Medical Physiology PDF Handouts for L25-Cardiac Pump & L26-Cardiac Cycle
Document Details
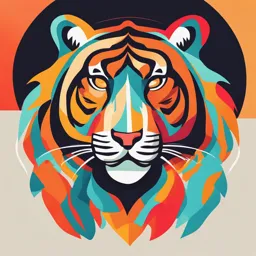
Uploaded by LuminousStonehenge6365
Tulane University
Dr. Dewan Majid
Tags
Summary
This document is a set of medical physiology handouts focusing on the cardiac pump and cardiac cycle. It discusses learning objectives, preload, afterload, stroke volume, and other key concepts related to cardiac function.
Full Transcript
Medical Physiology Dr. Dewan Majid Handouts for: L25 - Cardiac Pump L26 – Cardiac Cycle Learning Objectives: L25: Cardiac Pump The students should be able to: 1) define preload, after...
Medical Physiology Dr. Dewan Majid Handouts for: L25 - Cardiac Pump L26 – Cardiac Cycle Learning Objectives: L25: Cardiac Pump The students should be able to: 1) define preload, afterload, stroke volume, cardiac output, ejection fraction, cardiac work, and cardiac efficiency. 2) describe the factors influencing cardiac pump activity. 3) describe the ‘Frank-Starling relationship regulating ventricular contraction (Ventricular function curve) and explain its significance in cardiac function. 4) describe the indices of myocardial fiber length and ventricular performance. 5) understand how the heterometric autoregulation of both ventricular output is maintained. L26: Cardiac Cycle The students should be able to: 1) describe the sequential systolic and diastolic phases in a cardiac cycle. 2) describe the valvular mechanism that regulates the blood flow within the heart chambers. 3) describe the importance of changes in pressure within the heart chambers that regulate the valvular action. 4) reconstruct the Wiggar’s diagram describing the chronology of events (both mechanical and electrical) in one cardiac cycle. 5)describe the ventricular pressure-volume relationship in a single cardiac cycle and explain its significance to determine cardiac functional abnormalities. 6) describe the mechanisms of production of the normal and abnormal heart sounds. 7) identify the common causes of murmur/thrill. 1. Some terms and relationships that you must know: Preload and Afterload: In assessing the contractile properties of muscle, it is important to specify the initial degree of stretch in the muscle when it begins to contract, which is called the preload, and also to specify the load against which the muscle exerts its contractile force, which is called the afterload. In case of myocardial contraction, the preload refers to the stretch of the left ventricular muscle just before the onset of contraction and this stress depends on the volume of blood in the ventricle at the end of diastolic period (end-diastolic volume). Thus, preload in the context of cardiac contractility generally refers to end-diastolic volume, which is the volume of blood contained in the left ventricle at the end of diastole or just before contraction. The preload for the left ventricle is left ventricular end- diastolic volume, or end-diastolic fiber length; that is, preload is the resting length from which the muscle contracts. The relationship between preload (myocardial fiber length) and the developed force in myocardium during systole and diastole is illustrated in Figure 1 below, are mainly based on the degree of overlapping of thick and thin filaments of the myocardium. 1 Medical Physiology Dr. Dewan Majid Figure1: Relationship of myocardial resting fiber length (sarcomere length) or end-diastolic volume to the force developed or peak systolic ventricular pressure during ventricular contraction in an intact heart. Examples: 1) Factors that increases preload: a) Large volume infusion of saline b) Large volume blood transfusion c) Exercise or increased physical activity that resulted in an increase in venous return due to peripheral vasoconstriction. 2) Factors that decreases preload: a) Acute hemorrhage (Large volume of blood loss that resulted in a decrease in venous return ) b) Venous dilation (generally drug induced - nitroglycerine have predominantly relax venous smooth muscle that resulted in a decrease in venous return) The afterload is the load on ventricular myocytes during myocardial contraction. Therefore, afterload is the magnitude of load the heart must overcome to eject blood. It thus refers to the aortic pressure during the period when the ventricle has to eject blood volume in the aorta. Thus, afterload in the context of cardiac contractility generally refers to aortic blood pressure. Examples: Factors that increases afterload: a) Aortic constriction b) Increased sympathetic activity- increased peripheral vascular resistance c) Hypertension Factors that decreases afterload: a) Arterial vasodilation: generally drug induced – use of vasodilators; Hydralazine, Ca++Channel blockers etc. or anti-vasoconstrictor agents; Angiotensin II (AngII) receptor antagonist – Angiotensin converting enzyme (ACE) inhibitor. Stroke Volume: The amount of blood pumped out from each ventricle per beat. This is calculated as the difference of the amount of blood in the ventricle between two periods: at the end of diastole (end- diastolic volume) and at the end of systole (end-systolic volume). Stroke volume (SV) = End-diastolic volume (EDV) – End-systolic volume (ESV). 2 Medical Physiology Dr. Dewan Majid Cardiac Output: The amount of blood pumped out from each ventricle per minute. This is calculated as the product of stroke volume and the heart rate. Cardiac Output (CO) = Stroke volume (SV) x Heart rate (HR) Ejection Fraction: The ratio of the volume of blood ejected from the ventricle during systole (stroke volume) to the volume of blood present in the ventricle at the end of diastole (end-diastolic volume) i.e. just prior to the act of systole. Ejection fraction (EF) = Stroke volume (SV) / End-diastolic volume (EDV) Normal values to know: Values/sq.meter Typical values in a body surface area 70-kg individual (1.78 sq.meter approx.is the typical value of surface area) Cardiac Output 5600 mL 3146 mL (cardiac index)* End diastolic 125 mL 70 mL volume End systolic 45 mL 24 mL volume Stroke 80 mL 45 mL Volume Ejection 0.64 or 64% * Cardiac Index: When cardiac output (CO) is divided by body surface area (BSA), it is called the cardiac index (CI). Experiments have shown that the cardiac output increases approximately in proportion to the surface area of the body. Therefore, cardiac output is frequently stated in terms of the cardiac index, which is the cardiac output per square meter of body surface area. The average human being who weighs 70 kilograms has a body surface area of about 1.7 square meters, which means that the normal average cardiac index for adults is about 3 L/min/m 2 of body surface area. Individuals of same body size usually have same cardiac output at rest; however, this varies depending on other conditions. Let us say two individuals have the same body size. One in an athletic and another is a sedentary worker. They should have about the same cardiac output at rest (in this example 5.6 liters/min). The trained person might have a resting heart rate of 56 beats per minute and a stroke volume of 100ml/beat. The untrained person might have a heart rate of 70 beats per minute and a stroke volume of 80 ml/beat. Thus, the hearts of these two individuals meet the resting metabolic needs of the body in different ways. When these two people begin to exercise, again the hearts respond differently. The sedentary individual increases his/her cardiac output primarily by increasing heart rate. Stroke volume may increase but generally not much (perhaps 10%). Cardiac output may increase to three times the normal value. The athlete, in contrast, can both increase heart rate (to a higher level than the sedentary person) and stroke volume (perhaps 50% or more) and thus is capable of increasing cardiac output perhaps six or more times the resting level. Clearly we need a way to compare the cardiac output and other hemodynamic parameters measured in people of different age, sex, height and weight, etc. One way to do this is to express the value per sq. meter of body surface area. Cardiac work: Work is defined as the force multiplied by the distance of the object displaced by force. In terms of myocardial function, “work” is stroke work or the work the heart performs on each beat. For the left ventricle, stroke work is stroke volume multiplied by aortic pressure, where aortic pressure corresponds to force and stroke 3 Medical Physiology Dr. Dewan Majid volume corresponds to distance. The work output of the heart is the amount of energy that the heart converts to work while pumping blood into the arteries. Cardiac work can be calculated in a number of different ways. Simply, it can be calculated using the usual formula for work: Work = Force applied X Velocity of the object displacement When considering cardiac work under conditions of steady flow, force can be represented as the developed ejection pressure during ventricular contraction and the velocity of the object displacement can be represented as the stroke volume achieved during ventricular contraction. Thus, Cardiac work (W) = Ejection pressure (P) X Stroke volume output (V). The work performed by the left ventricle per beat (stroke work) is equal to the mean left ventricular ejection pressure times the stroke volume output. The work performed by the right ventricle per beat (stroke work) is equal to the mean right ventricular ejection pressure times the stroke volume output. Minute work or power is defined as work per unit time. In terms of myocardial function, cardiac minute work is cardiac output multiplied by aortic pressure. Therefore, cardiac minute work can be considered to have two components: volume work (i.e., cardiac output) and pressure work (i.e., aortic pressure). Sometimes the volume work component is called “external” work, and the pressure work component is called “internal” work. Thus, increases in cardiac output (due to an increase in stroke volume and/or an increase in heart rate) or increases in aortic pressure will increase the work of the heart. Remember! Left Ventricular stroke work is about 6 times the stroke work of the right ventricle. Cardiac Efficiency: Cardiac efficiency is the ratio of work done by the heart to the energy used to perform the work. This measurement is crucial for the diagnosis and management of several cardiac diseases including cardiomyopathy, hypertension and cardiac failure. Cardiac muscle utilizes chemical energy to provide the work of contraction. This energy is derived mainly from metabolism of fatty acids and to a lesser extent from metabolism of other nutrients like lactate and glucose. During muscular contraction most of the chemical energy is converted into heat and a small portion into work output. Basically, the ratio of the work output to the chemical energy expended by the cardiac muscle is called the ‘Cardiac Efficiency’. Efficiency is normally about 10 - 15 percent. During severe exercise, efficiency may increase to 20 percent in a normal person and up to 25 percent in a well-trained athlete. 2. Frank-Starling Relationship This phenomenon was identified with the work of two famous physiologists: Otto Frank and Earnest Starling. It also is described as ‘Frank-Starling Mechanism’ as well as ‘Starling law of the heart’. The graph is simply a length tension relationship as described for skeletal muscle. Basically it states that the contraction of the ventricles increases in proportion to the increased preload (i.e., the venous return to the heart). That means the volume of blood ejected by the ventricle depends on the volume present in the ventricle at the end of diastole (i.e., the output of blood is in accordance with the input). This relationship also is denoted as the ‘ventricular function curve’ (Figure 2) which is described as ‘The amount of blood pumped out of the heart during each contraction (Stroke Volume) is directly proportional to diastolic filling (End-diastolic Volume). The Frank- Starling law works only within limits: if the optimal lengthening of sarcomere is exceeded, the contractions become less powerful and thus the ventricular performance level (Stroke Volume) decreases. 4 Medical Physiology Dr. Dewan Majid Figure 2: The Frank–Starling relationship representative ventricular function. The effect of preload variation on the stroke volume is given as an indication of ventricular performance. The dotted portion of the curve represents the range of values of end-diastolic volume and stroke volume in a normal functioning heart. 3. Indices of myocardial fiber length and ventricular performance: Many years after Frank and Starling defined their law, it was also shown that the increased end- diastolic pressure leads to lengthening of the sarcomeres which thereupon contract more forcefully. In consideration of the ventricular function curve, it should also be considered that the changes in myocardial fiber length can be represented not only by the changes in end-diastolic volume or pressure, but also by changes in ventricular circumference. Changes in the atrial volume/pressure due to changes in venous return can also result in changes in sarcomere length. On the other hand, ventricular performance can be measured not only by the Stroke volume but also by the cardiac output, stroke work or cardiac work. According to Starling’s law of the heart, any change in the indices of myocardial fiber length will results a linearchanges in all the indices of ventricular performance as described by the ventricular function curve. 5 Medical Physiology Dr. Dewan Majid 4. Factors determining Stroke volume: Changes in the stroke volume can be produced by a variety of factors, both intrinsic and extrinsic to the heart. In general, the amount of the stroke volume depends mainly on the status of cardiac pump activity which primarily depends on two dominant factors in most physiological and pathophysiological conditions. These are: A) Contractile force of the ventricular muscle B) Pressures in the aorta/pulmonary artery Contractile force of the myocardium exerts positive impact while the aortic/pulmonary pressure exerts negative impact on the Stroke Volume. As mentioned earlier, generation of contractile force in the myocardium mainly is governed by the preload i.e., by the Starling’s law of the heart. However, at a constant preload, the contractility of the myocardium can also be increased by increasing the velocity of sarcomere shortening. Contractility is the strength of contraction, independent of the preload (i.e., at a given myocardial fiber length or end-diastolic volume). Contractility is regulated by chemical or hormonal influences (e.g., catecholamines) on the force of contraction. As catecholamine levels increase, there is an increase in the influx of calcium into the cardiac muscle fiber with each action potential, which leads to enhanced contractile force via calcium-induced calcium release into the sarcoplasmic reticulum. The aortic/pulmonary pressure exerts the resistance against which the ventricle works is termed, afterload. In the left ventricle, afterload may be caused by narrowing of the arteries or arterial hypertension, as well as by narrowing of the aortic valves by rheumatic disease or calcification. Afterload to the right ventricle results from various conditions causing pulmonary hypertension or left ventricular failure. Factors in determining Stroke Volume Preload Contractility Afterload + + _ Stroke Volume 5. Cardiac Cycle and its systolic & diastolic phases The cardiac cycle is the sequence of electrical and mechanical events occurring in the heart during a single beat and the resulting changes in pressure. The heart is a dual pump in that the left and right sides of the heart pump blood separately, but simultaneously, into the systemic and pulmonary circuits. Efficient pumping of blood requires that the atria contract first, followed immediately by the ventricles. The contraction of cardiac muscle is triggered by depolarization of the plasma membrane. The orderly process of depolarization triggers a recurring cardiac cycle of atrial and ventricular contractions and relaxations. One cardiac cycle is the period that elapses from the initiation of one contraction to the next one. At a heart rate of 75, one cardiac cycle period is only 0.8 sec.The chronology of events that occurs in one cardiac cycle is illustrated in a schematic diagram below: 6 Medical Physiology Dr. Dewan Majid Figure 3: Schematic representation of a cardiac cycle; Black areas represent systole and open areas represent diastole. 7. Valvular mechanism of blood flow inside the heart The heart can be classified as a reciprocating pump. The principles that regulate flow into and out of the ventricle are identical to those for the reciprocating pump which are simple as illustrated in the figure below. The pumping chamber has a variable volume and an input and an output port. A one-way valve in the input port is oriented such that it opens only when the pressure in the input chamber exceeds pressure within the pumping chamber. Another one-way valve in the output port opens only when pressure in the pumping chambers exceeds the pressure in the output chamber. As the diaphragm moves back and forth the chamber’s volume is constantly changing causing the pressure within to rise and fall. In the example, the rod and crankshaft cause the diaphragm to move back and forth. In the heart, that motion is the result of contraction and relaxation of the cardiac muscle which makes up the ventricular wall. Figure 4: A schematic representation of a reciprocating pump having a pumping chamber and input and output ports with oppositely oriented valves. 7 Medical Physiology Dr. Dewan Majid 8. Valvular action during changes in pressure within the heart chambers The temporal relationship between the changes in pressures within the heart chambers and the valvular function is illustrated in the figure below. As mentioned earlier, the chronology of events in a cardiac cycle usually follow the changes in the pressure and volume within the heart chambers that lead to closing and opening of the valves. Figure 5: Sequential changes in valve function in the heart leads to chronology of events in a cardiac cycle. 9. Chronology of events in a cardiac cycle The cardiac cycle refers to the sequence of electrical and mechanical events occurring in the heart during a single beat and the resulting changes in pressure, flow and volume in the various cardiacchambers. The functional interrelationships of the cardiac cycle are represented in the figure below. This is a modified figure of ‘Wiggers’ diagram’ named according to the name of a physiologist who originally described it. This figure illustrates how aortic pressure, ventricular pressure, atrial pressure, and ventricular volume are temporally correlated throughout the phases in a cardiac cycle. The cycle of events described here are for the left heart. However, these events occur almost simultaneously in the right and left heart; the main difference is that the pressures are higher on the left side. The values depicted in these graphs are representative of the values obtained in experiments in anesthetized dogs. 8 Medical Physiology Dr. Dewan Majid Figure 6: Modified Wiggers’ diagram illustrating chronology of events in one cardiac cycle. 10. Pressure-Volume relationship in one cardiac cycle An important determinant of cardiac function is the ventricular pressure-volume loop, which relates changes in ventricular volume to corresponding changes in pressure throughout the cardiac cycle. This is illustrated in the figure below. The pressure and volume values depicted in in this figure are representative to the values usually corresponds in a human subject. Changes in any of the determinant of cardiac function are reflected by alterations in the pressure volume loop. By analyzing the effects of a change in an individual parameter (preload, afterload, or contractility) on the pressure- volume loop, the resulting alterations in ventricular pressure and stroke volume can be predicted. 9 Medical Physiology Dr. Dewan Majid Pressure-Volume Loop for the left ventricle 120 Ventricular pressure (mmHg) c AV AV 80 closing b opening ESV SV 40 EDV MV a MV opening closing d 0 0 50 100 150 Ventricular volume (mL) Figure 7: A typical diagram for the ‘Pressure-Volume loop’ for the left ventricle representing the values in human. EDV, end-diastolic volume; ESV, end-systolic volume; SV, stroke volume; MV, mitral valve; AV, atrio-ventricular valves. a, closing of mitral valve; b, opening of aortic valve; c, closing of aortic valve; d, opening of mitral valve. Figure 8: Changes in the left ventricular pressure-volume loop in different conditions. A, Increased preload; B, increased afterload; C, increased contractility; The normal ventricular cycle is shown by the solid lines, and the effect of the change is shown by the dashed lines. 10 Medical Physiology Dr. Dewan Majid 11. Description of normal heart sounds 1st heart sound (S1): - Loudest and longer duration - Occurs at the isovolumetric contraction phase - Causes are: a) Closure of atrio-ventricular valves (mitral and tricuspid) b) Oscillation of blood in the closed ventricle c) Vibration of the ventricular wall during contraction 2nd heart sound (S2): - Lower intensity and shorter duration - Occurs at the isovolumetric relaxation phase - Cause: Closure of semilunar valves (aortic and pulmonic) 3rd heart sound (S3): - Usually not audible - Can be heard in children with thin chest, patient with left ventricular failure - Cause: Vibration of the ventricular wall at the first phase of early diastole - A pathologic S3 is often referred to as a ventricular “Gallop” 4th heart sound (S4): - Also known as atrial heart sound - Usually not audible - Cause: Oscillation of blood during atrial contraction 12. Description of cardiac murmurs A murmur is the sound generated by turbulant blood flow. Cardiac Murmurs is the sounds generated by turbulent blood flow in the cardiac chambers due to abnormalities in valve function or congenital heart defects. Cardiac Murmurs in result from the following mechanisms: 1) Flow across partial obstruction (stenosis of valves) 2) Regurgitant flow across and incompetant valve (regurgitation of valves) 3) Abnormal shunting of blood from high –pressure to a lower –pressure vascular chamber (Ventricular septal defect) 4) Increased flow through normal structures (such as in Anemia) 5) Ejection into a dilated chamber (aneurysmal dilatation of the aorta) Abnormalities in cardiac valves: a) Stenosis (inadequate opening) b) Regurgitation (improper closing) Congenital heart defects: Ventricular septal defect, Atrial septal defect, Patent ductus arteriosus, Coarctation of the aorta etc. Cardiac murmurs are occasionally so severe that they can be felt with the hand on the cardiac region of the chest wall. This is known as thrill (or palpable thrill). Types of Murmurs: Systolic Murmurs: Murmurs occurs during the period of systole. Examples: Stenosis of aortic or pulmonic valves, Regurgitation of mitral or tricuspid valves, Ventricular septal defect etc. Diastolic Murmurs: Murmurs occurs during the period of diastole.Examples: Mitral stenosis, regurgitation of aortic or pulmonic valves etc. 11