Lippincott's Biochemistry Chapter 18 - Cholesterol, Lipoprotein, and Steroid Metabolism PDF
Document Details
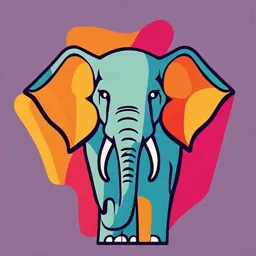
Uploaded by PrizeMeerkat
Tags
Summary
This document is a chapter from a biochemistry textbook, covering cholesterol, lipoprotein, and steroid metabolism.
Full Transcript
Cholesterol, Lipoprotein, and Steroid Metabolism Ma)or sources of llver cholesterol I. OVERVIEW Cholesterol...
Cholesterol, Lipoprotein, and Steroid Metabolism Ma)or sources of llver cholesterol I. OVERVIEW Cholesterol Cholesterol, the characteristic steroid alcohol of animal tissues, performs Dietary from a number of essential functions in the body. For example, cholesterol is a chol811terol utrahepatlc structural component of all cell membranes, modulating their fluidity, and, tlUU811 in specialized tissues, cholesterol is a precursor of bile acids, steroid hor- t Chylomlcron t mones, and vitamin D. Therefore, it is critically important that Iha cells of the remrwnte HDL body be assured an appropriate supply of cholesterol. To meet this need, a complex series of transport, biosynthetic, and regulatory mechanisms Denovo has evolved. The liver plays a central role in the regulation of the body's eyntheel cholesterol homeostasis. For example, cholesterol enters the hepatic cho- lnthellver lesterol pool from a number of sources including dietary cholesterol as well as that synthesized de novo by extrahepatic tissues and by the liver itself. Cholesterol is eliminated from the liver as unmodHied cholesterol in lhe bile, or it can be converted to bile salts that are secreted into the intestinal lumen. It can also serve as a component of plasma lipoproteins that carry lipids to the peripheral tissues. In humans, the balance between Liver cholesterol influx and efflux is not precise, resuHing in a gradual deposition Cholesterol of cholesterol in the tissues, particular1y in the endothelial linings of blood Pool vessels. This is a potentially life-threatening occurrence when the lipid deposition leads to plaque formation, causing the narrowing of blood ves- sels (atherosclerosis) and increased risk of cardio-, cerebro-, and periph- eral vascular disease. Figure 18.1 summarizes lhe major sources of liver cholesterol and the routes by which cholesterol leaves the liver. Secretion of Connnslon II. CHOLESTEROL STRUCTURE VLDL toblle acldltlaalta Cholesterol is a very hydrophobic compound. It consists of four fused hydrocarbon rings (A-D) called the steroid nucleus, and it has an eight- Ma)or routes by which carbon, branched hydrocarbon chain attached to carbon 17 of the D ring. cholesterol leaves the llver Ring A has a hydroxyl group at carbon 3, and ring B has a double bond between carbon 5 and carbon 6 (Fig. 18.2). Figure 18.1 Sources of liver cholesterol (influx) A. Sterols and routes by which cholesterol leaves the liver (efflux). HDL and Steroids with 8 to 1ocarbon atoms in the side chain at carbon 17 and VLDL =high- and very-low-density a hydroxyl group at carbon 3 are classified as sterols. Cholesterol is lipoproteins. 219 220 18. Cholesterol, Lipoprotein, and Steroid Metabolism Hydrocarbon chain the major sterol in animal tissues. It arises from de novo synthesis and absorption of dietary cholesterol. Intestinal uptake of cholesterol 22 24 is mediated by the Niemann-Pick C1 -like 1 protein, the target of the drug ezetimibe that reduces absorption of dietary cholesterol (see p. 176). [Note: Plant sterols (phytosterols), such as ~sitosterol, are poorty absorbed by humans (5% absorbed as compared to 40% for cholesterol). After entering the enterocytes, they are actively trans- ported back into the intestinal lumen. Defects in the efflux transporter HO (ABCG5/8) result in the rare condition of sitosterolemia. Because some cholesterol is transported back as well, plant sterols reduce the absorption of dietary cholesterol. Daily ingestion of plant sterol esters supplied, for example, in spreads, is one of a number of dietary strate- gies to reduce plasma cholesterol levels (seep. 363).] Flltty acid B. Cholesteryl esters i9 -c 0 Most plasma cholesterol is in an esterified form (with a fatty acid [FA] attached at carbon 3, as shown in Fig. 18.2), which makes the struc- Cholaetaryl aeter ture even more hydrophobic than free (nonesterified) cholesterol. Cholesteryl esters are not found in membranes and are normally present only in low levels in most cells. Because of their hydropho- bicity, cholesterol and its esters must be transported in association Figure 18.2 with protein as a component of a lipoprotein particle {see p. 227) or Structure of cholesterol and its ester. be solubilized by phospholipids and bile salts in the bile (seep. 226). Ill. CHOLESTEROL SYNTHESIS 0II 2CH,- C- CoA Cholesterol is synthesized by virtually all tissues in humans, although 2AcelylCoA liver, intestine, adrenal cortex, and reproductive tissues, including ova- ries, testes, and placenta, make the largest contributions to the choles- -~- terol pool. As with FA, all the carbon atoms in cholesterol are provided by acetyl coenzyme A (CoA), and nicotinamide adenine dinucleotide phos- phate (NADPH) provides the reducing equivalents. The pathway is end- 0II 0It ergonic, being driven by hydrolysis of the high-energy thioester bond of CHa- C - C"2- C- CoA acetyl CoA and the terminal phosphate bond of ATP.. Synthesis requires Acetoacetyl CoA (4 C) enzymes in the cytosol, the membrane of the smooth endoplasmic retic- ulum (SER), and the peroxisome. The pathway is responsive to changes "'°±c:J-- HMG CaA synthasei~ CoA in cholesterol concentration, and regulatory mechanisms exist to balance the rate of cholesterol synthesis against the rate of cholesterol excretion. An imbalance in this regulation can lead to an elevation in circulating lev- els of plasma cholesterol, with the potential for vascular disease. A. 3-Hydro.xy-3-methylglutaryl coenzyme A synthesis The first two reactions in the cholesterol biosynthetic pathway are similar to those in the pathway that produces ketone bodies (see S.Hydroxy-3-methylglutaryl CoA (6 C) (HllGCoA) Fig. 16.22, p. 196). They result in the production of 3-hydroxy..S.-meth- ylglutaryl CoA ([HMG CoA], Fig. 18.3). First, two acetyl CoA mol&- cules condense to form acetoacetyl CoA. Next, a third molecule of Figure 18.3 acetyl CoA is added by HMG CoA synthase, producing HMG CoA, Synthesis of HMG CoA. CoA = a six-carbon compound. [Note: Liver parenchymal cells contain two coenzymeA. isoenzymes of the synthase. The cytosolic enzyme participates in cholesterol synthesis, whereas the mitochondrial enzyme functions in the pathway for ketone body synthesis.] Ill. Cholesterol Synthesis 221 B. Mevalonata synthesis Expression le HMG CoA is reduced to mevalonate by HMG CoA reductase. This lnhlbHedby cholesterol. is 1he rate-limiting and key regulated step in cholesterol synthesis. It occurs in the cytosol, uses two molecules of NADPH as the reducing ~ q~a,.OH ! agent, and releases CoA, making the reaction irreversible (Fig. 18.4). ,C, ,...c, / C, [Note: HMG CoA reductase is an integral membrane protein of the -o CH2 CH2 CoA SER, with its catalytic domain projecting into the cytosol. Regulation r HMGCoA (IC) of reductase actMty is discussed in D. below.] HMG CaA ffKluctaBe 2 NADPH + 2 H+ c. Cholaaterol synthesis from mavalonata CoA~2NADP+ The reactions and enzymes involved in 1he synthesis of cholesterol from mevalonate are illustrated in Figure 18.5. [Note: The numbers shown in ~ C.,tl3,.0H brackets below correspond to numbered reactions shown in this figure.] ,C, _... C, ,.....CH.OH -o CH2 Ct\ (1] Mevalonate is converted to 5-pyrophosphomevalonate in two steps, each of which transfers a phosphate group from ATP. llevalo..a. (& C) A five-carbon isoprene unit, isopentenyl pyrophosphate (IPP), is formed by the decarboxylation of 5-pyrophosphomevalonate. The Flgure18A reaction requires ATP. [Note: IPP is 1he precursor of a family of Synthesis of mevalonate. HMG CoA = molecules with diverse functions, the isoprenoids. Cholesterol is hydroxymethyfglutaryl coenzyme A; a sterol isoprenoid. Nonsterol isoprenoids include dolichol (see NADP(H) =nicotinamide adenine dinucleotide phosphate. p. 167) and ubiquinone (or, coenzyme Q; seep. 75).] (3] IPP is isomerized to 3,3-dimethylallyl pyrophosphate (OPP). IPP and OPP condense to form 1a-carbon geranyl pyrophos- phate (GPP). A second molecule of IPP 1hen condenses with GPP to form 15-carbon farnesyl pyrophosphate {FPP}. [Note: Covalent attach- ment of famesyl to proteins, a process known as prenylation, is one mechanism for anchoring proteins (for example, ras) to the inner face of plasma membranes.] Two molecules of FPP combine, releasing pyrophosphate, and are reduced, forming the 30-carbon compound squalene. [Note: Squalene is formed from six isoprenoid units. Because 3 ATP are hydrolyzed per mevalonate residue converted to IPP, a total of 18 ATP are required to make the polyisoprenoid squalene.] Squalene is converted to the sterol lanosterol by a sequence of two reactions catalyzed by SER-associated enzymes that use molecular oxygen (02) and NADPH. The hydroxylation of linear squalene triggers the cyclization of the structure to lanosterol. The conversion of lanosterol to cholesterol is a multistep pro- cess involving shortening of the side chain, oxidative removal of methyl groups, reduction of double bonds, and migration of a double bond. Smith-Lemli-Opitz syndrome (SLOS), an auto- somal-recessive disorder of cholesterol biosynthesis, is caused by a partial deficiency in 7-dshydrocholBStsroJ-7-reductase, the enzyme that reduces the double bond in 7-dehydrocholesterol (7-DHC), thereby converting it to cholesterol. SLOS is one of sev- eral multisystem, embryonic malformation syndromes associated with impaired cholesterol synthesis. [Note: 7-DHC is converted to vitamin 03 in the skin (see p. 390).] 222 18. Cholesterol, Lipoprotein, and Steroid Metabolism Mevalonate 5-Pyrophoaphornevalonate laopentenyl pyrophoaphale (IPP) (&C) {&C) (5C) The plloaphorylallon ot mavalonalB and prmance al pyroph08ph819 In aubeaquant mln.lc:b.11118 help lmap lhMB watar-lnmluble compoundll In.alutlon. lsomenJ88 CH3 CH2 C=C. CHa / CH2')- ® - ® @@ CM- ~~ CH2')- @ - @ @- @ IPP ' _ / ' "' ,C- C, CH2 'H p - p IPP ~ ~ / ,C=C, (~ ~ CH3 H (-~--~- CH3 H Synlhllse Sym/laM FamNYI pyrophosphate (FPP) Geranyl pyrophosphlrte Dlm..hylallyl (15C) (10C) pyrophoephate FPP (5C) Pyrophoephate le released In each ot these tour ® -® condenaatlon atepe, making the reactions lrreverslble. NADPH+H+ Beginning with squalene, the Intermediates In cholesterol blosynthesls are nonphosphorylated NADp+ and are so hydrophobic that they require an Intracellular sterol carrier protein lo keep them soluble. ® -® Squlllene ~ 0. ~ ~ #~~ NADPH NADp+ HO +H+ Squalene Lanoelerol Cholesterol (30C) (30 C; ftrel sterol) (27C) Figure 18.5 Synthesis of cholesterol from mevalonate. ADP =adenoslne dlphosphate; ® =phosphate; ® ,., ® =pyrophosphate; NADP(H) = nicotinamide adenine dinucleotide phosphate. D. Cholesterol syn1hesis regulation HMG CoA reductass is the major control point for cholesterol biosyn- thesis and is subject to different kinds of metabolic control. 1. Sterol-dependent regulatlon of gene expression: Expression of the gene fer HMG CoA reductass is controlled by the trans-acting factor, sterol regulatory element-binding protein-2 (SREBP-2), which binds DNA.at the cis-acting sterol regulatory element (SAE) upstream of the reductase gene. Inactive SREBP-2 is an integral protein of the SER membrane and associates with a second SER membrane pro- tein, SREBP cleavage-activating protein {SCAP). When sterol levels Ill. Cholesterol Synthesis 223 in 1he SER are low, the SREBP-2-SCAP complex moves from the ER to the Golgi. In 1he Golgi membrane, SREBP-2 is sequentially acted upon by two proteases, which generate a soluble fragment that enters 1he nucleus, binds the SRE, and functions as a 1ranscription factor. This results in increased synthesis of HMG CoA reductase and, therefore, increased cholesterol synthesis (Fig. 18.8). However, if sterols are abundant, 1hey bind SCAP at its sterol-sensing domain and induce the binding of SCAP 10 yet other ER membrane proteins, the insulin-induced gene proteins (INSIG). This results in 1he reten- tion of the SCAP-SREBP complex in 1he SER, thereby preventing the activation of SREBP-2 and leading 10 downregulation of choles- terol synthesis. [Note: SREBP-1c upregulates expression of enzymes involved in FA synthesis in response to insulin (see p. 184).] 2. Sterol-accelerated enzyme degradation: The reductase itself is a sterol-sensing integral protein of 1he SER membrane. When ste· rol levels in the SER are high, the enzyme binds to INSIG proteins. Binding leads 10 cytosolic transfer, ubiquitination, and proteasomal degradation of 1he reductase {see p. 247). 3. Sterol-lndependent phosphorylatlonfdephosphorylatlon: HMG CoA reducta.se actMty is controlled covalently through the actions of adenosine monophosphate {AMP)-acfivated protein kinase (lAMPKJ see p. 183) and a phosphoprotein phosphatase (see Fig. 18.6). The phosphorylated form of 1he enzyme is inactive, whereas 1he dephosphorylated form is active. [Note: Because AMPKis activated by AMP, cholesterol synthesis, like FA synthesis, is decreased when ATP availability is decreased.] 4. Hormonal regulatlon: The activity of HMG CoA reductase is con- trolled hormonally. An increase in insulin favors dephosphorylation (activation) of the reductass, whereas an increase in glucagon and epinephrine has the opposite effect. r -SREBP.2~ ---......._ Pro1eolytlc. ~ DNA cleava - ~ CYTOSOL SRE i Tranacrlpllon '-~.--' Golgl ~ lleuenger RNA (mRNA) ,,, 1111.. )i.- 0 9 ~ mANA SREBP-2 SCAP INSIG ~ titO P1;:C1!: P I Traneletlon °' HllG CoA ~ ~ ~ ) HllGt CoA E'!:°.:lumlc l'fHlupta.. (inactive) +oxyl group. The carboxyl group has a pKa (see p. 6) of -6. In the duodenum {pH -6), this group will be protonated in haH of the molecules (the bile acids) and deprot- onated in the rest (the bile salts). The terms bile acid and bile salt are HO...... frequently used interchangeably, however. Both forms have hydroxyl H groups that are ll in orientation (they lie below the plane of the rings) Chollcacld and methyl groups that are~ {they lie above the plane of the rings). Therefore, the molecules have both a polar and a nonpolar surface COOH and can act as emulsifying agents in the intestine, helping prepare dietary fat (triacylglycerol [TAG]) and other complex lipids for degra- dation by pancreatic digestive enzymes. B. Synthesis HO'"' Bile acids are synthesized in the liver by a multistep, multiorganelle H pathway in which hydroxyl groups are inserted at specific positions Chanodeoxychollc acid on the steroid structure; the double bond of the cholesterol B ring is reduced; and the hydrocarbon chain is shortened by three carbons, introducing a carboxyl group at the end of the chain. The most com- Figure 18.8 mon resulting compounds, cholic acid (a triol) and chenodeoxycholic Bile acids. [Note: The ionized forms acid {a diol), as shown in Figure 18.8, are called primary bile acids. are bile salts.] [Note: The rate-limiting step in bile acid synthesis is the introduction of V. Bile Acids and Bile Salts 225 a hydroxyl group at carbon 7 of the steroid nudeus by 7-a-hydroxy/ase, a SER-associated cytochrome P450 monooxygenase found only in liver. Expression of the enzyme is downregulated by bile acids (Fig. 18.9)]. C. Conjugation Before the bile acids leave the liver, they are conjugated to a molecule of either glycine or taurine (an end product of cysteine metabolism) by an amide bond between the carboxyl group of the bile acid and the amino group of the added compound. These new structures include glycocholic and glycochenodeoxycholic acids and taurocholic and taurochenodeoxycholic acids (Fig. 18.10). The ratio of glycine to tau- rine forms in the bile is -3.11. Addition of glycine or taurine results in the presence of a carboxyl group with a lower pKa (from glycine) or a sulfonate group (from taurine), both of which are fully ionized (nega- tively charged) at the alkaline pH of bile. The conjugated, ionized bile salts are more effective detergents than the unconjugated ones because of their enhanced amphipathic nature. Therefore, only the HO conjugated forms are found in the bile. lndMduals with genetic defi- ciencies in the conversion of cholesterol to bile acids are treated with 7-o:-Hydraxycholeeterol exogenously supplied chenodeoxycholic acid. ~~ \ Challc acid Chenodeoxychollc acid Bile salts provide the only significant mechanism for choles· ( biol) (a dlol) terol excretion, both as a metabolic product of cholesterol and 11 as a aolubilizer of cholesterol in bile. Figure 18.9 Synthesis of the bile acids cholic acid and chenodeoxycholic acid from D. Bacterial action on bile saHs cholesterol. ------------- Bacteria of the intestinal microbiota (see p. 372) can deconjugate (remove glycine and taurine) bile salts. They can also dehydroxylate Challc acid Cllyclne carbon 7, producing secondary bile salts such as deoxycholic acid ~ H from cholic acid and lithocholic acid from chenodeoxycholic acid. C- N -~ E. Enterohapatlc clrculatlon Bile salts secreted into the intestine are efficiently reabsorbed (>95%) and reused. The liver actively secretes bile salts via the bile salt export pump. In the intestine, they are reabsorbed in the terminal ileum via H the apical sodium (Na+}-bile salt cotransporter and returned to the Glycoehollc acid blood via a separate transport system. [Note: Uthocholic acid is only (a con)ugatecl blle All} poor1y absorbed.] They are efficiendy taken up from blood by the hepa- tocytes via an isoform of the cotransporter and reused. [Note: Albumin binds bile salts and transports them through the blood as was seen with FA (see p. 181).] The continuous process of secretion of bile salts into the bile, their passage through the duodenum where some ----------- --- Ch9nodeoxychollc acid I? H Taurtne /C- N- CH:zCH~il are deconjugated then dehydroxylated to secondary bile salts, their uptake in the ileum, and their subsequent return to the liver as a mixture of primary and secondary forms is termed the enterohepatic circulation (Fig. 18.11). Between 15 and 30 g of bile salts are secreted from the H liver into the duodenum each day, yet only -0.5 g ( 90% of the TAG in its core is degraded by LPL, the particle decreases in size and increases in density. In addition, the C apoli- poproteins (but not apo B or E) are returned to HDL. The remaining particle, called a remnant, is rapidly removed from the ciroulation by the liver, whose cell membranes contain lipoprotein receptors that recognize apo E (see Fig. 18.16). Chyfomicron remnants bind to these receptors and are taken into the hepatocytes by endocyto- sis. The endocytosed vesicle then fuses with a lysosome, and the 230 18. Cholesterol, Lipoprotein, and Steroid Metabolism apolipoproteins, cholesteryl esters, and other components of the remnant are hydrolytically degraded, releasing amino acids, free cholesterol, and FA. The receptor is recycled. [Note: The mecha- nism of receptor-mediated endocytosis is illustrated for LDL in Fig. 18.20.] C. Vary-low-density llpoproteln matabollsm VLDL are produced in the liver (Fig. 18.17). They are composed pre- dominantly of endogenous TAG (-60%), and their function is to cany this lipid from the liver (site of synthesis) to the peripheral tissues. There, the TAG is degraded by LPL, as discussed for chylomicrons (seep. 228). [Note: Nonalcoholic fatty liver (hepatic steatosis) occurs in conditions in which there is an imbalance between hepatic TAG synthesis and the secretion of VLDL. Such conditions include obesity and type 2 diabetes mellitus {see p. 343).] 1. Release from the llver: VLDL are secreted directly into the blood by the liver as nascent particles containing apo B-100. They must obtain apo C-11 and apo E from circulating HDL (see Fig. 18.17). LIVER ~ Nucent VLDL ~ ~ Apo C-11 and apo E u.,. tnlnafwrr9d TISSUES (tor example, adipose) from HDL to the nucentVLDL VLDL i:::1ll Exlr8C911ular ca 1/poproltJ/n 0 Liver HCntlN nucent, TAO-rich YLDL partlclM containing prtmarlly endogenoualy ayntheelnd llplde. llpaH, acHvated by apo C-11, d8gradn1t. TAGlnVLDL.....__.,_ _....j~ Free fatty ApoC-11 acids andapo E (lo HDL) ~ EL G~rol \ To~ER ~'=- '' '\, LDL n Apo C-11 and apo E Ii.I ue 1'9tumlld to HDL. Figure 18.17 Metabollsm of very-low--denstty Hpoproteln (VLDL) and low-density llpoproteln (LDL) particles. Apo B-100, C-11, and E are apollpoprotelns found as components of plasma llpoproteln particles. The partlcles are not drawn to scale {see Fig. 18.13 for details of their size and density). [Note: IDL can also be taken up by liwr.] TAG= triacylglycerol; HDL and IDL =high- and intermediate-density lipoproteins; C =cholesterol; CE= cholesteryl ester. VI. Plasma Lipoproteins 231 As with chylomicrons, apo C-11 is required for activation of LPL. Very-low-density llpoproteln [Note: Abetalipoproteinemia is a rare hypolipoproteinemia caused (VLDL) by a defect in MTP, leading to an inability to load apo B with lipid. Consequently, few VLDL or chylomicrons are formed, and TAG accumulates in the liver and intestine. Absorption of fat-soluble vitamins is decreased. LDL are low.] 2. Modification In the clrculatlon: As VLDL pass through the cir- culation, TAG is degraded by LPL, causing the VLDL to decrease in size and become denser. Surface components, including the C and E apolipoproteins, are returned to HDL, but the par- ticles retain apo B-100. Additionally, some TAG are transferred from VLDL to HDL in an exchange reaction that concomitantly transfers cholesteryl esters from HDL to VLDL. This exchange is accomplished by cholesteryl ester transfer protein (CETP'), as shown in Figure 18.18. 3. Conversion to low-density llpoprotelns: With these modifica- tions, the VLDL is converted in the plasma to LDL. Intermediate- density lipoproteins (IDL) of varying sizes are formed during this transition. IDL can also be taken up by liver cells through recep- tor-mediated endocytosis that uses apo E as the ligand. Apo E is normally present in three isoforms, E-2 (the least common), E-3 High-density lipoprotein (the most common), and E-4. Apo E-2 binds poorly to receptors, (HDL) and patients who are homozygotic for apo E-2 are deficient in the clearance of IDL and chylomicron remnants. These individu- als have familial type Ill hyperlipoproteinemia (familial dysbetali- Figure 18.18 poproteinemia or broad beta disease), with hypercholesterolemia Transfer of cholesteryl ester (CE) and premature atherosclerosis. [Note: The apo E-4 isoform con- from HDL to VLDL in exchange for triacylglycerol (TAG). fers increased susceptibility to an earlier age of onset of the lats- onset form of Alzheimer disease. The effect is dose dependent, with homozygotes being at greatest risk. Estimates of the risk vary.] D. Low-density llpoproteln metabollsm LDL particles contain much less TAG than their VLDL predecessors and have a high concentration of cholesterol and cholesteryl esters (Fig. 18.19). About 70% of plasma cholesterol is in LDL. 1. Receptor-mediated endocytosls: The primary function of LDL particles is to provide cholesterol to the peripheral tissues (or return it to the liver). They do so by binding to plasma membrane LDL receptors that recognize apo B-100 (but not apo B-48). Because these LDL receptors can also bind apo E, they are known as apo B-100/apo E receptors. A summary of the uptake and degradation of LDL particles is presented in Figure 18.20. [Note: The numbers in brackets below refer to corresponding numbers on that figure.] A similar mechanism of receptor-mediated endocytosis is used for the uptake and degradation of chylomicron remnants and IDL by the liver. LDL receptors are negatively charged glycoproteins that are clus- tered in pits on cell membranes. The cytosolic side of the pit is coated with the protein clathrin, which stabilizes the pit. 232 18. Cholesterol, Lipoprotein, and Steroid Metabolism After binding, the LDL-receptor complex is endocytosed. [Note: Defects in the synthesis of functional LDL receptors causes a sig- nificant elevation in plasma LDL-C. Patients with such deficien- cies have type Ila hyperlipidemia (familial hypercholesterolemia [FH]) and premature atherosclerosis. Autosomal dominant hyper- cholesterolemia can also be caused by defects in apo B-100 that reduce its binding to the receptor and by increased activity of a protease, proprotein convertase subtilisin/kexin type 9 (PCSK~, which promotes internalization and lysosomal degradation of the Chylomlcron receptor. PCSK9 inhibitors are now available for the treatment of hypercholesterolemia.] The vesicle containing LDL loses its clathrin coat and fuses with other similar vesicles, forming larger vesicles called endosomes. The pH of the endosome falls (due to the proton-pumping activity of endosomal ATPase), which allows separation of the LDL from its receptor. The receptors then migrate to one side of the endo- some, whereas the LDL stay free within the lumen of the vesicle. Yery·low-de lty The receptors can be recycled, whereas 1he lipoprotein remnants llpoproteln (VLDL) in the vesicle are transferred to lysosomes and degraded by lyso- somal acid hydrolasss, releasing free cholesterol, amino acids, FA, and phospholipids. These compounds can be reutilized by the cell. [Note: Lysosomal storage diseases result from rare auto- somal-recessive deficiencies in the ability to hydrolyze lysosomal cholesteryl esters (Wolman disease) or to transport free choles- terol out of the lysosome (Niemann-Pick disease, type C).] 2. Endocytosed cholesterol and cholesterol homeostasis: The chylomicron remnant-, IDL-, and LDL-derived cholesterol affects Low-denalty llpoproteln (LDL) cellular cholesterol content in several ways (see Fig. 18.20). First, expression of the gene for HMG CcA reductase is inhibited by high cholesterol, and de nmm cholesterol synthesis decreases as a result. Additionally, degradation of the reductase is acceler- ated. Second, synthesis of new LDL receptor protein is reduced by decreasing 1he expression of the LDL receptor gene, thus lim- iting further entry of LDL-C into cells. [Note: As was seen with 1he reductass gene (see p. 222), transcriptional regulation of the LDL receptor gene involves an SRE and SREBP-2. This allows Hlgh·den lty llpaproteln (HDL) coordinate regulation of the expression of these pro1eins.] Third, if the cholesterol is not required immediately for some structural or synthetic purpose, it is esterified by acy/ CoA:cholsstsrol acyl- D TRIACYLGLYCEROL transferase (ACA1). ACAT 1ransfers a FA from a fatty acyl CoA to PROTat cholesterol, producing a cholesteryl ester that can be stored in the D PHOSPHOUPIDS cell (Fig. 18.21). The activity of ACATis enhanced in the presence of increased intracellular cholesterol. D CHOLESTEROL AND CHOLESTERYL ESTERS 3. Uptake by macrophage scavenger receptors: In addition to 1he highly specific and regulated receptor-mediated pathway for LDL uptake described above, macrophages possess high levels Figure 18.19 of scavenger receptor activity. These receptors, known as scav- Composition of 1he plasma lipoprotein enger receptor class A (SR-A), can bind a broad range of ligands particles. Note the high concentration and mediate the endocytosis of chemically modified LDL in which of cholesterol and cholesteryl esters In LDL. 1he lipid or apo B component has been oxidized. Unlike the LDL receptor, the scavenger receptor is not downregulated in response VI. Plasma Lipoproteins 233 LDL 't'-...lllf'l-t. -- Choleeteryl 881er ~......-- Apollpoproteln 1>100 LDL receptor ---> / PLASMA MEMBRANE COATEDPrr i [2J/. 'l\. Clathrln \ 0. (t) ~- 0 cQ ~ Endollome ~ ~ GOLGIAPPAAATUS p~ ~ Clathrln SYNTltESIS OF SYNTHESIS OF LDL RECEPTORS CHOLESTEROL Fattyack19 OVERSUPPLY CELL MEMBRANE, OF CHOLESTEROL STEROID HORMONES, BILE ACIDS ENDOPLASMIC RmCULUM ' Choleeterol STORAGE OF Receptor protaln CHOLESTEAVL ESTERS Figure 18.20 Cellular uptake and degradation of low-density lipoprotein (LDL) particles. [Note: Oversupply of cholesterol accelerates the degradation of HMG CoA 1'9ductaS6. It also decreases transcription of its gene as seen with the LDL receptor.] ACAT =acyl CoA:cholsstsrol acylttansfsrase; HMG CoA =hydrox.ymethylglutaryl coenzyme A; mRNA =messenger RNA. 234 18. Cholesterol, Lipoprotein, and Steroid Metabolism to increased intracellular cholesterol. Cholesteryl esters accumu- late in macrophages and cause their transformation into -roam cells, which participate in the formation of atherosclerotic plaque (Fig. 18.22). LDL-C is the primary cause of atherosclerosis. E. High-density llpoproteln metabollsm HO HDL comprise a heterogeneous family of lipoproteins with a complex metabolism that is not yet completely understood. HDL particles are Ac)'t= r Choleeterol (ACA1JrCoA Fatty acyl CoA formed in the blood by the addition of lipid to apo A-1, an apolipopro- tein made and secreted by the liver and intestine. Apo A-1 accounts for - 70% of the apolipoproteins in HDL. HDL perform a number of important functions, including the following. 1. Apollpoproteln supply: HDL particles serve as a circulating res- ervoir of apo C-11 (the apolipoprotein that is transferred to VLDL and chytomicrons and is an activator of LPL) and apo E (the apo- lipoprotein required for the receptor-mediated endocytosis of IDL and chylomicron remnants). 0II R - C- 0 2. Nonesterlfled cholesterol uptake: Nascent HDLare disc-shaped particles containing primarily phospholipid (largely PC) and apo A, C, and E. They take up cholesterol from nonhepatic (peripheral) Choleeterylester tissues and return it to the liver as cholesteryl esters (Fig. 18.23). [Note: HDL particles are excellent acceptors of nonesterified cho- Figure 1a.21 lesterol as a result of their high concentration of phospholipids, Synthesis of intracellular cholesteryl which are important solubilizers of cholesterol.] ester by ACAT. [Note: L6cithin: cholssterol acyl tnmsfstase S. Cholesterol esterlftcatlon: The cholesterol taken up by HDL is (LCA7) is 1he extracellular enzyme immediately esterified by the plasma enzyme leclthin:cholesterol that esterifies cholesterol using acyltransferase (LCAT, also known as PCAT, in which P stands for phosphatidylcholine (lecithin) as phosphatidylcholine, the source of the FA). This enzyme is synthe- the source of the fatty acid.] CoA = coenzymeA. sized and secreted by the liver. LCAT binds to nascent HDL and is activated by apo A-1. LCAT transfers the FA from carbon 2 of PC to cholesterol. This produces a hydrophobic cholesteryl ester, which is sequestered in the core of the HDL, and lysophosphati- dylcholine, which binds to albumin. [Note: Esterification maintains the cholesterol concentration gradient, allowing continued efflux of cholesterol to HDL.] As the discoidal nascent HDL accumulates cholesteryl esters, it first becomes a spherical, relatively choles- teryl ester-poor HDL3 and, eventually, a cholesteryl ester-rich HDL2 particle that carries these esters to the liver. Hepatic lipase, which degrades TAG and phospholipids, participates in the conver- sion of HDL.2 to HDL3 (see Fig. 18.23). CETP (see p. 231) trans- fers some of the cholesteryl esters from HDL to VLDL in exchange for TAG, relieving product inhibition of LCAT. Because VLDL are catabolized to LDL, the cholesteryl esters transferred by CETP are ultimately taken up by the liver (see p. 231). 4. Reverse cholesterol transport: The selective transfer of choles- terol from peripheral cells to HDL and from HDL to the liver for bile acid synthesis or disposal via the bile is a key component of cholesterol homeostasis. This process of reverse cholesterol trans- port (ACT) is, in part, the basis for the inverse relationship seen between plasma HDL concentration and atherosclerosis and for VI. Plasma Lipoproteins 235 Q r.:I High-affinity receptor pecltlc LDL 11!1 for LDL ue downreaulsted when the cell has sufficient Vitamin E ~0 tlo choleeterol. superoxlde -«·"'"""'' Aacorblc acid Nitric oxide p.carotene Hydrog.. peroxide Other oxldanbl O Other antloxldanbl from the diet \ MACROPHAGE n Low-efllnlty, nonspecHlc, and Iii.II nonregutated ace.venger receptore 1ake up rnodlfed (o.xldlzed) LDL (oxLDL). SlllOOTH MUSCLE CELL 0 In reeponae to endothellal ln)ury (ceused at lust In pert by Oxidized LDL) \ monocytee adhere to endothellal cella, move 10 the aubendothellum {lntlma), and are converted Into macrophages. EXTRACELLULAR MAlRIX EJ Foam cell accumulate, rel8811lng growth factors and cytoklnes ttwt 8tlmulate the migration of emooth r:1I Macrophages consume excess m11&cle cells (SMC) from the media U modified (oxidized) llpoproteln, to the tntlma. There, the SMC MONOCYTES beccmlng foam cells. ta proliferate; produce collagen; and up lipid, potenllally becoming foamcella. INTERIOR OF ARTERY Figure 18.22 Role of oxidized low-density lipoprotein (LDL) particles in plaque formation in an arterial wall. the designation of HDL as the *good~ cholesterol carrier. [Note: Exercise and estrogen raise HDL levels.] ACT involves efflux of cholesterol from peripheral cells to HDL, esterification of the cho- lesterol by LCAT, binding of the cholesteryl ester-rich HDL (HDL2) to liver (and, perhaps, steroidogenic cells), selective transfer of the cholesteryl esters into these cells, and release of lipid-depleted HDL (HDL3). The efflux of cholesterol from peripheral cells is medi- ated primarily by the transport protein ABCA1. [Note: Tangier dis- ease is a very rare deficiency of ABCA1 and is characterized by the virtual absence of HDL particles due to degradation of lipid-poor apo A-1.] Cholesteryl ester uptake by the liver is mediated by the cell-surface receptor SR-B1 (scavenger receptor class B type 1) 236 18. Cholesterol, Lipoprotein, and Steroid Metabolism LIVER SMALL INTESTINE rm t t LDL.. IDL t 0 PERIPHERAL 0 TISSUES VLDL YLDL Figure 18.23 Metabolism of high-density lipoprotein (HDL) particles. Apo= apolipoprotein; ABCA1 =ATP-binding cassette transport protein; C;:;: cholesterol; CE;:;: cholesteryl ester; LCAT =lecithin:cholsstsrol acyltransfBrasB; VLDL, IDL, and LDL =very-low-, = intermediate-, and low-density lipoproteins; CETP = cholesteryf ester transfer protsin; SR-B1 scavenger receptor 81. 1hat binds HDL (seep. 232 for SR-A receptors). The HDL particle itself is not taken up. Instead, there is selective uptake of the cho- lesteryl ester from the HDL particle. [Note: Low HDL-C is a risk factor for atherosclerosis.] ABCA1 is an ATP-binding cassette (ABC} protein. ABC pro- teins use energy from ATP hydrolysis to transport materials, including lipids, in and out of cells and across intracellu· lar compartments. In addition to Tangier disease, defects in speclftc ABC proteins result In sltosterolemla, cys11c fibrosis, X-llnk:ed adrenoleukodystrophy, respiratory distress syndrome due to decreased surfactant secretion, and liver disease due to decreased bile salt secretion. F. Lipoprotein (a) and heart disease Lipoprotein (a), or Lp(a), is nearly identical in structure to an LDL par- ticle. Its distinguishing feature is the presence of an additional apoli- poprotein molecule, apo(a), which is covalently linked at a single site to apo B-1 oo. Circulating levels of Lp(a) are determined primarily by genetics. However, factors such as diet may play some role, as trans FA have been reported to increase it. The physiologic function of Lp(a) is unknown. When present in large quantities in the plasma, Lp(a) is associated with an increased risk of coronary heart disease. [Note: Apo(a) is structurally homologous to plasminogen, the precursor of a VII. Steroid Hormones 237 blood prot98Se whose target is fibrin, 1he main protein component of blood clots {see Chapter 35 online). It is hypothesized that elevated Lp(a) slows the breakdown of blood clots that trigger heart attacks because it competes with plasminogen for binding to fibrin.] Niacin reduces Lp(a), as well as LDL-C and TAG, and raises HDL-C. VII. STEROID HORMONES Cholesterol Cholesterol is the precursor of all classes of steroid hormones: glucocorti- coids (for example, cortisol), mineraJocorticoids (for example, aldosterone), i and the sex hormones (that is, androgens, estrogens, and progestins), as shown in Figure 18.24. [Note: Glucocorticoids and mineraJocorticoids are collectively called corticosteroids.] Synthesis and secretion occur in the adrenal cortex (cortisol, aldosterone, and androgens), ovaries and pla- HO centa (estrogens and progestins), and testes (testosterone). Steroid hor- mones are transported by the blood from their sites of synthesis to their PNgnenolone target organs. Because of their hydrophobicity, they must be complexed with a plasma protein. Albumin can act as a nonspecific carrier and does carry aldosterone. However, specific steroid-carrier plasma proteins bind the steroid hormones more tightly than does albumin (for example, corti- costeroid-binding globulin, or transcortin, is responsible for transporting cortisol}. A number of genetic diseases are caused by deficiencies in spe- cific steps in the biosynthesis of steroid hormones. Some representative diseases are described in Figure 18.25. Pragulerone A. Synthesis II' Synthesis involves shortening the hydrocarbon CHrPH JI' chain of cholesterol and hydroxylating the steroid 0 nucleus. The initial and rate-limiting reaction con- ,,,..,OH verts cholesterol to the 21-carbon pregnenolone. It is catalyzed by the cholesterol side-chain cleavage enzyme, a cytochrome P450 {CYP) mixed function oxidase of the inner mitochondrial membrane (see 0 p. 149} that is also known as P450scc and desmo- CortlllOI lsse. NADPH and 02 are required for the reaction. ( glucocortlcold) The cholesterol substrate can be newly synthe- sized, taken up from lipoproteins, or released by an esterase from cholesteryl esters stored in the cytosol of steroidogenic tissues. The cholesterol moves to 1he outer mitochondrial membrane. An important control point is the subsequent move- ment from the outer to the inner mitochondrial membrane. This process is mediated by StAR (ste- roidogenic acute regulatory) protein. Pregnenolone Eatradlol is the parent compound for all steroid hormones Aldoeterone (an estrogen) ( mlneralocortlcold) (see Fig. 18.25). It is oxidized and then isomer- ized to progesterone, which is further modified to the other steroid hormones by CYP protein-cata- lyzed hydroxylation reactions in 1he SER and mito- chondria. A defect in the activity or amount of an Figure 18.24 enzyme in 1his pathway can lead to a deficiency Key steroid hormones. in the synthesis of hormones beyond the affected 238 18. Cholesterol, Lipoprotein, and Steroid Metabolism CONGENITAL ADRENAL HVPERPLASIAS (CAH) STEROID HORMONE SYNTHESIS 3-/J-HYDROXYSTEROID DEHYDROGENASEDEFICIENCY Cholaetarol (1:1 C) Ylrtualty no glucocortlcolda, mlneralocortlcolda, acttv. androgeRS, or estrogens Salt excretion In urine NADPH Ot tIDesmolase (CYP1fA, Female-like genitalia ~ P450sGC) +.. _ 17-a-HYDROJCYLASEDEFICIENCY Pregnenolone (21 C) Ylrtualty no eex hormones or cortlaol Increased production of mlneralocortlcolds causing aodlum and ftuld retlantlon and, thandore, hypertanalon Female-like genltalla i~~ 11·/l1·HYDROXYLASEDEFICIENCY 11·Deoxycortlcost.erone(21 C) 11-Deoxycortleol (21 C) Andl08lenedlone (19 C) t Decreased production of corllaol, TI 1"~ aldatarone, and cortlcomrone 11-/J-Hydroxy/ase lnc1'88118d production of deoxy- (CYP1181) eoltlcost.wone causing fluid retention (became thla hormone 11~ supp"'88N the renln-englotensln Cortlcoaterone rir&Q T88tostwone (19 C) tt ayatem, It caueea low-renln I hypertension) ~SJ!I~ (CYP11521 Atotnatase Overproduction of androgens causing maacullnl:ratlon and vlrlllzallon {as with (CYP19) 21-a-hydroxl.s deficiency) Aldoet.wone (21 C) Cortisol {21 C) Eslrlldlol (18 C} Figure 18.25 Steroid hormone synthesis and associated diseases. [Note: 3-{J-HydroxystBfOid d6hydrogsnase, CYP17. and CYP1182 are multifunctional enzymes. Synthesis of testosterone and the estrogens occurs primarily outside of the adrenal gland.] NADPH = nlcoUnamlde adenine dlnucleotlde phosphate; CYP = cytochrome P450. step and to an excess in the hormones or metabolites before that step. Because all members of the pathway have potent biologic activ- ity, serious metabolic imbalances occur with enzyme deficiencies (see Fig. 18.25). Collectively, these disorders are known as the con- genital adrenal hyperplasias (CAH), because they result in enlarged adrenals. [Note: Addison disease, due to autoimmune destruction of the adrenal cortex, is characterized by adrenocortical insufficiency.] B. Adrenal cortical steroid hormonaa Steroid hormones are synthesized and secreted in response to hor- monal signals. The corticosteroids and androgens are made in different VII. Steroid Hormones 239 regions of the adrenal cortex and are secreted into blood in response HYPOTiiAl..AMUS to different signals. [Note: The adrenal medulla makes catecholamines (seep. 285).] 1. Cortlsol: Its production in the middle layer (zona fasciculata) of the adrenal cortex is controlled by the hypothalamus, to which the pituitary gland is attached {Fig. 18.26). In response to severe stress (for example, infection), corticotropin-releasing hormone (CRH}, produced by the hypothalamus, travels through capillar- ies to the anterior lobe of the pituitary, where it induces the pro- duction and secretion of adrenocorticotropic hormone (ACTH), a peptide. ACTH stimulates the adrenal cortex to synthesize and secrete the glucocorticoid cortisol, the stress hormone. [Note: LUTEINIZING HORMONE ACTH binds to a membrane G protein-coupled receptor, result- Induces teatoeterone ing in cyclic AMP (cAMP) produc1ion and ac1ivation of protein aynthnle In Leydlg C911a kinase A {[PKAJ seep. 94). PKA phosphorylates and activates oftllet..u. both the esterase that converts cholesteryl ester to free cho- Induces ovulation In fem11lee lesterol and StAR protein.] Cortisol allows the body to respond Stlmulatea..1rogen and to stress through its effects on intermediary metabolism (for progesterone synthesis In the corpua luteum example, increased gluconeogenesis) and the inflammatory and immune responses {which are decreased). As cortisol levels rise, FOLLICLE-sTIMULATING the release of CRH and ACTH is inhibited. [Note: The reduction HORMONE of cortisol in CAH results in a rise in ACTH that causes adrenal StlmulatH ncretlon of eetrogens In females or hyperplasia.] androgena In males 2. Aldoaterone: Its production in the outer layer (zona glomeru- Promol88 apermato- gennle In 199t9s and losa) of the adrenal cortex is induced by a decrease in 1he oogenesls In ovartea plasma Na+/potassium (K+) ratio and by the hormone angiotensin II (Ang-II). Ang-II (an octapeptide) is produced from angioten- ADRENOCOATICOTROPIC sin I ([Ang-I] a decapeptide) by angiotensin-convsrting enzyme HORMONE (ACE), an enzyme found predominantly in the lungs but also Stfmulatee eeel1dlon of distributed widely in the body. [Note: Ang-I is produced in the g lucocortlcold blood by cleavage of an inactive precursor, angiotensinogen, secreted by the liver. Cleavage is catalyzed by rsnin, made and secreted by the kidneys.] Ang-II binds to cell surface receptors. However, in contrast to ACTH, its effects are mediated through the phosphatidylinositol 4,5-bisphosphate pathway (see p. 205) and not by cAMP. Aldosterone's primary effect is on the kidney tubules, where it stimulates Na+ and water uptake and K+ excre- tion (Fig. 18.27). [Note: An effect of aldosterone is an increase in blood pressure. Competitive inhibitors of ACE are used to treat rsnin-dependent hypertension.] 3. Androgens: Both the inner (zona reticularis) and middle layers of the adrenal cortex produce androgens, primarily dehydroepi- OVARIES androsterone and androstenedione. Although adrenal andro- gens themselves are weak, they are converted by aromatase (CYP1!1} to testosterone, a stronger androgen, in the testes and to estrogens in the ovaries (primarily) of premenopausal women. [Note: Postmenopausal women produce estrogen at TESTIS extragonadal sites such as the breast. Aromatase inhibitors are used in the treatment of estrogen-responsive breast cancer in Figure 18.26 these women.] Pituitary hormone sttmulatfon of steroid hormone synthesis and secretion. 240 18. Cholesterol, Lipoprotein, and Steroid Metabolism c. Gonadal steroid hormones ADRENAL CORTEX The testes and ovaries (gonads) synthesize hormones necessary for sexual differentiation and reproduction. A single hypothalamio- ,_4~1 releasing factor, gonadotropin-releasing hormone, stimulates the anterior pituitary to release the glycoproteins luteinizing hormone (LH) and follicle-stimulating hormone (FSH). Like ACTH, LH and FSH bind to surface receptors and cause an increase in cAMP. LH stimulates ALDOSTERONE the testes to produce testosterone and the ovaries to produce estro- StlmulatH renal n1111baorptlon gens and progesterone (see Fig. 18.27}. FSH regulates the growth of of Na+ and e.xcredon of~ ovarian follicles and stimulates testicular spermatogenesis. CORTISOL D. Mechanism lncreaHS gluconeogeneal Antl·lnflammatory action Each steroid hormone diffuses across the plasma membrane of its Protein breakdown In muacle target cell and binds to a specific cytosolic or nuclear receptor. These receptor-ligand complexes accumulate in the nucleus, dimerize, and bind to specific regulatory DNA sequences (hormone response ~ elements [HRE]) in association with coactivator proteins, thereby causing increased transcription of targeted genes (Fig. 18.28}. An HRE is found in the promoter or an enhancer element (see p. 440) for genes that respond to a specific steroid hormone, thus insur- ing coordinated regulation of these genes. Hormone-receptor OVARIES complexes can also inhibit transcription in association with core- pressors. [Note: The binding of a hormone to its receptor causes ESTROGENS Control menstrual cycle a conformational change in the receptor that uncovers its DNA- Promota development of female binding domain, allowing the complex to interact through a zinc fin- secondary HX Characteitetlcs ger motif with the appropriate DNA sequence. Receptors for the steroid hormones, plus those for thyroid hormone, retinoic acid (see PROC1ESTERONE p. 386), and 1,25-dihydroxycholecalciferol (vitamin D; see p. 390), are members of a superfamily of structurally related gene regula- Secretory phae of uterus and mammary gland tors that function in a similar way.] Implantation and maturation of fertlllzed ovum E. Further metabolism Steroid hormones are generally converted into inactive metabolic excretion products in the liver. Reactions include reduction of unsat- urated bonds and the introduction of additional hydroxyl groups. The. G~ resulting structures are made more soluble by conjugation with gluc- uronic acid or sulfate (from 3'-phosphoadenosyl-5'-phosphosulfate; frTESTIS see p. 162). These conjugated metabolites are fairly water soluble and do not need protein carriers. They are eliminated in feces and urine. TESTOSTERONE Stimulates spennatogen ls VIII. CHAPTER SUMMARY Promotee development of male eecondary MX chanurterlstlce Promotee anabollem Choleaterol is a hydrophobic compound, with a single hydroxyl group Maecullnlzatlon of the r.tus located at carbon 3 of the A ring, to which a fatty acid (FA) can be attached, producing an even more hydrophobic choluteryl ester. Cholesterol is Figure 18.27 syn1hesized by virtually all human tissues, although primarily by the llver, Actions of steroid hormones. Na+ = Intestine, adrenal cortex, and reproductive tlsaun (Fig. 18.29). All the = sodium; K potassium. VIII. Chapter Summary 241 carbon atoms are provided byacetyl coenzymeA (CoA), and nlcotlnamlde adenine dlnucleotlde phosphate provides the reducing equivalents. The pathway is driven by hydrolysis of the high-energy thioester bond of acetyl CoA and the terminal phosphate bond of ATP. Synthesis requires Slerold hormone enzymes of the cytosol, smooth endoplasmic reticulum (SER), and pen>Xlsomes. The rate-limiting and regulated S1ep in cholesterol synthesis is catalyzed by the SER-membrane protein hydrmcymelhylglutaryl coenzyme A (HMG CoA) l8ductas8, which produces llMMllonate from HMG CoA. The enzyme is regulated by a number of mechanisms: 1) increased expreeelon of 1he reductase gene when cholesterol levels are low, via the transcription factor, eterol regulatory element-binding protein·2 (SREBP·2), bound to a aterol regulatory element (SRE), resulting in increased enzyme and, 1herefore, cholesterol, synthesis; 2} accelerated degradation of the reductase protein when cholesterol levels are high; 3) phosphorylation (causing Inactivation of reductase activity) by adenoslne monophosphat8-41Cthtattld pt0teln kinase [AlfPK] and dephosphorylatlon (activation) by a phasphoprofllln phollphata89', and 4) hormonal regulation by Insulin and glucagon. Stallns are competitive lnhlbltora of HMG CoA rsductase. These drugs are used to decrease plasma cholesterol in patients with hypercholesterolemla. The ring structure of cholesterol cannot be degraded in humans. 9lerold honnone- receptor complex Cholesterol is eliminated from the body either by conversion to bile salts or by secretion into the blle. Biie salts and phoaphatldylchollne (PC) are quantltatlvely the moS1 Important organic components of blle. The rate-limiting step in bile acid synthesis is catalyzed by choluterot-7- a·hydroxy/ase, which is inhibited by blle acids. Before the bile acids leave the liver, they are conjugated to a molecule of either glyc:lne or taurlne, producing the conjugated blle salts glycochollc or taurochollc: acid and glycochenodeoxychollc ortaurochenodeoxychollc acid. Bile salts{deprotonated}are moreamphipathicthan bile acids (protonated}and, therefore, are more effective emuleifiere of dietary fat. Intestinal bacteria can remove the glycine and taurine as well as a hydroxyl group from the steroid nucleus, producing the secondary blle nits, deoxychollc and llthochollc acids. Bile salts are efficiently reabsorbed {>95%) in the Binding of the eta'old Intestinal Ileum by a sodlum-blle salt cotranaporter, returned to the honnone-t'eceptor blood, and carried by albumln back to the liver where they are taken up 0 complex to the HRE of the enhancet by the hepatic isoform of the cotransporter and reused (enterohepatlc region aetlvatea the clrculatlon, which blla acid aquestranta reduce). If more cholesterol gene promoter, enters the bile than can be solubilized by the available bile salts and PC, causing traMcrlptlon. cholastarol gallstone dls8Ue (choleltthlasls) can occur. The plasma lipoproteins {see Rg. 18.29) include chylomlcrona, very- Promoter region low-danslly llpoprotelns (VLDL), Intermediate-density llpoprotelns (IDL), low-density llpoprolelns {LDL), and high-density llpoprvtelns (HDL}. They function to keep lipids (primarily trlacylglycarol [TAG] and chalesteryl asters) soluble as they transport them between tissues. Lipoproteins are composed of a neutral llpld (TAG, cholasteryl asters, or both) core surrounded by a shell of amphlpathlc apollpoprotelns, phoephollpld, and noneetertfled cholesterol. Chylomlcrone are mANA assembled in inteetinal mucoeel cell from dietary lipid (primarily TAG}. Each nascent chylomicron particle has one molecule of apolipoprotein (apo} B-48. They are released from the cells into the lymphatic system Flgure 18.28 and travel to the blood, where they receive apo C-11 and apo E from HDL. Activation of transcription by Apo C·ll activates endothelial llpoproteln llpus (LPL}, which degrades interaction of steroid hormone- receptor complex with hormone the TAG in chylomicrons to FA and glycerol. The FA that are released are response element (HRE}. The stored (In adipose tissue) or used for energy (In muscle). The glycerol ls receptor contains domains that bind the hormone, DNA. and coactivating = proteins. mRNA messenger RNA. 242 18. Cholesterol, Lipoprotein, and Steroid Metabolism metabolized by the liver. Patients with a deficiency of LPL or apo C-11 show a dramatic accumulation of chylomicrons in the plasma (type I hyperllpoprotelnemla or famlllal chylomlcronemla} even if fasted. After most of the TAG is removed, apo C·ll is returned to HDL, and the chylomlcron remnant, carrying most of the dietary cholesterol, binds to a liver receptor that recognizes apo E. The particle is endocytond, and its contents degraded by lysoeomal enzymes. Defective uptake of these remnants (and IDL) causes type Ill hyperllpoprotalnemla or dyabatallpoprotelnamla. Nascent VLDL are produced In the liver and are composed predominantly of TAG. They contain a single molecule of apo B-100. Uke chylomicrons, VLDL receive apo C·ll and apo E from HDL in the plasma. VLDL carry hepatic TAG to the peripheral tissues where LPL degrades the lipid. Additionally, the VLOL particle receives cholesteryl eaters from HDL in exchange for TAG. This process is accomplished by cholestetyl ester transfer pmteln (CETPJ. VLDL in the plasma is first converted to IDL and then to LDL, a much smaller, denser particle. Apo C-11 and apo E are returned to HDL, but the LDL retains apo B-100, which is recognized by receptors on peripheral tissues and the liver. LOL undergo receptor-mediated endocytoela, and their contents are degraded in the lyeoeomea. The protease proptOteln convertaee aubtllltllnllculn type 9 (PCSK!TJ prevents receptor recycling. Defects in the synthesis of functional LDL receptors causes type Ila hypeltipoproteinemia (femlllal hypercholesterolemla [FH]). The endocytosed cholesterol decreases expression of HMG CoA r6ductase {and LDL receptors) through prevention of SREBP-2 binding to the SRE. Some of it can be esterified by ecyl CoA:chole#Jterol scyltnJn.,.,_ae (ACA1) and stored. HDL are created by llpldallon of apo A-1 synthesized in the liver and intestine. They have a number of functions, Including 1) serving as a clrculaUng reservoir of apo C-11 and apo E for chylomlcrons and VLDL; 2) removing cholesterol from peripheral tissues via ABCA1 and esterlfylng It using leclthln:chotesteml acyl transferase (LCAl), a liver-synthesized plasma enzyme that is activated by apo A-1; and 3) delivering these cholesteryl esters to the liver (raverae cholesterol transport} for uptake via scavenger racaptor·B1 (SR·B1). Cholesterol is the precursor of all classes of eteroid hormonee, which include glucocorticoida, mineralocorticoide, and the eex honnonee (andl'Qgene, eetrogene, and progeatina). Synthesis, using primarily cytochmme P450 mixed function oxldnes, occurs in the adrenal cortex {cortlaol in the zone faaclculata, aldosterone in the zone glomeruloaa, and androgens in the zone retlcularla), ovarlaa and placenta (estrogens and progastlna), and testes (testosterone). The lnldal and rate-limiting step Is the conversion of cholesterol to pregnenolone by the side-chain cleavage enzyme P450.t:e. Deficiencies In synthesis lead to congenital adrenal hyparplaala {CAH). Each steroid hormone diffuses across the plasma membrane of its target cell and binds to a specific intracellular receptor. These recaptor-ttormone complexes accumulate in the nucleus, dimerize, and bind to specific regulatory DNA sequences (hormone ntSponae elements) in association with coactivator proteins, thereby causing increased transcription of targeted genes. In association with corepressors, transcription is decreased. Study Questions Correct answer.. A. The reductaae is regulated by cova- Chooee the ONE beet anawer. lent phosphorylation and dephoephorylation. Depletion of ATP resuns in a rise in adenosine monophosphate (AMP), 18.1 Mice were genetically engineered to contain which activates AMP kinase (AMPK), 1hereby phosphory· hydroxymethytglutaryl coenzyme A reductase in which la11ng and Inactivating the reductase. In the abeence of the serine 871, a phosphorylation site, was replaced by serine, a common phosphorylation site, the enzyme can- alanine. Which of the following statements concerning not be phosphorylated by AMPK. Tha enzyme is also regu· the modified form of the enzyme is most likely to be lated physiologically 1hrough changes in transcription and correct? degradation and pharmacologically by statin drugs (com· pe11tlve Inhibitors), but none of these depends on ser1ne A. The enzyme is nonresponsive to ATP depletion. phosphorylallon. B. The enzyme is nonresponsive to statin drugs. C. The enzyme is nonresponsive to the sterol response element-sterol response element-binding protein system. D. The enzyme is unable to be degraded by the ubiquitin-proteasome system. VIII. Chapter Summary 243 Cholesterol synthesis 3.Acetyl CoA Major regulatory mechanlam: Rate-llmttlng Ste t atarol-dependant ragulatlon of gene expraulon cataJyzss HMO CoA t respdnds to ,-----A~---~ Drug target in therapy for hy~er HMGCoA ~, f'9duclas6 ~~Joo -+----t-~ ~ t ntracelluar dlole81e,;=] t Dlntac:elluar choleeter;=J cholesterolem1a by the stalln - - -- leads to leads to Mevalanlc cld t ~--~t_ _ _~ drugs t D SREBP·2 activity =1 nm SAEBP-2 actlvlly ~ occuntln 5C leads to ~ to t... 10C DGane trsnacription D Gene transcription t leads to leads to 15C t t t -IJ Amount of enzyme Amount of enzyme NADPH 30C Acetate as. __utlllns _ _-1 t Cho1Mt91'0I eole eou rce of carbon atoms 127CJ Lipoproteins Liver and lntMllne lnte81fne I I I generates ~r ~ i HDL LDL Chylomlcrona I I composed of VLDL compOsecJ of composed I t t i Ga~@llil 'U'&@ °"'®w lf&@ 11.@l.'/\7 @Dn@- ~n@Lr®Q l:al~h~1 @~0)]C®1@J©J functions to functions to t Dellver cholesterol from perlpharal Dellver endogenoua TAG to partphend " Dellver chalea1Brol to parlpharal Dellver dietary (axogenoua) TAG to tlu111111 to the llver llMllllll tissues and bl.ck partphenll tlau for allmlnatlon tollv Figure 18.29 Concept map for cholesterol and ttie lipoproteins. HMG CoA = hydroxymethylglutaryl coenzyme A; SREBP = sterol regulatory element-binding protein; HDL, LDL, and VLDL = high-, low-, and very-low-density lipoproteins; TAG = triacylglycerol; NADPH = nicotinamide adenine dinucleotide phosphate; C = carbon. 244 18. Cholesterol, Lipoprotein, and Steroid Metabolism 18.2 calculate the amount of cholesterol in the low-density Correct answer = D. The total cholesterol in the blood of a lipoproteins in an individual whose fasting blood gave fasted individual is equal to the sum of the cholesterol in the following lipid-panel test results: total cholesterol = low-density lipoproteins plus the cholesterol in high-density 300 mg/di, high-density lipoprotein cholesterol = 25 lipoproteins plus the cholesterol in very-low-density lipo- mgfdl, triglycerides= 150 mgfdl. proteins (VLDL). This last term is calculated by dividing the A. 55 mg/di triacylglycerol value by 5 because cholesterol