and controls drug intercalation) Face-to-face Not favoured − interactions Lacosamide (anticonvulsant) Note: if one ring is electron rich and the other electron poor, charge transfer processes become important Cation- interactions Very common in protein structure Useful for designing drug-receptor interactions Cationic groups on drugs can interact with -systems of the receptor Strength: 5 – 25 kJ/mol Lisdexamfetamine (ADHD) Halogen bonding Covalently bonded halogen can act as electron acceptors ➔halogen bonding to electron-rich donor atoms (O, N, S) Distance between halogen and donor atom is less than the sum of their van der Waals radii Strength: 5 – 25 kJ/mol (exceptionally A phosphodiesterase to 60 kJ/mol) type 5 inhibitor Order: H ≈ I > Br > Cl >>F London dispersion forces Transient dipoles Arise from fluctuations in electron distribution between spatially close species ➔ creation of a dipole moment Atomic nucleus Electron ≡ + - cloud As another species approaches, this instantaneous dipole induces a dipole on the approaching species + - + - + - London dispersion ➔ van der Waals forces These events eventually become a bulk phenomenon van der Waals interactions Fall into three main categories Strength: weak individually < 3 kJ/mol Depend on surface area of contact between molecules and polarizability of the electron shells in those molecules Non-directional Cannot be used to aid drug design, but can be vital to drug-receptor binding Regardless of other interactions, they will always be present Cooperativity Unlikely that only one type of non-covalent interaction is occurring in a system at any one time Formation of first interaction ➔ loss of translational energy in system This means that in subsequent binding events, entropy losses will be lower Consequence: several weak interactions may combine to produce an overall strong interaction Cooperativity A possible example Unlikely that only one type of non-covalent interaction is occurring in a system at any one time, e.g. dibucaine hydrophobic H-bond − ionic, ion-dipole or cation- charge-transfer or H-bond dipole-dipole, hydrophobic H- or halogen-bond hydrophobic Determining interaction strengths There are many ways to measure the strength of complexation between species:- Spectroscopic methods Displacement methods Isothermal titration calorimetry (ITC) Atomic force microscopy (AFM) https://www.nanoscience.com/techniques/atomic-force-microscopy/ Spectroscopic methods UV/Vis and fluorescence spectroscopy Relies on the change in absorbance/fluorescence properties of the system on complexation Kd ≈ 1.4 x 10-3 M (in DMSO) Hall et al., J. Org. Chem. Kd ≈ 2.2 x 10-4 M (in DMSO) 2005, 70, 1732-1736 Spectroscopic methods NMR spectroscopy Relies on the change in chemical shift values on complexation (a) 1H NMR titration of CO with caffeine (amine). From bottom spectrum to top spectrum, they represent different molar ratios of CO against caffeine, from pure CO (n = 0) to much caffeine excess (n = 32). n is the equivalent of caffeine to CO. (b) 1H NMR titration of CO with caffeine (7.70–6.30 ppm range). Inset refers to the proton labelling scheme. The detailed NMR condition has been depicted in Methods. Kd ≈ 16.81 mM (16.81 x 10-3 M) (in CDCl3) Xu et al., Scientific Reports 2013, Volume: 3, Article number:2255 Isothermal titration calorimetry Experiment measures change in enthalpy (H) on complexation RNase A titrated with 2’-CMP Left: signal (heat) produced following each addition of inhibitor; right: integration of heats versus mole role inhibitor/protein Ka = 1 x 106 M-1; DH = -65 kJ/mol Observed binding constant (Ka) can be calculated ➔ determination of G (= -RT lnKa) We now know G and H, so can calculate S (= [H – G] / T) Single experiment ➔ all thermodynamic parameters Learning outcomes Take-home points You should now: Understand why and how molecules interact with each other recognise and understand the different types of non-covalent interactions that may occur between molecules understand how the nature and strength of intermolecular interactions may be measured appreciate how this knowledge can help us in many areas relating to drugs and medicines