Introduction to Neuroimaging - Transcranial Magnetic Stimulation (TMS) & Transcranial Electric Stimulation (TES) PDF
Document Details
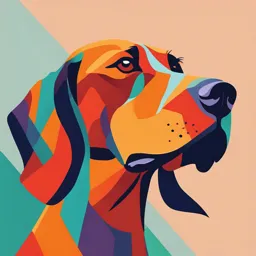
Uploaded by SpellboundLove
Ghent University
2023
Nicoleta Prutean
Tags
Summary
This document is a presentation about Transcranial Magnetic Stimulation (TMS) and Transcranial Electric Stimulation (TES). It explains the basics, methods, experimental designs, clinical applications, and pros and cons of these non-invasive brain stimulation techniques. The presentation is from Ghent University in October 2023.
Full Transcript
DEPARTMENT OF EXPERIMENTAL PSYCHOLOGY NOTEBAERT LAB/EFFORT GROUP INTRODUCTION TO NEUROIMAGING – TRANSCRANIAL MAGNETIC STIMULATION (TMS) & TRANSCRANIAL ELECTRIC STIMULATION (TES) NICOLETA PRUTEAN – October 20th 2023 Slide credits: Dr. David Wisniewski Prof. Antonino Vallesi OVERVIEW TMS 1. TMS B...
DEPARTMENT OF EXPERIMENTAL PSYCHOLOGY NOTEBAERT LAB/EFFORT GROUP INTRODUCTION TO NEUROIMAGING – TRANSCRANIAL MAGNETIC STIMULATION (TMS) & TRANSCRANIAL ELECTRIC STIMULATION (TES) NICOLETA PRUTEAN – October 20th 2023 Slide credits: Dr. David Wisniewski Prof. Antonino Vallesi OVERVIEW TMS 1. TMS BASICS 2. • • • • TMS METHODS Location Coils Parameters Neural state 3. EXPERIMENTAL DESIGNS • MEPs • Phosphenes • fMRI-guided TMS 4. CLINICAL APPLICATIONS 5. SUMMARY PROS AND CONS 3 OVERVIEW TMS 1. TMS BASICS 2. • • • • TMS METHODS Location Coils Parameters Neural state 3. EXPERIMENTAL DESIGNS • MEPs • Phosphenes • fMRI-guided TMS 4. CLINICAL APPLICATIONS 5. SUMMARY PROS AND CONS 4 WHAT IS TMS? ̶ A non-invasive technique for neural interference and neuromodulation: it allows to temporarily change the functioning of cortical regions, and the related cognitive functions ̶ It is used for research and clinical applications 5 VERY SHORT HISTORY Faraday, 1831: electromagnetic induction D’Arsonval , 1896: magneto-phosphene Barker, Jalinous & Freeston, 1985: modern TMS 6 HOW DOES IT WORK? Faraday-Neumann-Lenz law of electromagnetism: a current flux of a given intensity (I) in a conductor creates a magnetic field (B) around the conductor itself Rule of right hand e.g., circular conductors 7 HOW DOES IT WORK? Faraday-Neumann-Lenz law of electromagnetism: a current flux of a given intensity (I) in a conductor creates a magnetic field (B) around the conductor itself A current up to 8000 Ampere is abruptly generated and passed to a coil. In 200 µs the current produces a magnetic pulse which lasts < 1 ms and is up to 2 Tesla strong. This abrupt change in the magnetic field generates an electric field in a conductor placed close to the coil (e.g., head) , which interferes with ongoing activity à membrane depolarization/hyperpolarization depending on the orientation of neurons. 8 WHY DO WE USE IT? To establish a causal relationship between brain and cognitive functions: interference (stimulation/inhibition) in a cortical area à change in behavior ≠ neuroimaging technique Cfr. Lesion studies (serendipity and spatial resolution) and cortical stimulation (invasiveness and feasibility) Walsh & Cowey, 2000 9 OVERVIEW TMS 1. TMS BASICS 2. • • • • TMS METHODS Location Coils Parameters Neural state 3. EXPERIMENTAL DESIGNS • MEPs • Phosphenes • fMRI-guided TMS 4. CLINICAL APPLICATIONS 5. SUMMARY PROS AND CONS 10 LOCATION Constraint: stimulation depth of 2 cm - mainly cortical surface (gyri) - limited in sulci - possible in subcortical areas only with non-standard and expensive coils (e.g., H-coil by BrainsWay) Localization: - Anatomical landmarks e.g., V5 3 cm up, 5 cm right from inion - 10-20 international EEG system e.g., Broca area around F3 https://www.youtube.com/watch?v=t31wOK7eVDA - (Neuroimaging) + neuronavigation 11 BEYOND LOCATION E.g., sensorimotor areas Primary effects: - Motor cortex = measure motor-evoked potentials (MEPs) https://www.youtube.com/watch?v=ALkWlMem1aw - Visual cortex = induce phosphenes, transient scotomas, block motion processing Secondary effects: - Interference in neighbouring regions - Interference in directly connected regions Felleman&Van Essen, 1991 12 COILS Shape of coil determines focality and depth of stimulation E.g., circular vs figure-8 coil Available at Ugent PP02 13 COILS Position of coil determines location of stimulation E.g., find FDI representation in motor homunculus 14 COILS Position of coil determines location of stimulation Orientation of coil (rotation and tilting) determines location and focality E.g., find FDI representation in motor homunculus Opitz et al., 2013 15 PARAMETERS: INTENSITY Intensity of stimulation depends on strenght of electric current of TMS machine (maximum stimulator output, MSO%) It’s a percentage of a subject-by-subject thereshold: - Motor threshold: lowest intensity (M1) needed to evoke MEPs in 50% of trials Differs for e.g., healthy vs clinical populations (MS, stroke) - Phosphene threshold: lowest intensity (V1,V5) needed to evoke phosphenes in 50% trials Differs for e.g., healthy vs clinical populations (migraine) NB: Above threshold = generates action potential Below threshold = modulates resting potential 16 PARAMETERS: FREQUENCY Single pulse e.g., M1 (MEPs), V1 (phosphenes) Paired pulse (2 pulses from same coil after time interval) e.g., intracortical inhibition/facilitation depending on interstimulus interval Short ISI (1-4 ms) à inhibition; Long ISI (7-20 ms) à facilitation Double pulse (2 pulses from 2 coils) for functional connectivity Repetitive TMS (1-20+ Hz) <= 1Hz à inihbitory 5-20Hz à excitatory Special protocols e.g., theta burst 17 PARAMETERS: TIMING Only online: 100 ms aftereffects Online & offline: aftereffects up to 7090 minutes. Longer effects are hardly ethical in research, but highly desirable in clinical applications. 18 NEURAL STATE TMS induces neural noise which interferes and interacts with ongoing neural processing à statedependent effects Stochastic resonance: addition of noise can push weak subthreshold signals across threshold, improving processing; if noise level is too high processing can be disrupted (Miniussi et al., 2013). 19 NEURAL STATE TMS induces neural noise which interferes and interacts with ongoing neural processing à statedependent effects Stochastic resonance: addition of noise can push weak subthreshold signals across threshold, improving processing; if noise level is too high processing can be disrupted (Miniussi et al., 2013). Silvanto et al., 2008 20 OVERVIEW TMS 1. TMS BASICS 2. • • • • TMS METHODS Location Coils Parameters Neural state 3. EXPERIMENTAL DESIGNS • MEPs • Phosphenes • fMRI-guided TMS 4. CLINICAL APPLICATIONS 5. SUMMARY PROS AND CONS 21 EXPERIMENTAL DESIGNS: MEPS TMS over left M1 (representing right FDI) during a cue-to-target interval à MEP measured with EMG MEP amplitude quantifies corticospinal excitability (CSE) CSE suppression = index of motor preparation Increseased motor preparation for rewarded trials Bundt et al., 2016 22 EXPERIMENTAL DESIGNS: PHOSPHENES Measure phosphene threshold Subthreshold TMS (left/right) over V1 à light flashes (right/left) Task: report if flash is on left/right Subthreshold auditory stimuli are also presented left/right Incresead V1 excitability reveals multi-sensory integration 23 EXPERIMENTAL DESIGNS: FMRI-GUIDED TMS fMRI study to find within-subjects peak acivity during subtractions/multiplications Neuronavigation to position TMS over hIPS/PSPL Virtual lesion approach Nb: sham condition Causal role of hIPS in numerical cognition 24 OVERVIEW TMS 1. TMS BASICS 2. • • • • TMS METHODS Location Coils Parameters Neural state 3. EXPERIMENTAL DESIGNS • MEPs • Phosphenes • fMRI-guided TMS 4. CLINICAL APPLICATIONS 5. SUMMARY PROS AND CONS 25 CLINICAL APPLICATIONS: THERAPEUTIC USE Stroke • Aphasia • Motor disability Tinnitus Anxiety disorders • Panic disorder • OCD Amyotrophic lateral sclerosis Multiple sclerosis Epilepsy Movement disorders • Parkinson’s, Huntington’s • Dystonia Schizophrenia • Schizoaffective disorder • Auditory hallucinations Addiction Major depressive disorder Migraines Epilepsy Hemispatial neglect Increase inhibition in M1 Slow rTMS (1Hz) Improvement in motor coordination 26 CLINICAL APPLICATIONS: THERAPEUTIC USE Stroke • Aphasia • Motor disability Tinnitus Anxiety disorders • Panic disorder • OCD Amyotrophic lateral sclerosis Multiple sclerosis Epilepsy Movement disorders • Parkinson’s, Huntington’s • Dystonia Schizophrenia • Schizoaffective disorder • Auditory hallucinations Addiction Major depressive disorder Migraines Epilepsy Hemispatial neglect “Voices” may be due to overactivation of temporal regions (involved with speech). Increase inhibition in temporal regions Slow rTMS (1Hz) Sustained reductions activity 27 CLINICAL APPLICATIONS: THERAPEUTIC USE Stroke • Aphasia • Motor disability Tinnitus Anxiety disorders • Panic disorder • OCD Amyotrophic lateral sclerosis Multiple sclerosis Epilepsy Movement disorders • Parkinson’s, Huntington’s • Dystonia Schizophrenia • Schizoaffective disorder • Auditory hallucinations Addiction Major depressive disorder Migraines Epilepsy Hemispatial neglect Rapid rTMS (>1Hz) over affected hemisphere Enhanced excitability. Increases in MEPs and improved finger motor task. 28 CLINICAL APPLICATIONS:THERAPEUTIC USE Stroke • Aphasia • Motor disability Tinnitus Anxiety disorders • Panic disorder • OCD Amyotrophic lateral sclerosis Multiple sclerosis Epilepsy Movement disorders • Parkinson’s, Huntington’s • Dystonia Schizophrenia • Schizoaffective disorder • Auditory hallucinations Addiction Major depressive disorder Migraines Epilepsy Hemispatial neglect In treatment-resistant patients: - High frequency rTMS of the left DLPFC (hypoactivity); - Low frequency rTMS of the right DLPFC (hyperactivity). 29 CLINICAL APPLICATIONS: MECHANISMS NB: control condition (group/sham stimulation) Long-term effects (synaptic plasticity) could be due to: - direct effects: cortical excitability of dysfunctional area are altered - indirect effects: cortical excitability of up/downstream areas are altered Long-term ≠ permanent 30 SUMMARY: TMS PROS AND CONS PROS: CONS: - Non-invasive - Allows causal inference - Great temporal (ms) and good spatial resolution (depending on parameters) - No long-term side effects - Flexibility in choosing within/between ss designs - Clinical applications - Shallow stimulation (2 cm depth) - Need for good control condition (group/site/sham) - Exclusion criteria for safety reasons - Can be painful/annoying (muscular twiches) 31 OVERVIEW TES 1. tDCS BASICS 2. tDCS CLINICAL USE 3. tDCS EXPERIMENTAL EXAMPLE 4. tDCS CONTROVERSIES 5. tACS EXPERIMENTAL EXAMPLE 6. SUMMARY PROS AND CONS 32 OVERVIEW TES 1. tDCS BASICS 2. tDCS CLINICAL USE 3. tDCS EXPERIMENTAL EXAMPLE 4. tDCS CONTROVERSIES 5. tACS EXPERIMENTAL EXAMPLE 6. SUMMARY PROS AND CONS 33 TDCS: VERY SHORT HISTORY Galvani vs Volta (~1781): electric conduction in animal cells Aldini: ”tDCS” ameliorates melancholia Priori et al., 1998, Nitsche et al., 2003: modern TES Stimulator delivering current 2 active electrodes 34 TDCS: PRINCIPLES A constant electrical current (between 1-2 mA) flows from a positive pole (anode) to a negative pole (cathode) Constant current from stimulator Positive ions from anode to cathode, Negative ions from cathode to anode 35 TDCS: PRINCIPLES Polarity dependent modulation: Anodal (+) tDCS Increases cortical excitability (nb: no action potentials!) Depolarizes resting membrane potential à more spontaneous neuronal firing Cathodal (-) tDCS Decreases cortical excitability Hyperpolarizes resting membrane à less spontaneous neuronal firing 36 TDCS: PRINCIPLES Modulatory evidence from MEPs Pizzolato et al., 2013 Nitsche and Paulus 2000-2001 37 TDCS: MONTAGE 38 TDCS: TIMING ‒ Offline (before task) ‒ Online (during) ‒ Both Dockery et al., 2009 39 RELATED METHODS Current is constantly delivered Current is alternating at a specific frequency Oscillatory current is coupled with a direct current (positive/negative) 40 OVERVIEW TES 1. tDCS BASICS 2. tDCS CLINICAL USE 3. tDCS EXPERIMENTAL EXAMPLE 4. tDCS CONTROVERSIES 5. tACS EXPERIMENTAL EXAMPLE 6. SUMMARY PROS AND CONS 41 TDCS: CLINICAL USE 42 OVERVIEW TES 1. tDCS BASICS 2. tDCS CLINICAL USE 3. tDCS EXPERIMENTAL EXAMPLE 4. tDCS CONTROVERSIES 5. tACS EXPERIMENTAL EXAMPLE 6. SUMMARY PROS AND CONS 43 TDCS: EXPERIMENTAL EXAMPLE Does anodal tDCS enhance performance in a sequential-letter working memory task? Anodal tDCS over DLPFC (associated with WM) Fregni et al., 2009 44 TDCS: EXPERIMENTAL EXAMPLE Does anodal tDCS enhance performance in a sequential-letter working memory task? Fregni et al., 2009 45 TDCS: EXPERIMENTAL EXAMPLE Does anodal tDCS enhance performance in a sequential-letter working memory task? Fregni et al., 2009 46 TDCS: EXPERIMENTAL EXAMPLE Does anodal tDCS enhance performance in a sequential-letter working memory task? Fregni et al., 2009 47 OVERVIEW TES 1. tDCS BASICS 2. tDCS CLINICAL USE 3. tDCS EXPERIMENTAL EXAMPLE 4. tDCS CONTROVERSIES 5. tACS EXPERIMENTAL EXAMPLE 6. SUMMARY PROS AND CONS 48 TDCS: CONTROVERSIES 49 OVERVIEW TES 1. tDCS BASICS 2. tDCS CLINICAL USE 3. tDCS EXPERIMENTAL EXAMPLE 4. tDCS CONTROVERSIES 5. tACS EXPERIMENTAL EXAMPLE 6. SUMMARY PROS AND CONS 50 TACS: EXPERIMENTAL EXAMPLE Can we use tACS to manipulate rhythmic brain activity? Fiene et al., 2020 51 TACS: EXPERIMENTAL EXAMPLE Rhythmic brain activity important for stimulus processing Participants watch a flickering light Visual cortex synchronizes with flickering frequency Fiene et al., 2020 52 TACS: EXPERIMENTAL EXAMPLE Manipulate synchrony between flicker and tACS More synchronous = stronger neural response Fiene et al., 2020 53 OVERVIEW TES 1. tDCS BASICS 2. tDCS CLINICAL USE 3. tDCS EXPERIMENTAL EXAMPLE 4. tDCS CONTROVERSIES 5. tACS EXPERIMENTAL EXAMPLE 6. SUMMARY PROS AND CONS 54 SUMMARY PROS AND CONS PROS: CONS: - Easy to apply - Cheap - Easy sham condition - Less ‘adverse’ effects - Low temporal resolution - Low spatial resolution - Questionable efficacy in healthy population - Exclusion criteria 55