Lecture 5 Reading: From DNA to Protein PDF
Document Details
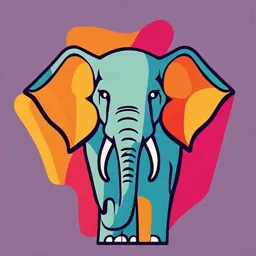
Uploaded by JollyNephrite1296
Tags
Summary
This document is a lecture overview on Introductory Molecular Biology, specifically focusing on the process of transcription, from DNA to RNA. It includes key information about RNA polymerases, transcription in bacteria and eukaryotes, and different types of RNA. It contains diagrams and figures.
Full Transcript
BIOM-2131 Introductory Molecular Biology LECTURE 5 From DNA to RNA LECTURE OVERVIEW ▪ 5.1 RNA ▪ 5.2 RNA polymerases ▪ 5.3 Transcription in bacteria ▪ 5.4 Transcription in eukaryotes ▪ 5.5 Eukaryotic mRNA ...
BIOM-2131 Introductory Molecular Biology LECTURE 5 From DNA to RNA LECTURE OVERVIEW ▪ 5.1 RNA ▪ 5.2 RNA polymerases ▪ 5.3 Transcription in bacteria ▪ 5.4 Transcription in eukaryotes ▪ 5.5 Eukaryotic mRNA DNA → RNA → Protein nucleotide sequence of appropriate segment of DNA is first copied into another type of nucleic acid - RNA resulting RNA copies are used to direct the synthesis of the protein central dogma of molecular biology Alberts, et. al, Essential Cell Biology, 6th edition, Figure 7-1, p. 238 Ribonucleic Acid linear polymer made of four different nucleotide subunits RNA differs from DNA chemically in: ribonucleotides contain sugar ribose base differences contains uracil Alberts, et. al, Essential Cell Biology, 6th edition, Figure 7-3 (partial), p. 239 RNA nucleotides are linked together by: phosphodiester bond complementary base-pairing properties A U Alberts, et. al, Essential Cell Biology, 6th edition, Figure 7-3 (partial) and 7-4, p. 239 RNA differs from DNA…. …. quite dramatically in overall structures DNA occurs always as double-stranded helix RNA is largely single-stranded RNA chain can fold into variety of shapes hypothetical folded RNA structures Alberts, et. al, Essential Cell Biology, 6th edition, Figure 7-5 (partial, modified), p. 240 RNA differs from DNA…. RNA is largely single-stranded RNA chain can fold up into variety of shapes actual RNA molecule involved in RNA splicing RNA can have structural, regulatory, or catalytic roles Alberts, et. al, Essential Cell Biology, 6th edition, Figure 7-5 (partial), p. 240 From DNA to RNA gene a segment of DNA that directs the production of a particular protein or functional RNA molecule From DNA to RNA Transcription the first step in gene expression, the process by which cells read out the instruction in their genes many identical RNA copies can be made from the same gene for protein coding genes, RNA serves solely as an intermediary on protein synthesis path Translation RNA molecule direct synthesis of protein molecules A cell can express different genes at different rates fine-tune the amount of protein produced rate of transcription rate of translation Alberts, et. al, Essential Cell Biology, 6th edition, Figure 7-2, p. 238 Transcription of a gene produces… … an RNA complementary to one strand of DNA nontemplate strand coding strand template strand Alberts, et. al, Essential Cell Biology, 6th edition, Figure 7-6, p. 240 Transcription produces RNA that is complementary to one strand of DNA all the RNA in the cells is made by transcription this process involves: opening and unwinding of a small portion of DNA double helix one of the two DNA strands acts as a template RNA polymerase incorporates complementary ribonucleotides the product is an RNA transcript Transcription produces RNA that is complementary to one strand of DNA transcription differs from DNA replication: the RNA strand does not remain H-bonded to the DNA template strand RNA transcript dissociates and is single-stranded given RNA molecule is copied from only a limited region of DNA most mature RNAs are no more than a few thousand nt long, many much shorter a replicated DNA molecule in a human chromosome - up to 250 million nt DNA is transcribed into RNA by the enzyme RNA polymerase RNA polymerase catalyzes formation of phosphodiester bond moves stepwise along the DNA growing RNA chain is elongated by one ribonucleotide at a 5’-to-3’ direction time Alberts, et. al, Essential Cell Biology, 6th edition, Figure 7-7, p. 241 Many RNA copies can be made from the same gene simultaneously the RNA strand is almost immediately released from the DNA as it is synthesized the synthesis of next RNA is usually started before the first RNA has been completed electron micrograph of two adjacent ribosomal genes (ribosomal rRNA) Alberts, et. al, Essential Cell Biology, 6th edition, Figure 7-8, p. 241 RNA polymerase vs DNA polymerase RNA polymerase uses ribonucleoside triphosphates can start an RNA chain without a primer does not accurately proofread its work makes about 1 mistake in every 10,000nt RNA is not used as the permanent storage form of genetic information minor consequences of mistakes in RNA transcript for a cell Cells produce various types of RNA 1/3 RNA molecules encoded by protein coding genes messenger RNA (mRNA) RNA molecules that ultimately direct synthesis of proteins in eukaryotes mRNA typically carries info transcribed from just one gene codes for a single protein in bacteria set of adjacent genes is often transcribed as a single mRNA codes for several different proteins Cells produce various types of RNA 2/3 the final product of other genes is RNA itself noncoding RNA regulatory, catalytic or structural functions ribosomal RNAs (rRNAs) form the structural and catalytic core of the ribosome transfer RNAs (tRNAs) serve as adaptors between mRNA and aa during protein synthesis microRNAs (miRNAs) regulate gene expression Cells produce various types of RNA 3/3 small interfering RNAs (siRNAs) provide protection from viruses and proliferating transposable elements long noncoding RNAs (lncRNAs) act as scaffolds and serve other diverse functions, many of which are still being discovered other noncoding RNAs used in RNA splicing, gene regulation, telomere maintenance and many other processes Initiation of transcription especially critical process to begin transcription, RNA pol must be able to recognize the start of the gene and bind firmly to the DNA at this site transcription start site of a gene recognition mechanism by RNA polymerase differs between bacteria and eukaryotes Transcription initiation in bacteria RNA polymerase binds tightly to DNA double helix after it recognizes specific nucleotide sequence which lies immediately upstream of the transcription start site, a gene region called a promoter bacterial RNA polymerase contains a subunit called sigma factor – recognizes the promoter Alberts, et. al, Essential Cell Biology, 6th edition, Figure 7-9 (partial), p. 243 Transcription initiation in bacteria RNA polymerase opens up the DNA double helix immediately in front of the promoter one of the two exposed DNA strands then acts as a template for complementary base-pairing with incoming ribonucleosides triphosphates once RNA synthesis begins RNA pol clamps firmly down RNA synthesis continues Alberts, et. al, Essential Cell Biology, 6th edition, Figure 7-9 (partial), p. 243 Transcription in bacteria elongation continues until RNA polymerase reaches second signal in the DNA, the terminator (or stop site) termination and release of both, completed RNA transcript and RNA pol + + Alberts, et. al, Essential Cell Biology, 6th edition, Figure 7-9 (partial), p. 243 Bacterial promoters and terminators have specific sequences importance of initiation of transcription ensures that a segment of DNA will be transcribed only if it is preceded by a promoter nucleotide sequence of a typical bacterial promoter Alberts, et. al, Essential Cell Biology, 6th edition, Figure 7-10 (partial), p. 244 Recognition of promoter sequence by bacterial RNA polymerase sigma () factor is primarily responsible for recognizing the promoter sequence on the bacterial DNA the nucleotide sequence labelled -35 is recognized based on unique features present on the outside of the double helix as the factor begins to open the DNA double helix it can recognize the sequence at the -10 position of the promoter Once the helix has been open by RNA polymerase Which of the two DNA strands should be used as a template for transcription? secret lies in the structure of the promoter itself every promoter has a certain polarity it contains two different nt sequences, laid out in a specific 5’-to-3’ order upstream of the transcription start site these asymmetric sequences position RNA pol that it binds in only one orientation → can only use the DNA strand that is oriented in the 3’-to-5’ direction as a template Direction of transcription on an individual chromosome direction of transcription can vary from one gene to the next Alberts, et. al, Essential Cell Biology, 6th edition, Figure 7-11, p. 244 Bacterial promoters and terminators have specific sequences the nucleotide sequence of a typical terminator Alberts, et. al, Essential Cell Biology, 6th edition, Figure 7-10 (partial), p. 244 Initiation of eukaryotic gene transcription bacterial RNA polymerase relies on a single accessory protein to initiate transcription, sigma factor eukaryotic transcription initiation differs in several important ways from the process in bacteria bacteria use single type of RNA polymerase eukaryotic cells employ three RNA polymerases Eukaryotic gene transcription RNA polymerase I RNA polymerase II RNA polymerase III responsible for transcribing different types of genes Alberts, et. al, Essential Cell Biology, 6th edition, Table 7-2, p. 245 mRNA RNA pol II Initiation of eukaryotic gene transcription eukaryotic RNA polymerases require the assistance of a large set of proteins that are not subunits of the RNA polymerase general transcription factors must assemble at each promoter, along with the RNA polymerase, before transcription can begin Important differences between bacterial and eukaryotic systems bacterial genes tend to lie very close to one another, with very short lengths of nontranscribed DNA between them Important differences between bacterial and eukaryotic systems in eukaryotes individual genes are spread out along the DNA with stretches of up to 100,000nt pairs between one gene and the next this architecture allows a single gene to be controlled by a large variety of regulatory DNA sequences scattered along the DNA more complex form of transcriptional regulation the eukaryotic DNA is packed into nucleosomes and higher order forms of chromatin structure Eukaryotic RNA pol requires general TFs purified RNA pol II cannot initiation transcription alone requires general transcription factors (TFs) accessory proteins that assemble on the promoter position RNA polymerase pull apart the DNA double helix to expose the template strand allowing the RNA pol to begin transcription play functionally equivalent roles to factor Assembly of general transcription factors at a promoter used by RNA pol II TATA box recognized by TATA-box Binding Protein, a subunit of TFIID binding causes dramatic local distortion in the DNA double helix enables binding of TFIIB landmark for subsequent assembly of other proteins on the promoter Alberts, et. al, Essential Cell Biology, 6th edition, Figure 7-13 (partial), p. 246 Transcription initiation complex the rest of the general TFs, and the RNA polymerase assemble at the promoter TFIIH opens the double helix at the transcription start site TFIIH phosphorylates RNA pol II releasing its attachment by the general TF except TFIID TFs dissociate and EFs join in Alberts, et. al, Essential Cell Biology, 6th edition, Figure 7-13 (partial), p. 246 Other proteins involved Elongation factors (EFs) allow the RNA pol II to move through DNA that is packed into nucleosomes Alberts, et. al, Essential Cell Biology, 6th edition, Figure 7-15, p. 247 when RNA pol II finishes transcribing a gene it is released from the DNA tail residue - dephosphorylated by phosphatase Eukaryotic promoters contain sequences Alberts, et. al, Essential Cell Biology, 6th edition, Table 7-14, p. 247 Eukaryotic mRNAs are processed … in the nucleus RNA-processing steps that take place as the RNA is being synthesized capping, splicing, and polyadenylation carried out by enzymes bound to phosphorylated tail of RNA pol II ~ 25 nt Alberts, et. al, Essential Cell Biology, 6th edition, Figure 7-17, p. 248 Eukaryotic mRNA molecules are modified by …. capping and polyadenylation RNA capping polyadenylation modifies the 5’ end special structure of the RNA transcript at the 3’ end series of repeated introduces atypical nt adenines methyl-guanine Alberts, et. al, Essential Cell Biology, 6th edition, Figure 7-18 (partial), p. 249 Capping and polyadenylation of eukaryotic mRNA increase the stability of the mRNA molecule facilitate export from the nucleus to the cytosol mark the RNA molecule as mRNA used by protein-synthesis machinery to ensure that the message is complete before protein synthesis begins In eukaryotes, protein-coding genes are interrupted by noncoding sequences introns range in length from a single nt to > 10,000nt some protein-coding genes lack altogether some have only few most have many Alberts, et. al, Essential Cell Biology, 6th edition, Figure 7-20, p. 250 In eukaryotes, protein-coding genes are interrupted by noncoding sequences exons are usually shorter than introns often represent only a small fraction of the total length of the gene terms “introns” and “exons” apply to both, the DNA and the corresponding RNA sequences Introns are removed from pre-mRNA by RNA splicing splicing happens concurrently with transcription after capping and as the RNA pol II continues to transcribe the gene, RNA splicing begins pre-mRNA (precursor mRNA) in this process, introns are removed from the newly synthesized RNA and exons are stitched together each transcript receives a polyA tail functional mRNA ready to leave nucleus Introns are removed from pre-mRNA by RNA splicing intron contains special short nt sequences that identify it for removal R = A/G Y = C/U N = any nucleotide Alberts, et. al, Essential Cell Biology, 6th edition, Figure 7-21, p. 250 Introns are removed from pre-mRNA by RNA splicing guided by these sequences, an elaborate splicing machine cuts out the intron in the form of a “lariat” structure this structure is produced when an free 3’-OH of adenine nucleotide exon sequence within the intron reacts reacts with the with the beginning of start of next exon the intron + joining the ends together Alberts, et. al, Essential Cell Biology, 6th edition, Figure 7-22, p. 251 RNA splicing by snRNPs splicing is carried out largely by RNA molecules small nuclear RNAs (snRNAs) packed with additional proteins forms small nuclear riboproteins (snRNPs) there are 5 snRNPs and about 200 additional proteins required at each splicing reaction only 3 most important snRNPs will be shown in the upcoming figure; U1, U2 and U6 RNA splicing by snRNPs U1 recognized 5’ splice site U2 recognizes the lariat branch-point site U6 rereads the 5’ splice site by displacing U1 once the splicing rxn have occurred, the spliceosome deposits a group of RNA-binding proteins, called exon junction complex, on the mRNA Alberts, et. al, Essential Cell Biology, 6th edition, Figure 7-23 (partial), p. 251 RNA splicing by snRNPs snRNPs form the core of spliceosome spliceosome large assembly of RNA and protein molecules that carry out splicing in the nucleus assembles on the RNA molecule as it is being synthesized new spliceosome must be assembled for each splicing reaction Intron-exon type of gene arrangement in eukaryotes transcripts of many genes can be spliced in different ways, each of which can produce a distinct protein alternative splicing Alberts, et. al, Essential Cell Biology, 6th edition, Figure 7-25, p. 252 Mature mRNA are exported from the nucleus transport of mRNA from the nucleus to the cytoplasm is highly selective only correctly processed mRNAs are exported export ready mRNA molecule require specific protein partners: cap-binding protein poly-A binding protein exon junction complex export mediated by nuclear pore complex Mature mRNA are exported from the nucleus Alberts, et. al, Essential Cell Biology, 6th edition, Figure 7-27, p. 254 What happens to “other” RNAs in the nucleus? … such as RNA fragments, excised introns, broken RNAs, aberrantly spliced RNAs these are not only useless, but could be potentially dangerous to the cell if allowed to leave the nucleus will be degraded released ribonucleotides are reused for transcription mRNA molecules are eventually degraded in the cytosol single mRNA molecule can be translated into protein many times the length of the time in the cytoplasm greatly influences the amount of protein it produces variable time 10 hrs controlled by nt sequence within 3’ untranslated region (UTR) mRNA will be degraded by ribonuclease (RNase) Eukaryotic and prokaryotic synthesis, processing and degradation of RNA Alberts, et. al, Essential Cell Biology, 6th edition, Figure 7-27, p. 254 Review: From gene to pseudogene Pseudogene Alberts, et. al, Essential Cell Biology, 6th edition, Table 9-2 (partial), p. 333 Lecture 5 Reading Chapter 7: From DNA to Protein: How Cells Read the Genome pages 237-255 Upcoming Lecture 6 Reading Chapter 7: From DNA to Protein: How Cells Read the Genome pages 254-276