Lecture 11: Lipids and Membranes PDF
Document Details
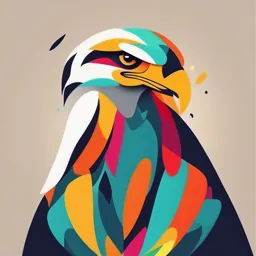
Uploaded by SimplerFoxglove
University of Minnesota
Robert Roon
Tags
Summary
This document provides a detailed overview of lipids and membranes. It covers the types of lipids, their characteristics, and their functions. It also explores the structure of fatty acids and the fluid mosaic model of membrane structure.
Full Transcript
BioC 3021 Notes Robert Roon Lecture 11: Lipids and Membranes Slide 1. Lipids and Membranes Today, we will consider the structure of lipids and membranes. We include membranes along with lipids because membranes contain a high percentage of lipids, and the...
BioC 3021 Notes Robert Roon Lecture 11: Lipids and Membranes Slide 1. Lipids and Membranes Today, we will consider the structure of lipids and membranes. We include membranes along with lipids because membranes contain a high percentage of lipids, and the behavior of membranes is largely defined by their lipid content. Slide 2. Types of Lipids As a class, lipids are more diverse than proteins or carbohydrates. The term lipid defines a diffuse group of compounds whose commonality is the element of non-polarity. While lipids are soluble in non-polar organic solvents, they are sparingly soluble in water. The common lipids listed here include fatty acids, triacylglycerols, phosphoglycerols, sphingolipids, and sterols. This list encompasses compounds that are quite dissimilar in appearance but share a highly hydrophobic chemical nature. Slide 3. Characteristics of Lipids In general, lipids have all or most of the following features: -They are the most highly hydrophobic (non-polar) class of biological compounds. -As a consequence of their hydrophobic nature, lipids can be extracted into non-polar solvents. -Lipids are the most reduced of the biological polymers and can potentially release the most energy per gram upon biological oxidation. -Lipids exhibit limited solubility in water, but often have some amphipathic character—that is, they generally have some regions of polarity to offset their predominantly non-polar character. 1 BioC 3021 Notes Robert Roon Slide 4. Functions of Lipids Lipids serve a number of diverse functions including: -the major energy storage material in humans. A typical human carries 20-25 pounds of lipid. This constitutes enough of an energy reserve to last for a month or two under conditions of starvation. -a critical constituent of biological membranes. In water, phospholipids will spontaneously assemble into bilayers and form closed vesicles. In living organisms, phospholipids constitute the defining component of membrane bilayers. To function properly, mammalian membranes also require a second type of lipid, namely cholesterol. -lipid derivatives (sterols) serve as hormones—compounds that are synthesized in one tissue, travel in the blood to another tissue, and then trigger changes in biological activity. -other lipid derivatives (the bile acids) function as biological detergents, helping to break up large complexes of dietary lipids into smaller micelles. - the phospholipid, sphingomyelin, serves as an insulating material by wrapping around neurons and preventing the leakage of ions from the axons -polyisoprenoid lipids serve as vitamins—compounds that must be obtained in the human diet and function as enzyme cofactors Slide 5. Characteristics of Fatty Acids One key component of many lipids is the fatty acid. Fatty acids are linear hydrocarbons that have one carboxyl group at one end, and many methylene groups in the side chain. They generally have an even number of carbons because they are synthesized and 2 BioC 3021 Notes Robert Roon degraded two carbons at a time. Most fatty acids range from 12 to 24 carbons in length. The hydrophobic character of saturated fatty acids (that have no double bonds) is variable. At the low end, we have the two-carbon compound acetic acid, which is infinitely soluble in water—you can make a single phase system containing any percentage of acetic acid and water. As the number of carbon atoms increases, these compounds become increasingly hydrophobic—their solubility in water decreases dramatically as the effects of the single polar carboxyl group are outweighed by the increasing number of non-polar methylene groups. At the high end, the 20- carbon compound arachidic acid is highly insoluble in water. Slide 6. Naturally Occurring Fatty Acids This table shows that the melting point of saturated fatty acids increases with chain length. Saturated fatty acids with twelve or more carbons are solid at human body temperature, whereas saturated fatty acids in the range of two to ten carbon atoms are liquids. The presence of one double bond (monounsaturated fatty acids) or two or more double bonds (polyunsaturated fatty acids) increases the solubility of the compounds in water and decreases their melting point. All of the common monounsaturated and polyunsaturated fatty acids are liquid at human body temperature. The fluid character of monounsaturated and polyunsaturated fatty acids is critical to the proper function of biological membranes. Slide 7. Fatty Acid Structure The carbon atoms in fatty acids are numbered starting at the carboxyl terminal end of the molecule. Carbons 2 and 3 are often referred to as α and β, respectively. The methyl carbon atom at the distal end of the chain is called the omega (ω) carbon atom. 3 BioC 3021 Notes Robert Roon Slide 8. Space Filling Model of Saturated Fatty Acid Saturated fatty acids with only single carbon-carbon bonds have a zig-zag structure from one carbon atom to another, but overall these molecules are linear in character. Slide 9. Space Filling Model of Unsaturated Fatty Acid In contrast to the linear saturated fatty acids, most naturally occurring unsaturated and polyunsaturated fatty acids have bends or kinks. The double bonds in naturally occurring fatty acids tend to be in the cis configuration. When these cis bonds occur, it causes the fatty acid chain to continue at a new angle, which produces a bent side chain. These non-linear unsaturated and polyunsaturated fatty acids contribute to the liquid nature of membrane bilayers. Slide 10. Hydrogenation of Fatty Acids Commercial hydrogenation of oils containing unsaturated fats increases their solidity. This process is used in the food industry to produce fatty acids that are more useful in food products. The process also makes the fatty acids less healthy, because it increases the percentage of saturated fat. Another unhealthy result of hydrogenation is the production of a reaction side product—trans fatty acids. Trans fatty acids have double bonds in the trans configuration instead of the naturally occurring cis configuration. Mayo Clinic 6/20/11 2:35 PM Comment: Perhaps explain more about why it is unhealthy for the double bonds to be trans instead of Slide 11. Occurrence of Saturated and Unsaturated Fatty cis (trans is harder to break down, if I remember correctly?) Acids in Foods The graph compares the levels of Saturated and Unsaturated Fatty Acids in olive oil, butter, and beef fat. In general, plant oils have much lower levels of saturated fat than are found in animal products such as butter or beef fat. A healthy diet should contain a relatively low amount of total fat. Of that fat, a higher percentage should be unsaturated, and a correspondingly lower percentage should be saturated. A diet low in saturated fat correlates with a 4 BioC 3021 Notes Robert Roon lower occurrence of coronary artery disease. Slide 12. Triacylglycerols Triacylglycerols serve as the major storage lipids in humans. They consist of three fatty acids esterified to glycerol. Triacylglycerols are relatively non-polar molecules, and the component fatty acids tend to be highly reduced (saturated). When unsaturated fatty acids are present, they tend to be linked to the number 2 carbon of glycerol. Slide 13. Occurrence by Triacylglycerols Most dietary and storage lipids occur in the form of triacylglycerols. The dietary triacylglycerols are broken down in the lumen of the intestine. The component fatty acids and monoacylglycerols are taken up into intestinal epithelial cells, where the triacylglycerols are reassembled. They are then released into the lymph and transported in large lipoprotein complexes (chylomicrons) to adipocyte cells. The triacylglycerols are then taken up and stored in adipocytes until there is a need for extra energy. Slide 14. Phosphatidic Acid Phosphoglycerols are related to triacylglycerols; phosphoglycerols are different in that, on the number three carbon, they are connected to a phosphate residue, which is in turn connected to an alcohol derivative. Phosphatidic acid is a precursor to more complex phosphoglycerol compounds. It contains glycerol, two fatty acids, and phosphate, but lacks the alcohol residue. In phosphatidic acid, the two fatty acids are esterified to glycerol at the number 1 and 2 carbons. The fatty acid at position 1 is usually saturated, whereas the fatty acid at position 2 is often unsaturated. The hydroxyl group at position 3 is connected to the phosphate. Slide 15. Phosphoglycerol Structure Phosphoglycerols serve as membrane components. They contain 5 BioC 3021 Notes Robert Roon two fatty acids, glycerol, phosphate, and an ionic or polar alcohol. Similar to phosphatidic acid, the fatty acid at position 2 is often unsaturated, and the fatty acid at position 1 is usually saturated. The phosphoglycerol derivative shown here is phosphatidyl choline (lecithin). The space filling model on the right shows what this compound actually looks like. The model with a spherical polar head and two linear hydrocarbon tails shows the shorthand representation of phospholipids that is often used to represent the phospholipid components of membranes. Slide 16. Phosphatidyl Ethanolamine and Phosphatidyl Choline Two of the most common phosphoglycerol derivatives are phosphatidyl ethanolamine and phosphatidyl choline (also called lecitin). The alcohol derivative in phosphatidyl ethanolamine Mayo Clinic 6/20/11 2:46 PM contains an amine function that is positively charged at Comment: Did you mean lecithin? physiological pH. The alcohol derivative in phosphatidyl choline has a quaternary amine function, which carries a permanent positive charge that is not effected by changes in pH. Slide 17. Phosphatidyl Serine, and Phosphatidyl Inositol Two other common phosphoglycerol derivatives are phosphatidyl serine and phosphatidyl inositol. The alcohol group of phosphatidyl serine is one of the 20 common amino acids, and it carries both a positive charge (on the amino group) and a negative charge (on the carboxylate group) at physiological pH. The alcohol in phosphatidyl inositol is a polyhydroxy compound, which is highly polar but uncharged. Slide 18. Space Filling Model of Phosphatidyl Choline This diagram of phosphatidyl choline shows how the occurrence of a polyunsaturated fatty acid at position 2 can cause the molecule to become kinked. In such structures, the two fatty acid residues do not fit closely together, which increases the liquidity of the 6 BioC 3021 Notes Robert Roon glycerol phosphate derivative in membrane structures. Slide 19. Sphingosine and Sphingomyelin Sphingolipids serve similar membrane functions as phosphoglycerides, but sphingosine replaces glycerol. The sphingosine core provides one hydrocarbon chain, and a long chain fatty acid in amide linkage provides a second hydrocarbon chain. Phosphate and a polar or ionic alcohol make up the remainder of the compound. The sphingomyelin structure shown is very similar to phosphatidyl choline. Although sphingolipids may appear to be different than phosphoglycerols, they actually are quite similar in overall structure, and they also function in a similar capacity in biological membranes. Slide 20. Space Filling Models of Phosphatidyl Choline and Sphingomyelin A comparison of the space filling models of phosphatidyl choline and sphingomyelin reveals that the two structures have very similar dimensions. Slide 21. Membrane Bilayer Containing Saturated Fatty Acids The figure shows a membrane bilayer composed of phospholipids that contain only saturated fatty acid derivatives. Such a membrane would be very compact and solid. It would not function very well in promoting the activity of membrane associated proteins. Slide 22. Membrane Bilayer Containing Unsaturated Fatty Acids In contrast, a membrane containing unsaturated fatty acids esterified in its phospholipids is more diffuse and fluid in character. The occurrence of polyunsaturated fatty acids in membrane phospholipids is a key component of the “fluid mosaic model” of membrane structure. The fluidity of the membrane allows membrane proteins to diffuse in a lateral direction in the plane of 7 BioC 3021 Notes Robert Roon the membrane, which permits various proteins to associate with each other and interact in a functional manner. This highlights the importance of unsaturated fatty acids in promoting the fluid structure of the membrane. Slide 23. Glycolipids Cerebrosides are sphingolipids that have a glucose or galactose unit instead of phosphate and choline. As the name suggests, these compounds are widely distributed in the brain and other nervous tissue. Gangliosides have a similar structure, but the carbohydrate component is more complex and varied. Slide 24. Structure of a Cerebroside Here is the structure of a cerebroside. The molecule is like a phosphoglycerol in that it has two hydrocarbon chains. However, unlike a phosphoglycerol, one of the hydrocarbon chains is built into the core sphingosine unit (shown in blue). The other hydrocarbon unit is provided by a fatty acid (shown in green). The compound also has a single monosaccharide unit, either glucose or galactose (shown in red). Slide 25. Isoprenoid Lipids Polyisoprenoids resemble polymers of isoprene, but have a very different biochemical origin. Vitamin A is a polyisoprenoid compound, as is β-carotene. Slide 26. Cholesterol We have already seen cholesterol in the context of lipoprotein particles, but here it is again for your viewing enjoyment. Cholesterol belongs to the class of sterols, compounds that have a perhydrocyclopentanophenanthrene ring structure. These compounds are not aromatic and not planar, and thus have interesting stereochemistry about the C-C bonds. Cholesterol is a Mayo Clinic 6/20/11 5:29 PM necessary component of mammalian membranes, as it promotes Comment: This adjective is perhaps a little vague 8 BioC 3021 Notes Robert Roon the proper fluidity. It is also a precursor of bile acids and steroid hormones. Slide 27. Bile Salts Bile salts are derived from cholesterol. They act as biological detergents. The bile salts are stored in the gall bladder and released when lipids are being digested. They help to break up large lipid particles into smaller particles. This makes the ingested triacylglycerol compounds more accessible to lipase enzymes. The two examples of bile salts shown here are glycocholate and taurocholate. Slide 28. Steroid Hormones Cholesterol is the parent compound from which a number of steroid hormones are derived. The pathways for hormone synthesis are extensive and complicated. Common steroid hormones derived from cholesterol include progesterone, testosterone, and estradiol. Slide 29. Prostaglandins Prostaglandins are lipids that are synthesized in complex pathways from the polyunsaturated fatty acid arachidonic acid. The arachidonic acid precursor itself is synthesized from the essential polyunsaturated fatty acids linoleic and linolenic acid. Some of the prostaglandins have very nifty oxygen bridges, epoxides, and other structures that are not commonly seen in biological compounds. The prostaglandins have hormone-like actions, and function for short time periods and at short ranges from their site of synthesis. Slide 30. Sensitivity of Prostaglandins to NSAIDs This figure shows how some of the prostaglandins are metabolized. Two of the reactions shown are inhibited by non-steroidal anti- inflammatory drugs (NSAIDs) such as Aspirin or Ibuprofen. Even if you don’t particularly care for prostaglandins, you now know 9 BioC 3021 Notes Robert Roon something about those handy little pills that alleviate your pain. Slide 31. Structures Formed by Detergents and Phospholipids In water, free fatty acids and detergents tend to form monolayers at the air water interface. They can also form micelles, which are spherical monolayer complexes with the polar carboxyl groups facing the water and the nonpolar side chains in the interior. Under the same aqueous conditions, at the air-water interface, phospholipids can form monolayers or vesicles, which are water- filled spherical lipid bilayers. These vesicles have polar phosphate and alcohol components that interact with water on the interior and exterior faces of the vesicle, and nonpolar hydrocarbon chains that form a hydrophobic core in the middle of the bilayer. Slide 32. A Micelle and a Bilayer Here, we see a comparison between micelles and bilayers. Water is excluded from the interior of a micelle, so the interior of the micelle is completely hydrophobic. Bilayers also have a hydrophobic interior, but when they form vesicles, the inside of the vesicle contains water. The phospholipid bilayer vesicles that form in water are similar in character to biological cells. Living cells are surrounded by a plasma membrane bilayer, and their structure is defined by that bilayer. Slide 33. Space Filling Model of a Phospholipid Bilayer The lipid bilayer is composed of a double layer of various phospholipids. Some of the fatty acids in the phospholipids are unsaturated, and they contribute to the fluidity of the bilayer. In the bilayer, the polar phospholipid heads face out toward the surrounding water, and the hydrophobic hydrocarbon chains are in the interior, away from water. Slide 34. Melting Curve for a Phospholipid Bilayer 10 BioC 3021 Notes Robert Roon Membranes have melting points. At temperatures below the melting point, membranes behave as if they were solid, but above the melting temperature, they exhibit a more fluid character. Slide 35. Fluid Mosaic Model In 1972, Singer and Nicolson proposed the fluid mosaic model for membrane structure. This model as currently constituted has the following properties: -The defining feature of membranes is the lipid bilayer composed of phospholipid and glycolipid molecules. -The membrane is a mosaic of nonpolar protein molecules that are embedded in the membrane bilayer. -The lipid bilayer serves as a permeability barrier for polar and ionic molecules, and as a semi-fluid solvent for integral membrane proteins. -Interaction with membrane lipids is necessary for the function of integral membrane proteins. -Membrane lipids and proteins diffuse laterally in the plane of the membrane, but do not rotate (flip-flop) from one side of the membrane to the other. Slide 36. Fluid Mosaic Model for Mammalian Membranes Mammalian membranes include the following common components: - The defining feature of the membrane is a phospholipid bilayer. - Immersed in the non-polar core of the membrane are integral proteins. - Peripheral proteins are found attached two both faces of the bilayer. - There is a significant amount of cholesterol immersed in the non- polar interior of the bilayer. - There are glycolipid and glycoprotein components localized on the outer, extracellular face of the plasma membrane. 11 BioC 3021 Notes Robert Roon Slide 37. Sidedness of Membranes The lack of transverse diffusion of proteins and lipids allows for a sidedness of membrane structure. Membranes have a different phospholipid composition in their inner and outer leaflet. Complex carbohydrates are generally found only on the extracellular face of the plasma membrane, attached to glycoproteins and proteoglycans. Integral proteins have a definite orientation in the membrane. Specific peripheral proteins are attached to either the inner or outer face of the membrane. Slide 38. Diffusion of Membrane Lipids A variety of experiments have demonstrated that both lipids and proteins move relatively rapidly in the plane of the membrane. That is called lateral diffusion. In contrast, transverse diffusion (flip-flop of lipids from one side of the bilayer to the other) occurs very slowly. Once the phospholipids are inserted into a membrane bilayer with a certain orientation, they tend to retain that orientation. Slow transverse diffusion is what permits the membrane phospholipid composition to differ markedly between the two leaflets of the bilayer. Slide 39. Asymmetric Distribution of Membrane Lipids This figure shows that the inner and outer membrane leaflets have very distinct populations of phospholipid. The inner leaflet is rich in phospholipids that have a net negative charge. The outer leaflet has phospholipids that tend to be neutral, with the positive and negative charges canceling each other out. Slide 40. Evidence for Membrane Fluidity Cell fusion experiments demonstrate that proteins diffuse at an appreciable rate in the plane of the membrane. In this experiment, the membrane proteins from human and mouse cells were tagged with distinct fluorescent labels. The two cell populations were 12 BioC 3021 Notes Robert Roon then fused to create hybrid human-mouse heterokaryons. Initially, the two populations of fluorescently labeled proteins were separated, but after a relatively short incubation period, the two populations were totally interspersed. Slide 41. Functions of Membrane Proteins Membrane proteins carry out a wide variety of functions. They serve as enzymes, structural elements, transport systems, ion channels, and as hormone and neurotransmitter receptors. Slide 42. Characteristics of Peripheral and Integral Membrane Proteins -Integral proteins are immersed in the nonpolar interior of the membrane, and have a high percentage of nonpolar amino acids. -Peripheral proteins are not immersed in the interior of the membrane. They are attached to the outer faces of the membrane by various interactions, such as a noncovalent affinity for integral membrane proteins, or a covalent attachment to membrane lipids or carbohydrates. Slide 43. Solubilization of Membrane Proteins -Integral proteins are difficult to remove from the membrane. It is necessary to use detergents to achieve their release. Because they have a highly hydrophobic character, they must continue to be stabilized by detergents after removal from the membrane. In the absence of detergent molecules, integral proteins tend to form gelatinous precipitates. -Peripheral proteins can often be removed from the inner or outer face with mild treatments, such as high salt or enzymatic cleavage of a single chemical bond. They are relatively polar molecules and do not need to be stabilized with detergents after removal from the membrane. Slide 44. Solubilization and Reconstitution of an Integral Membrane Protein 13 BioC 3021 Notes Robert Roon The calcium-ATPase is an integral membrane protein that transports calcium ions across mammalian membranes. Researchers have been able to solubilize this protein using detergents, and to purify it from other contaminating proteins. The investigators were then able to reinsert this protein into an artificial membrane bilayer vesicle system. In these bilayer vesicles, which serve as artificial cells, the transport function of the calcium- ATPase was restored. Because the molecule was able to reconstitute calcium transport using a purified protein, it was unambiguously identified as the calcium transporter. Slide 45. Glycophorin The glycophorin molecule illustrates some common features of integral membrane proteins. Like most integral membrane proteins, glycophorin has a series of hydrophobic amino acids that are immersed in the membrane and are thought to assume an α- helical conformation. Glycophorin exhibits a single membrane spanning α-helix that is highly hydrophobic. The portion of the molecule outside of the cell membrane has mostly hydrophilic amino acids and is water soluble. The green squares and hexagons represent sites where carbohydrate polymers are attached to serine, threonine, and asparagine residues of the protein. The portion of the molecule on the inside of the cell membrane also has hydrophilic amino acids, but it has no carbohydrate attached. The general observation is that membrane associated carbohydrate polymers are attached to the outer leaflet, but not to the inner leaflet. Slide 46. Hydropathy Plot for Glycophorin Analysis of the hydropathy index of glycophorin allows researchers to predict the occurrence of its hydrophobic, membrane spanning α-helix structures. Areas of extensive runs of nonpolar amino acids exhibit positive values on this index, and they suggest the presence of a membrane spanning region in the protein. (Conventional X-ray analysis is difficult or impossible with 14 BioC 3021 Notes Robert Roon hydrophobic membrane proteins, because they generally cannot be readily crystallized. For that reason, data from hydropathy plots are used to construct models for membrane proteins.) The hydropathy plot that is shown here was constructed by averaging the hydropathy indices for ten amino acids at a time, starting at the amino terminal end of the protein, and moving over one amino acid residue at a time. The average hydropathy index for each ten amino acid set was then plotted against the first amino acid residue in the ten amino acid window. The data clearly indicate that there is one membrane spanning a-helix in glycophorin. Slide 47. Transport Mechanisms We will now turn our attention to transport mechanisms—the systems that are used to move molecules through membranes. It turns out that most molecules cannot penetrate a membrane without the intervention of a specific protein transport system. Large Molecules, such as proteins and lipids, move into cells using specific endocytosis mechanisms. Endocytosis involves the mass transport of many molecules (or even whole viruses or bacteria) using a vesicular system. During endocytosis, the cell membrane surrounds the substrates and then invaginates to form an intracellular vesicle. Large molecules move out of cells using specific secretory mechanisms. For example, extracellular proteins contain an N- terminal amino acid sequence that is recognized by protein secretion complexes in the cell membrane. The secretory system insures that the labeled protein ends up outside of the cell. Small Molecules such as sugars and amino acids use a variety of mechanisms to cross the cell membrane. Simple diffusion can occur with small non-polar molecules, such as oxygen or ether. With simple diffusion, the substrate moves directly through a membrane with neither a protein tunnel nor energy coupling. With 15 BioC 3021 Notes Robert Roon facilitated diffusion, the substrate will move through a tunnel, but no energy coupling is involved. With active transport, the substrate moves though a tunnel, and that movement is coupled to the expenditure of energy. Slide 48. Membranes are Impermeable to Most Metabolites Small, relatively non-polar molecules such as carbon dioxide, oxygen, nitrogen, urea, and ethanol can cross a membrane using simple diffusion. Such molecules pass directly though the membrane lipid bilayer. Most other molecules can only cross the membrane using a specific transport system, ion channel, or pore protein. These larger and/or more polar molecules include sugars, amino acids, ions, nucleotides, and even water. Slide 49. Features of Simple Diffusion and Facilitated Diffusion Simple diffusion has a number of distinguishing characteristics. The substrate moves directly through the membrane lipids with no involvement of a protein tunnel. Because there is no energy coupling, the flow of substrate is bidirectional, with net movement in the direction of the concentration gradient—from high concentration to low concentration. Because there is no protein involved, the process is not saturable and not sensitive to inhibitors. Facilitated diffusion involves the movement of a substrate through a protein tunnel. There is no energy coupling with facilitated diffusion. Again, the flow of the substrate is bidirectional, with net flow being in the direction of the concentration gradient, from high concentration to low. Because there is a protein involved, and the system has a selective binding site, the process is saturable and sensitive to inhibitors. Slide 50. A Model for Facilitated Diffusion. The glucose transporter serves as a model for facilitated transport. This transporter has a binding site for glucose, which can be oriented either outward or inward. Glucose on the outside of the 16 BioC 3021 Notes Robert Roon cell can bind to the outward facing binding site. Reorientation of the bound glucose allows it to diffuse through the transporter tunnel to the interior of the cell. The unoccupied binding site can then reorient toward the outside of the cell and accept another glucose molecule. Because this system is not coupled to energy, the net flow of glucose is always from high concentration to low concentration. Slide 51. Kinetics of Simple and Facilitated Diffusion The figure shows that a plot of velocity vs substrate concentration for simple diffusion is slow and linear. This happens because, with simple diffusion, there are no specific binding sites to be filled. Thus, the rate of transport is directly proportional to the substrate level over a wide range of substrate concentrations. The plot for facilitated diffusion (mediated transport) is faster and hyperbolic—similar to the V vs S plot for an enzyme. Facilitated diffusions systems are saturable because there are a limited number of transport proteins involved. Once all of the binding sites on the transport proteins are filled, the rates of transport do not increase with increasing substrate. Slide 52. Specificity of the Glucose Transporter The data show that Km values for glucose transport differ for various substrates. The Km for the favored substrate, D-glucose, is 1.5 mM, whereas for L-glucose, the Km is above 3000 mM. Transport proteins have binding sites that can discriminate between the stereochemical features of their potential substrates. Slide 53. Features of Active Transport Systems Active transport systems are coupled to energy sources, such as ATP or ion gradients. -Primary Active Transport systems are directly coupled to ATP hydrolysis. Because of this, transport can occur against a concentration gradient—from areas of low substrate concentration 17 BioC 3021 Notes Robert Roon towards areas of high substrate concentration. Since going against a substrate’s concentration gradient is a thermodynamically uphill process, active transport systems require the direct coupling to an energy source. The involvement of a protein with a distinct binding site means that active transport systems are saturable and can be inhibited. -Secondary Active transport is energized by ion gradients— typically by sodium or potassium ions. These gradients must first be established by a primary active transport system. Thus, secondary active transport is indirectly coupled to ATP hydrolysis. Because energy is involved, secondary active transport can accomplish the concentrative uptake of a substrate. The involvement of a protein with a distinct binding site means that secondary active transport systems are saturable and can be inhibited. Slide 54. Examples of Primary Active Transport Systems The substrates for primary, ATP driven transport systems include sodium, potassium, calcium, and hydrogen ions. Although primary active transport systems are few in number, they account for a large percentage of the energy expenditure in humans. In fact, the sodium, potassium ATPase utilizes more energy than any other physiological process. Slide 55. The Sodium Potassium ATPase The figure illustrates the mechanism of the sodium, potassium ATPase, a primary active transport protein. Like other primary active transport proteins, this ATPase is energized by the direct hydrolysis of ATP inside the cell. The transport system is oriented so that, for each ATP used, it pumps three sodium ions out of the cell and two potassium ions into it. The ATP cofactor must be on the inside of the cell in order to bind to the active site of the ATPase protein and be hydrolyzed. Because three positive sodium ions move out of the cell and only two positive potassium ions 18 BioC 3021 Notes Robert Roon move into the cell, the system helps maintain the inside negative membrane potential. Slide 56. Secondary Active Transport Systems In place of ATP hydrolysis, secondary active transporter systems use a gradient of one ion or compound to power the concentrative transport of a second ion or compound. The downhill flow of one substrate from an area of high concentration to low concentration energizes the uphill flow of another substrate from an area of low concentration to high. -Antiporters couple the downhill flow of one species (usually an ion) to energize the uphill flow of another in the opposite direction across the membrane. -Symporters couple the downhill flow of one species (again, usually an ion) to energize the uphill flow of another in the same direction across the membrane. -Uniporters have only one substrate being transported. These uniporter systems have always confused me, when they are listed as a type of secondary transport system. The only way that I can envision them being energized, is if the substrate is charged and crosses a cell membrane that has an inside negative membrane potential. If a negatively charged substrate leaves the cell, it could be pushed away from the inside negative membrane potential. A positively charged substrate could be brought into the cell by its attraction to the inside negative membrane potential. Maybe??? Slide 57. A Model for Secondary Active Transport Lactose permease is a symporter that uses a hydrogen ion gradient to drive the uptake of lactose and other sugars. Lactose permease has the following mechanism: -the permease binds a proton from the outside of the cell -the sugar substrate then binds, also from the outside 19 BioC 3021 Notes Robert Roon -the permease everts (changes conformation so that the substrates are reoriented toward the inside of the cell) -the sugar substrate is released inside the cell -the proton is released inside the cell -the permease changes conformation so that its binding sites are once more available to bind a proton and a sugar on the outside. Again, do not take the model shown here too seriously. The overall kinetics may be correct, but the picture of a large segment of the protein rocking back and forth is almost certainly incorrect. Biophysical studies of membrane transport proteins do not show such massive movements of entire proteins. A more correct model might show a protein tunnel, with a small section in the tunnel undergoing small transitions. 20