Transport of Substances Through the Cell Membrane Lecture PDF
Document Details
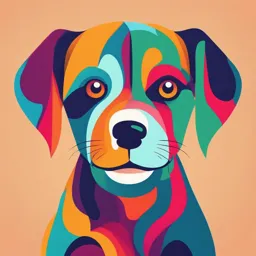
Uploaded by DurableRomanArt
Tags
Summary
This document covers the transport of substances through the cell membrane. It explains different mechanisms, such as diffusion, active transport, and vesicular transport, along with examples and illustrations. The text also delves into the structure and function of membrane components like lipids, glycolipids, and proteins. This is likely lecture material for an undergraduate-level biology or physiology course.
Full Transcript
Physiology (from the Greek physis = nature; logos = study) is the study of biological function—of how the body works, from cell to tissue, tissue to organ, organ to system, and of how the organism as a whole accomplishes particular tasks essential for life. In the study of physiology, the emphasis i...
Physiology (from the Greek physis = nature; logos = study) is the study of biological function—of how the body works, from cell to tissue, tissue to organ, organ to system, and of how the organism as a whole accomplishes particular tasks essential for life. In the study of physiology, the emphasis is on mechanisms—with questions that begin with the word how and answers that involve cause-and-effect sequences. Physiology is the study of body functions, their mechanisms and regulations in all living organisms. Human physiology is the study of functions of various cells, organs and organ systems of human body. Medical physiology is the application of knowledge of human physiology in the management of dysfunctions and diseases in human beings. Physiology is the key subject in medicine. Starting from the knowledge of body functions, physiology provides the concept of dysfunctions, the basis of understanding the disease processes and the insight into disease management and prevention. Physiology is the core of medical wisdom. Due to its enormous contribution to the growth of medical knowledge, the Nobel Prize in health sector has been designated as Nobel Prize in Physiology and Medicine. Physiology is the foundation of medical practice. Many clinical investigations related to neurological disorders, autonomic dysfunctions, cardiovascular and respiratory diseases, endocrinal, renal, reproductive and metabolic problems are carried out in the well- equipped laboratories of physiology departments. Further, many research investigations are conducted in physiology laboratories. In 1972, S. Singer and G. Nicolson revised the model in a simple but profound way: they proposed that the globular proteins are inserted into the lipid bilayer, with their nonpolar segments in contact with the nonpolar interior of the bilayer and their polar portions protruding out from the membrane surface In this model, called the fluid mosaic model, a mosaic of proteins float in the fluid lipid bilayer like boats on a pond Membrane Lipids Typically about 98% of the molecules in the membrane are lipids, and about 75% of the lipids are phospholipids. These amphiphilic molecules arrange themselves into a bilayer, with their hydrophilic phosphate-containing heads facing the water on each side of the membrane and their hydrophobic tails directed toward the center of the membrane, avoiding the water. Fatty acids are monocarboxylic acids that usually contain an even number of carbon atoms. Fatty acid chains may contain no double bonds—that is, be saturated—or contain one or more double bonds—that is, be mono- or polyunsaturated Glycolipids Glycolipids are molecules that contain both carbohydrate and lipid components. Like the phospholipid sphingomyelin, glycolipids are derivatives of ceramides in which a long-chain fatty acid is attached to the amino alcohol sphingosine. They are, therefore, more precisely called glycosphingolipids. Like the phospholipids, glycosphingolipids are essential components of all membranes in the body, but they are found in greatest amounts in nerve tissue. They are located in the outer leaflet of the plasma membrane, where they interact with the extracellular environment. As such, they play a role in the regulation of cellular interactions, growth, and development. Glycosphingolipids are antigenic, and they have been identified as a source of blood group antigens, various embryonic antigens specific for particular stages of fetal development, and some tumor antigens. [Note: The carbohydrate portion of a glycolipid is the antigenic determinant.] They also serve as cell surface receptors for cholera and tetanus toxins, as well as for certain viruses and microbes Prostaglandins and Related Compounds Prostaglandins, and the related compounds thromboxanes and leukotrienes, are collectively known as eicosanoids to reflect their origin from polyunsaturated fatty acids with 20 carbons They are extremely potent compounds that elicit a wide range of responses, both physiologic and pathologic. Although they have been compared to hormones in terms of their actions, eicosanoids differ from the true hormones in that they are produced in very small amounts in almost all tissues rather than in specialized glands. They also act locally rather than after transport in the blood to distant sites, as occurs with true hormones such as insulin Phosphatidylinositol Is a Precursor of Second Messengers it is cleaved into diacylglycerol and inositol trisphosphate, both of which act as internal signals or second messengers. Aspirin Cholesterol molecules, found among the fatty acid tails, constitute about 20% of the membrane lipids. By interacting with the phospholipids and “holding them still,” cholesterol can stiffen the membrane (make it less fluid) in spots. Higher concentrations of cholesterol, however, can increase membrane fluidity by preventing the phospholipids from becoming packed closely together. Steroid Hormones Steroids derived from cholesterol in animals include five families of hormones (the androgens, estrogens, progestins, glucocorticoids and mineralocorticoids) and bile acids Androgens such as testosterone and estrogens such as estradiol mediate the development of sexual characteristics and sexual function in animals Membrane Proteins Although proteins are only about 2% of the molecules of the plasma membrane, they are larger than lipids and constitute about 50% of the membrane weight. Some of them, called integral (transmembrane) proteins, pass through the membrane. They have hydrophilic regions in contact with the cytoplasm and extracellular fluid, and hydrophobic regions that pass back and forth through the lipid of the membrane Most integral proteins are glycoproteins, which are conjugated with oligosaccharides on the extracellular side of the membrane. Others are anchored to the cytoskeleton an intracellular system of tubules and filaments The functions of membrane proteins include the following Receptors The chemical signals by which cells communicate with each other (epinephrine, for example) often cannot enter the target cell, but bind to surface proteins called receptors Second-messenger systems. When a messenger binds to a surface receptor, it may trigger changes within the cell that produce a second messenger in the cytoplasm. Proteins also function as enzymes, catalyzing reactions at the surfaces of the membrane Peripheral proteins do not protrude into the phospholipid layer but adhere to the intracellular face of the membrane. Channel proteins. Channel proteins are integral proteins with pores that allow passage of water and hydrophilic solutes through the membrane. These gates open or close in response to three types of stimuli: ligand-regulated gates respond to chemical messengers, voltage-regulated gates to changes in electrical potential (voltage) across the plasma membrane, and mechanically regulated gates to physical stress on a cell, such as stretch and pressure. There are proteins that function as pumps, actively transporting ions across the membrane. Other proteins function as carriers, transporting substances down electrochemical gradients by facilitated diffusion Most integral proteins are glycoproteins, which are conjugated with oligosaccharides on the extracellular side of the membrane. Others are anchored to the cytoskeleton an intracellular system of tubules and filaments Peripheral proteins do not protrude into the phospholipid layer but adhere to the intracellular face of the membrane. A peripheral protein is typically associated with an integral protein and tethered to the cytoskeleton. For example, the anion exchanger, a major protein of the human erythrocyte membrane, is bound to the spectrin network that undergirds the membrane via a protein called ankyrin. Model of the organization of the erythrocyte membrane showing the peripheral and integral proteins and lipids. Spectrin is the predominant protein of the skeletal protein lattice. The functions of membrane proteins include the following Receptors The chemical signals by which cells communicate with each other (epinephrine, for example) often cannot enter the target cell, but bind to surface proteins called receptors Second-messenger systems. When a messenger binds to a surface receptor, it may trigger changes within the cell that produce a second messenger in the cytoplasm. Proteins also function as enzymes, catalyzing reactions at the surfaces of the membrane Channel proteins. Channel proteins are integral proteins with pores that allow passage of water and hydrophilic solutes through the membrane. Some channels are always open, while others are gates that open and close under different circumstances, thus determining when solutes can pass through These gates open or close in response to three types of stimuli: ligand-regulated gates respond to chemical messengers, voltage-regulated gates to changes in electrical potential (voltage) across the plasma membrane, and mechanically regulated gates to physical stress on a cell, such as stretch and pressure. There are proteins that function as pumps, actively transporting ions across the membrane. Other proteins function as carriers, transporting substances down electrochemical gradients by facilitated diffusion Transport of Substances Through the Cell Membrane The structure of membranes makes them quite selective about which molecules can pass through them. The hydrophobic interior of the lipid bilayer makes membranes highly impermeable to most polar molecules. This prevents water-soluble components of the cell from easily entering or escaping. “Diffusion” Versus “Active Transport.” Transport through the cell membrane, either directly through the lipid bilayer or through the proteins, occurs by one of two basic processes: diffusion or active transport. One of the most important factors that determines how rapidly a substance diffuses through the lipid bilayer is the lipid solubility of the substance. For instance, the lipid solubilities of oxygen, nitrogen, carbon dioxide, and alcohols are high, so that all these can dissolve directly in the lipid bilayer and diffuse through the cell membrane in the same manner that diffusion of water solutes occurs in a watery solution. Membrane Permeability The term permeable means that a structure permits the passage of substances through it, while impermeable means that a structure does not permit the passage of substances through it. The permeability of the plasma membrane to different substances varies. Plasma membranes permit some substances to pass more readily than others. This property of membranes is termed selective permeability The lipid bilayer portion of the membrane is permeable to nonpolar, uncharged molecules, such as oxygen, carbon dioxide, and steroids, but is impermeable to ions and large, uncharged polar molecules such as glucose. It is also slightly permeable to small, uncharged polar molecules such as water and urea, a waste product from the breakdown of amino acids. The slight permeability to water and urea is an unexpected property since they are polar molecules. These two small molecules are thought to pass through the lipid bilayer in the following way. As the fatty acid tails of membrane phospholipids and glycolipids randomly move about, small gaps briefly appear in the hydrophobic environment of the membrane’s interior. Water and urea molecules are small enough to move from one gap to another until they have crossed the membrane. Simple diffusion Characteristics of simple diffusion -is the only form of transport across cell membranes that is not carrier- mediated. -occurs down an electrochemical gradient ("downhill"). - -does not require metabolic energy and therefore is passive. - Diffusion can be measured using the following equation:. Fick’s law of diffusion J = -PA (C1-C2) J flux (flow) mmol/sec A area cm 2 P permeability cm/sec C1 concentration I mmol/L C2 concentration2 mmol/L Net diffusion of the solute is called flux, or flow (J), and depends on the following variables: size of the concentration gradient, partition coefficient, diffusion coefficient, thickness of the membrane, and surface area available for diffusion. Characteristics of simple diffusion 1-is the only form of transport across cell membranes that is not carrier- mediated. 2-occurs down an electrochemical gradient ("downhill"). - 3-does not require metabolic energy and therefore is passive. -. FACILITATED DIFFUSION Like simple diffusion, facilitated diffusion occurs down an electrochemical potential gradient; thus, it requires no input of metabolic energy. Unlike simple diffusion, however, facilitated diffusion uses a membrane carrier and exhibits all the characteristics of carrier-mediated transport: saturation and competition. Properties of Mediated Transport A substance that is transported by mediated transport is transported much more rapidly than other molecules that are of similar molecular weight and lipid solubility and that cross the membrane by simple diffusion The transport rate shows saturation kinetics. As the concentration of the transported compound is increased, the rate of transport at first increases, but eventually a concentration is reached, above which the transport rate increases no further Whether a substance is being transported actively or passively relates to the following rules: 1. If the direction of the net flux is down an electrochemical gradient, the transport is passive. 2. If the direction of the net flux is up an electrochemical gradient, the transport is active. The two basic forms of active transport—primary and secondary active transport—differ in the nature of the energy source expended. Primary active transport uses ATP or some other chemical energy source directly to transport substances. Proteins involved in primary active transport are called pumps. Secondary active transport is powered by a concentration gradient or an electrochemical gradient that was previously created by primary active transport. Active transport processes have most of the properties of facilitated transport. In addition, active transport systems allow the concentration of their substrates against concentration or electrochemical potential gradients PRIMARY ACTIVE TRANSPORT In active transport, one or more solutes are moved against an electrochemical potential gradient (uphill). In other words, solute is moved from an area of low concentration (or low electrochemical potential) to an area of high concentration (or high electrochemical potential). Na+-K+ ATPase (Na+-K+ Pump) H+ pump ? Ca+2 pump ? the Na-K pump has at least four functions: 1. Regulation of cell volume. 2. Secondary active transport. 3. Heat production. 4. Maintenance of a membrane potential. SECONDARY ACTIVE TRANSPORT Secondary active transport processes are those in which the transport of two or more solutes is coupled. One of the solutes, usually Na+, moves down its electrochemical gradient (downhill), and the other solute moves against its electrochemical gradient (uphill). The downhill movement of Na+ provides energy for the uphill movement of the other solute. There are two types of secondary active transport, distinguishable by the direction of movement of the uphill solute. If the uphill solute moves in the same direction as Na+, it is called cotransport, or symport. If the uphill solute moves in the opposite direction of Na+, it is called countertransport, antiport, or exchange. Cotransport For example, Na+-glucose cotransport and Na+-amino acid cotransport are present in the luminal membranes of the epithelial cells of both small intestine and renal proximal tubule. Another example of cotransport involving the renal tubule is Na+-K+- 2Cl- cotransport, which is present in the luminal membrane of epithelial cells of the thick ascending limb. In each example, the Na+ gradient established by the Na+-K+ ATPase is used to transport solutes such as glucose , amino acids , K+, or Cl- against electrochemical gradients. Give examples for co- transporters present in digestive system and in the kidneys with figures ??? SGLT2 inhibitors are a class of prescription medicines that are FDA-approved for use with diet and exercise to lower blood sugar in adults with type 2 diabetes. Medicines in the SGLT2 inhibitor class include canagliflozin, dapagliflozin, and empagliflozin Countertransport Countertransport (antiport or exchange) is a form of secondary active transport in which solutes move in opposite directions across the cell membrane. Na+ moves into the cell on the carrier down its electrochemical gradient; the solutes that are countertransported or exchanged for Na+ move out of the cell. Osmosis is defined as the flow of water across a semipermeable membrane from a compartment in which the solute concentration is lower to a compartment in which the solute concentration is greater A semipermeable membrane is defined as a membrane permeable to water but impermeable to solutes. Osmosis takes place because the presence of solute decreases the chemical potential of water. Water tends to flow from where its chemical potential is higher to where its chemical potential is lower. Decreasing the chemical potential of water in a solution (because of the presence of solute) also reduces vapor pressure, lowers the freezing point, and increases the boiling point of the solution as compared with pure water Osmolarity The total solute particle concentration of a solution is known as its osmolarity. A solution containing 1 mole of solute particles is said to be at a concentration of 1 osmolar (1 Osm). One mole of solute particles is referred to as 1 osmole. We use the term solute particle to distinguish osmolar from molar because certain solutes when put into solution dissociate into particles, and each solute particle present decreases the concentration of water. For example, 1 liter of a solution containing 0.1 mole of glucose has an osmolarity of 0.1 osmolar because glucose does not dissociate in solution. However, a 1-liter solution containing 0.1 mole of NaCl has an osmolarity of approximately 0.2 osmolar because NaCl dissociates in solution to give approximately 0.1 mole of sodium ions and 0.1 mole of chloride ions, for a total of 0.2 mole of solute particles. In physiology, the terms milliosmole and milliosmolar (mOsm) are frequently used because the solute concentration of body fluids is only a fraction of 1 osmolar. A 1 milliosmolar (1 mOsm) solution contains 1 milliosmole (1/1000 of an osmole) of solute particles per liter. The normal osmolarity of intracellular and extracellular fluid is approximately 300 mOsm (the actual range is 280–296 mOsm), which means that its total solute concentration is 300 milliosmoles per liter. Calculating osmotic pressure (van't Hoff's law) Osmolarity is the concentration of osmotically active particles in a solution. - -can be calculated using the following equation: Osmolarity = g x C osmolarity concentration of particles osmol/L C concentration mol/L g number of particles in solution osmol/mol Ex: g Nacl = 2 g glucose = 1 Osmotic Pressure Osmotic pressure is another term that describes a solution’s total solute concentration; it is often used when comparing other physiological forces described in terms of pressure. The osmotic pressure of a solution (which is indicated by the symbol p) is an indirect measure of its solute concentration and is expressed in ordinary units of pressure, such as atmospheres or millimeters of mercury (mm Hg). As the total solute concentration (osmolarity) increases, the osmotic pressure increases. Therefore, when water moves by osmosis from low solute concentration to high solute concentration, water is also flowing up an osmotic pressure gradient Movement of water up an osmotic pressure gradient. When a semipermeable membrane—which is permeable to water but not to solute—separates two solutions in a chamber, water flows down its concentration gradient toward the side with higher solute concentration. Because osmotic pressure increases as solute concentration increases, water also flows from lower to higher osmotic pressure, or up the osmotic pressure gradient Diffusion of Water Through Aquaporins Although some water crosses the lipid bilayer of cell membranes by simple diffusion, most water diffuses across cell membranes through aquaporins, highly selective pores that permit water—but no solutes—to move across the membrane by diffusion A different situation results if RBCs are placed in a hypotonic solution hypo- ! less than), a solution that has a lower concentration of solutes than the cytosol inside the RBCs. In this case, water molecules enter the cells faster than they leave, causing the RBCs to swell and eventually to burst. The rupture of RBCs in this manner is called hemolysis ( ; hemo- ! blood; -lysis ! to loosen or split apart); the rupture of other types of cells due to placement in a hypotonic solution is referred to simply as lysis. Pure water is very hypotonic and causes rapid hemolysis. A hypertonic solution ; hyper- " greater than) has a higher concentration of solutes than does the cytosol inside RBCs. One example of a hypertonic solution is a 2% NaCl solution. In such a solution, water molecules move out of the cells faster than they enter, causing the cells to shrink. Such shrinkage of cells is called crenation. Vesicular Transport So far, we have considered processes that move one or a few ions or molecules at a time through the plasma membrane. Vesicular transport processes, by contrast, move large particles, droplets of fluid, or numerous molecules at once through the membrane, contained in bubblelike vesicles of membrane. Vesicular processes that bring matter into a cell are called endocytosisand those that release material from a cell are called exocytosis.These processes employ motor proteins whose movements are energized by ATP. There are three forms of endocytosis: phagocytosis, pinocytosis, and receptor-mediated endocytosis. Phagocytosisor “cell eating,” is the process of engulfing particles such as bacteria, dust, and cellular debris— particles large enough to be seen with a microscope. For example, neutrophils (a class of white blood cells) protect the body from infection by phagocytizing and killing bacteria. A neutrophil spends most of its life crawling about in the connective tissues by means of its pseudopods