Harper's Biochemistry Chapter 27 - Biosynthesis of the Nutritionally Nonessential Amino Acids PDF
Document Details
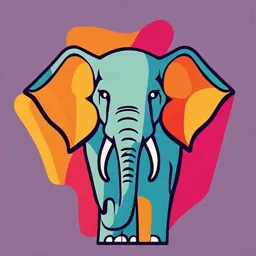
Uploaded by PrizeMeerkat
Victor W. Rodwell, PhD
Tags
Summary
This textbook chapter details the biosynthesis of nutritionally nonessential amino acids. It explores the vital roles of these amino acids in various metabolic pathways and examines their importance for human health.
Full Transcript
S E C T I O N Metabolism of Proteins & VI Amino Acids Biosynthesis of the C H A P T E R Nutritionally...
S E C T I O N Metabolism of Proteins & VI Amino Acids Biosynthesis of the C H A P T E R Nutritionally Nonessential Amino Acids Victor W. Rodwell, PhD 27 O B J E C TI V E S Explain why the absence of certain amino acids that are present in most proteins from the human diet typically is not deleterious to human health. After studying this chapter, Appreciate the distinction between the terms “essential” and “nutritionally you should be able to: essential” amino acids, and identify the amino acids that are nutritionally nonessential. Name the intermediates of the citric acid cycle and of glycolysis that are precursors of aspartate, asparagine, glutamate, glutamine, glycine, and serine. Illustrate the key role of transaminases in amino acid metabolism. Explain the process by which the 4-hydroxyproline and 5-hydroxylysine of proteins such as collagen are formed. Describe the clinical presentation of scurvy, and provide a biochemical explanation for why a severe deprivation of vitamin C (ascorbic acid) results in this nutritional disorder. Appreciate that, despite the toxicity of selenium, selenocysteine is an essential component of several mammalian proteins. Define and outline the reaction catalyzed by a mixed-function oxidase. Identify the role of tetrahydrobiopterin in tyrosine biosynthesis. Indicate the role of a modified transfer RNA (tRNA) in the cotranslational insertion of selenocysteine into proteins. BIOMEDICAL IMPORTANCE significant nutritiona and metaboic abnormaities. Both the nutritiona disorder scurvy, a dietary deficiency of vitamin C, Amino acid deficiency states can resut if nutritionay essentia and specific genetic disorders are associated with an impaired amino acids are absent from the diet, or are present in inade- abiity of connective tissue to form peptidy 4-hydroxyproine quate amounts. In certain regions of West Africa, for exampe, and peptidy 5-hydroxyysine. The resuting conformationa kwashiorkor sometimes resuts when a chid is weaned onto instabiity of coagen is accompanied by beeding gums, a starchy diet poor in protein, whie marasmus may occur sweing joints, poor wound heaing, and utimatey in death. if both caoric intake and specific amino acids are deficient. Menkes syndrome, characterized by kinky hair and growth Patients with short bowe syndrome, if unabe to absorb suf- retardation, resuts from a dietary deficiency of copper, an ficient quantities of caories and nutrients, may suffer from essentia cofactor for the enzyme ysy oxidase that functions 273 274 SECTION VI Metabolism of Proteins & Amino Acids in formation of the covaent cross-inks that strengthen coa- Biosynthesis of the Nutritionally gen fibers. Genetic disorders of coagen biosynthesis incude severa forms of osteogenesis imperfecta, characterized by Essential Amino Acids Involves fragie bones, and Ehlers-Danlos syndrome, a group of con- Lengthy Metabolic Pathways nective tissue disorders that resut in mobie joints and skin The existence of nutritiona requirements suggests that depen- abnormaities due to defects in the genes that encode enzymes, dence on an external source of a specific nutrient can be of incuding procoagen-ysine 5-hydroxyase. greater surviva vaue than the abiity to biosynthesize it. Why? Because if the diet contains ampe quantities of a nutrient, retention of the abiity to biosynthesize it represents informa- NUTRITIONALLY ESSENTIAL & tion of negative surviva vaue, because ATP and nutrients are NUTRITIONALLY NONESSENTIAL not required to synthesize “unnecessary” DNA—even if spe- AMINO ACIDS cific encoded genes are no onger expressed. The number of enzymes required by prokaryotic ces to biosynthesize the Whie often empoyed with reference to amino acids, the terms nutritionay essential amino acids is arge reative to the num- “essentia” and “nonessentia” are miseading, since for human ber of enzymes required for the formation of the nutritionay subjects a 20 common amino acids are essentia to ensure nonessential amino acids (Table 27–2). This suggests a sur- heath. Of these 20 amino acids, 8 must be present in the human viva advantage for humans to retain the abiity to biosynthe- diet, and thus are best termed “nutritionally essential.” The other tize “easy” amino acids whie osing the abiity to make others 12 “nutritionally nonessential” amino acids, whie metabolically more difficut to biosynthesize. The reactions by which organ- essentia, need not be present in the diet (Table 27–1). The dis- isms such as pants and bacteria, but not human subjects, form tinction between these two casses of amino acids was estabished certain amino acids are not discussed. This chapter addresses in the 1930s by feeding human subjects a diet in which purified the reactions and intermediates invoved in the biosynthesis of amino acids repaced protein. Subsequent biochemica investiga- the 12 nutritionay nonessential amino acids in human tissues tions utimatey reveaed the reactions and intermediates invoved and emphasizes seected medicay significant disorders asso- in the biosynthesis of a 20 amino acids. Amino acid deficiency ciated with their metaboism. disorders are endemic in certain regions of West Africa where diets rey heaviy on grains that are poor sources of tryptophan and ysine. These nutritiona disorders incude kwashiorkor, TABLE 27–2 Enzymes Required for the Synthesis of which resuts when a chid is weaned onto a starchy diet poor in Amino Acids From Amphibolic Intermediates protein, and marasmus, in which both caoric intake and spe- cific amino acids are deficient. Number of Enzymes Required to Synthesize Nutritionally Essential Nutritionally Nonessential Arga 7 Ala 1 TABLE 27–1 Amino Acid Requirements of Humans His 6 Asp 1 Nutritionally Essential Nutritionally Nonessential Thr 6 Asnb 1 Argininea Alanine Met 5 (4 shared) Glu 1 Histidine Asparagine Lys 8 Glna 1 Isoleucine Aspartate Ile 8 (6 shared) Hylc 1 Leucine Cysteine Val 6 (all shared) Hypd 1 Lysine Glutamate Leu 7 (5 shared) Proa 3 Methionine Glutamine Phe 10 Ser 3 Phenylalanine Glycine Trp 5 (8 shared) Glye 1 Threonine Hydroxyprolineb 59 (total) Cysf 2 Tryptophan Hydroxylysineb Tyrg 1 Valine Proline 17 (total) Serine a From Glu. Tyrosine b From Asp. c From Lys. a d Nutritionally “semiessential.” Synthesized at rates inadequate to support growth of From Pro. e children. From Ser. b f Not necessary for protein synthesis, but is formed during posttranslational process- From Ser plus sulfate. g ing of collagen. From Phe. CHAPTER 27 Biosynthesis of the Nutritionally Nonessential Amino Acids 275 + NH3 – O3PO O– O O FIGURE 27–3 γ-Glutamyl phosphate. Glutamate Dehydrogenase, Glutamine FIGURE 27–1 The reaction catalyzed by glutamate dehydro- Synthetase, & Aminotransferases genase (EC 1.4.1.3). Play Central Roles in Amino Acid Biosynthesis The combined action of the enzymes gutamate dehydrogenase, gutamine synthetase, and the aminotransferases (see Figures 27–1, BIOSYNTHESIS OF THE 27–2, and 27–4) resuts in the incorporation of potentiay cyto- NUTRITIONALLY NONESSENTIAL toxic ammonium ion into nontoxic amino acids. AMINO ACIDS Asparagine Glutamate The conversion of aspartate to asparagine, catayzed by aspara- Gutamate, the precursor of the so-caed “gutamate famiy” of gine synthetase (Figure 27–5), resembes the gutamine syn- amino acids, is formed by the reductive amidation of the citric thetase reaction (see Figure 27–2), but gutamine, rather than acid cyce intermediate α-ketogutarate, a reaction catayzed ammonium ion, provides the nitrogen. Bacteria asparagine by mitochondria gutamate dehydrogenase (Figure 27–1). synthetases can, however, aso use ammonium ion. The reac- The reaction both strongy favors gutamate synthesis and tion invoves the intermediate formation of asparty phosphate owers the concentration of cytotoxic ammonium ion. (Figure 27–6). The couped hydroysis of PPi to Pi by pyro- phosphatase, EC 3.6.1.1, ensures that the reaction is strongy favored. Glutamine The amidation of gutamate to gutamine catayzed by gutamine Serine synthetase (Figure 27–2) invoves the intermediate formation of Oxidation of the α-hydroxy group of the gycoytic interme- γ-gutamy phosphate (Figure 27–3). Foowing the ordered bind- diate 3-phosphogycerate, catayzed by 3-phosphogycerate ing of gutamate and ATP, gutamate attacks the γ-phosphorus of dehydrogenase, converts it to 3-phosphohydroxypyruvate. ATP, forming γ-gutamy phosphate and ADP. NH4+ then binds, Transamination and subsequent dephosphoryation then and uncharged NH3 attacks γ-gutamy phosphate. Reease of Pi form serine (Figure 27–7). and of a proton from the γ-amino group of the tetrahedra inter- mediate then aows reease of the product, gutamine. Glycine Gycine aminotransferases can catayze the synthesis of gy- Alanine & Aspartate cine from gyoxyate and gutamate or aanine. Unike most Transamination of pyruvate forms aanine (Figure 27–4). Simi- aminotransferase reactions, is strongy favored gycine syn- ary, transamination of oxaoacetate forms aspartate. thesis. Additiona important mammaian routes for gycine formation are from choine (Figure 27–8) and from serine (Figure 27–9). NH3+ NH3+ – O O– H2N O– O O O O L-Glutamate L-Glutamine NH4+ Mg-ATP Mg-ADP + Pi FIGURE 27–4 Formation of alanine by transamination of FIGURE 27–2 The reaction catalyzed by glutamine synthetase pyruvate. The amino donor may be glutamate or aspartate. The other (EC 6.3.1.2). product thus is α-ketoglutarate or oxaloacetate. 276 SECTION VI Metabolism of Proteins & Amino Acids + + O NH 3 O NH 3 – – O O – O H 2N O O L-Aspartate L-Asparagine H2O + Gln Glu Mg-ATP Mg-AMP + PPi FIGURE 27–5 The reaction catalyzed by asparagine synthe- tase (EC 6.3.5.4). Note similarities to and differences from the gluta- mine synthetase reaction (Figure 27–2). FIGURE 27–7 Serine biosynthesis. Oxidation of Proline 3-phosphoglycerate is catalyzed by 3-phosphoglycerate dehydroge- The initia reaction of proine biosynthesis converts the nase (EC 1.1.1.95). Transamination converts phosphohydroxypyruvate γ-carboxy group of gutamate to the mixed acid anhydride of to phosphoserine. Hydrolytic removal of the phosphoryl group cata- lyzed by phosphoserine hydrolase (EC 3.1.3.3) then forms l-serine. gutamate γ-phosphate (see Figure 27–3). Subsequent reduc- tion forms gutamate γ-semiadehyde, which foowing spon- taneous cycization is reduced to l-proine (Figure 27–10). Cysteine Whie not itsef nutritionay essentia, cysteine is formed from methionine, which is nutritionay essentia. Foowing con- version of methionine to homocysteine (see Figure 29–18), homocysteine and serine form cystathionine, whose hydroy- sis forms cysteine and homoserine (Figure 27–11). Tyrosine Phenyaanine hydroxyase converts phenyaanine to tyro- sine (Figure 27–12). If the diet contains adequate quantities of the nutritionay essentia amino acid phenyaanine, tyrosine is nutritionay nonessentia. However, since the phenyaanine hydroxyase reaction is irreversibe, dietary tyrosine cannot repace phenyaanine. Cataysis by this mixed-function oxidase incorporates one atom of O2 into the para position of phenyaa- nine and reduces the other atom to water. Reducing power, pro- vided as tetrahydrobiopterin, derives utimatey from NADPH. Hydroxyproline & Hydroxylysine FIGURE 27–8 Formation of glycine from choline. Catalysts Hydroxyproine and hydroxyysine occur principay in coagen. include choline dehydrogenase (EC 1.1.3.17), betaine aldehyde dehy- Since there is no tRNA for either hydroxyated amino acid, nei- drogenase (EC 1.2.1.8), betaine-homocysteine N-methyltransferase (EC 2.1.1.157), sarcosine dehydrogenase (EC 1.5.8.3), and dimethylgly- ther dietary hydroxyproine nor dietary hydroxyysine is incor- cine dehydrogenase (EC 1.5.8.4). porated during protein synthesis. Peptidy hydroxyproine and hydroxyysine arise from proine and ysine, but ony after these Methylene amino acids have been incorporated into peptides. Hydroxyation H4 folate H4 folate of peptidy proy and peptidy ysy residues, catayzed by prolyl NH3+ NH3+ hydroxylase and lysyl hydroxylase of skin, skeeta musce, and O – O– + HO O O O NH 3 – Serine H2O Glycine O –O PO 3 O FIGURE 27–9 Interconversion of serine and glycine, cata- lyzed by serine hydroxymethyltransferase (EC 2.1.2.1). The reac- FIGURE 27–6 Aspartyl phosphate. tion is freely reversible. (H4 folate, tetrahydrofolate.) CHAPTER 27 Biosynthesis of the Nutritionally Nonessential Amino Acids 277 FIGURE 27–11 Conversion of homocysteine and serine to homoserine and cysteine. The sulfur of cysteine derives from methio- nine and the carbon skeleton from serine. The catalysts are cystathionine β-synthase (EC 4.2.1.22) and cystathionine γ-lyase (EC 4.4.1.1). Valine, Leucine, & Isoleucine Whie eucine, vaine, and isoeucine are a nutritionay essentia amino acids, tissue aminotransferases reversiby interconvert a three amino acids and their corresponding α-keto acids. FIGURE 27–10 Biosynthesis of proline from glutamate. Catalysts for these reactions are glutamate-5-kinase (EC 2.7.2.11), glutamate-5-semialdehyde dehydrogenase (EC 1.2.1.41), and pyrroline- 5-carboxylate reductase (EC 1.5.1.2). Ring closure of glutamate semi- aldehyde is spontaneous. granuating wounds requires, in addition to the substrate, moec- uar O2, ascorbate, Fe2+, and α-ketogutarate (Figure 27–13). For every moe of proine or ysine hydroxyated, one moe of α-ketogutarate is decarboxyated to succinate. The hydroxyases are mixed-function oxidases. One atom of O2 is incorporated into proine or ysine, the other into succinate (see Figure 27–13). A FIGURE 27–12 Conversion of phenylalanine to tyrosine by deficiency of the vitamin C required for these two hydroxyases phenylalanine hydroxylase (EC 1.14.16.1). Two distinct enzymatic activities are involved. Activity II catalyzes reduction of dihydrobiop- resuts in scurvy, in which beeding gums, sweing joints, and terin by NADPH, and activity I the reduction of O2 to H2O and of phe- impaired wound heaing resut from the impaired stabiity of co- nylalanine to tyrosine. This reaction is associated with several defects agen (see Chapters 5 and 50). of phenylalanine metabolism discussed in Chapter 29. 278 SECTION VI Metabolism of Proteins & Amino Acids Subsequent repacement of the serine oxygen by seenium invoves seenophosphate formed by seenophosphate synthe- tase (see Figure 27–14). Successive enzyme-catayzed reactions convert cystey-tRNASec to aminoacryy-tRNASec and then to seenocystey-tRNASec. In the presence of a specific eongation factor that recognizes seenocystey-tRNASec, seenocysteine FIGURE 27–13 Hydroxylation of a proline-rich peptide. can then be incorporated into proteins. Molecular oxygen is incorporated into both succinate and proline. Procollagen-proline 4-hydroxylase (EC 1.14.11.2) thus is a mixed- function oxidase. Procollagen-lysine 5-hydroxylase (EC 1.14.11.4) SUMMARY catalyzes an analogous reaction. A vertebrates can form certain amino acids from amphiboic intermediates or from other dietary amino acids. The These α-keto acids thus can repace their corresponding amino intermediates and the amino acids to which they give rise are acids in the diet. α-ketogutarate (Gu, Gn, Pro, Hyp), oxaoacetate (Asp, Asn), and 3-phosphogycerate (Ser, Gy). Selenocysteine, the 21st Amino Acid Cysteine, tyrosine, and hydroxyysine are formed from nutritionay essentia amino acids. Serine provides the carbon Whie the occurrence of seenocysteine (Figure 27–14) in pro- skeeton and homocysteine the sufur for cysteine biosynthesis. teins is reativey uncommon, at east 25 human seenoproteins In scurvy, a nutritiona disease that resuts from a deficiency are known. Seenocysteine is present at the active site of severa of vitamin C, impaired hydroxyation of peptidy proine and human enzymes that catayze redox reactions. Exampes incude peptidy ysine resuts in a faiure to provide the substrates for thioredoxin reductase, gutathione peroxidase, and the deiodinase cross-inking of maturing coagens. that converts thyroxine to triiodothyronine. Where present, Phenyaanine hydroxyase converts phenyaanine to tyrosine. seenocysteine participates in the cataytic mechanism of these Since the reaction catayzed by this mixed function oxidase is enzymes. Significanty, the repacement of seenocysteine by irreversibe, tyrosine cannot give rise to phenyaanine. cysteine can actuay reduce cataytic activity. Impairments in Neither dietary hydroxyproine nor hydroxyysine is human seenoproteins have been impicated in tumorgenesis incorporated into proteins because no codon or tRNA dictates and atheroscerosis, and are associated with seenium deficiency their insertion into peptides. cardiomyopathy (Keshan disease). Peptidy hydroxyproine and hydroxyysine are formed Biosynthesis of seenocysteine requires serine, seenate by hydroxyation of peptidy proine or ysine in reactions (SeO42−), ATP, a specific tRNA, and severa enzymes. Serine pro- catayzed by mixed-function oxidases that require vitamin C as vides the carbon skeeton of seenocysteine. Seenophosphate, cofactor. formed from ATP and seenate (see Figure 27–14), serves as the Seenocysteine, an essentia active site residue in severa seenium donor. Unike 4-hydroxyproine or 5-hydroxyysine, mammaian enzymes, arises by cotransationa insertion from a seenocysteine arises cotranslationally during its incorporation previousy modified tRNA. into peptides. The UGA anticodon of the unusua tRNA caed tRNASec normay signas STOP. The abiity of the protein syn- thetic apparatus to identify a seenocysteine-specific UGA REFERENCES codon invoves the seenocysteine insertion eement, a stem- Gadyshev VN, Arner ES, Brigeius FR, et a: Seenoprotein gene oop structure in the untransated region of the mRNA. tRNASec nomencature. J Bio Chem 2016;291:20436. is first charged with serine by the igase that charges tRNASer. Kiberg MS: Asparagine synthetase chemotherapy. Annu Rev Biochem 2006;75:629. Rayman RP: Seenium and human heath. Lancet H 2012;379;9822;1256. H Se CH2 C COO– Ruzzo EK, Capo-Chichi JM, Ben-Zeev B, et a: Deficiency of NH3+ asparagine synthetase causes congenita microcephay and a O progressive form of encephaopathy. Neuron 2013;80:429. Sticke F, Inderbitzin D, Candinas D: Roe of nutrition in iver Se + ATP + H2O AMP + Pi + H Se P O– transpantation for end-stage chronic iver disease. Nutr Rev O– 2008;66:47. Turanov AA, Shchedrina VA, Everey RA, et a: Seenoprotein S FIGURE 27–14 Selenocysteine (top) and the reaction cata- is invoved in maintenance and transport of mutiprotein lyzed by selenophosphate synthetase (EC 2.7.9.3) (bottom). compexes. Biochem J 2014;462:555.