Gen Bio 1 Reviewer PDF
Document Details
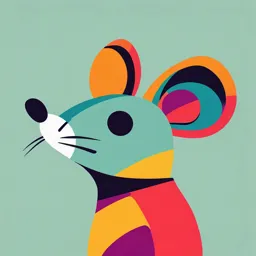
Uploaded by PrudentIambicPentameter
Tags
Summary
This document summarizes lessons on biological processes, focusing on energy (ATP) and the role of pigments in photosynthesis. It explains the structure and function of ATP, coupled reaction processes, and different types of pigments involved in energy capture for cellular processes.
Full Transcript
– Lesson 1: ATP and the Different Coupled Reaction Processes A Summary of Gen Bio 1 - Lesson 1 and 1.-ATP-and-The-Different-Coupled-Reaction-Processes.pptx ATP Structure and Hydrolysis How do organisms carry out essential life processes? Cells in organisms obtain energy from the chemical bonds...
– Lesson 1: ATP and the Different Coupled Reaction Processes A Summary of Gen Bio 1 - Lesson 1 and 1.-ATP-and-The-Different-Coupled-Reaction-Processes.pptx ATP Structure and Hydrolysis How do organisms carry out essential life processes? Cells in organisms obtain energy from the chemical bonds that hold together certain organic compounds, such as carbohydrates from the food that we eat. This energy in turn is used to produce adenosine triphosphate (ATP). ATP is an organic molecule used for short-term energy storage and transport in the cell. It is composed of three parts: (1) a nitrogenous base (adenine), (2) a sugar (ribose), and (3) three phosphate groups The three phosphate groups in an ATP molecule are negatively charged. Recall that molecules having the same charge will tend to repel from each other. Thus, this means that the three phosphate groups are in an unstable arrangement. The third phosphate group is so eager to get further away from the two phosphate groups. A bond between them is broken through hydrolysis (water-mediated breakdown) reaction releasing energy (Figure 2). The remaining free phosphate group and low-energy molecule is called adenosine diphosphate (ADP). Pi stands for an inorganic phosphate group. ATP is hydrolyzed in (?)*. ATP + H2O →← ADP + Pi + energy ATP - ADP Cycle ATP → ADP: phosphate removed, energy released ADP → ATP: phosphate added, energy added - ATP can be produced from existing ADP molecules - A phosphate is added to ADP at the mitochondria. - Requires ATP synthase - A protein complex in the mitochondria that acts a molecular mill and converts ADP into ATP. ATP-ADP Cycle The hydrolysis of ATP to ADP is reversible. ATP and ADP are like charged and uncharged forms of a rechargeable battery. ATP (charged battery) has energy that can be used to power cellular processes or reactions. Once the energy is used up, ADP (uncharged battery/dead battery) needs to be recharged in order to be used as a power source. ATP regeneration reaction is the reverse of hydrolysis reaction: energy + ADP + Pi →← ATP + H2O ATP in Energy Coupling How is the energy released by ATP hydrolysis used to power cells to carry out useful functions? The hydrolysis of ATP not only results in a release of energy but also would simply result in organisms’ overheating because the dissipation of energy would excite nearby molecules, resulting in heat or thermal energy. Energy in a cell needs to be linked to other processes to be useful. Energy coupling is the transfer of energy from one chemical reaction to another. An energetically favorable reaction (exergonic, e.g., ATP hydrolysis) is directly linked with an energetically unfavorable reaction (endergonic, e.g., ATP regeneration). Through energy coupling. Coupled Reactions a. ATP breakdown is exergonic b. Muscle contraction is endergonic and cannot occur without an input of energy c. Muscle contraction becomes exergonic and can occur when it is coupled to ATP breakdown Exergonic Reaction - products have less energy than reactants, spontaneous Endergonic Reaction - products have more energy than reactants, not spontaneous Chemical reactions can be classified as either exergonic or endergonic Exergonic reaction - proceeds with a net release of free energy Endergonic reaction - one that absorbs free energy from its surroundings Formation of sucrose Uncoupled Reaction: Glucose + Fructose → Surcrose Coupled Reaction: Glucose + ATP → Glucose P + ADP Glucose-P + Fructose → Sucrose + Pi One example of energy coupling involving ATP is the formation of sucrose (table sugar) from glucose and fructose (Figure 5). In the uncoupled reaction, glucose and fructose combine to form sucrose. In the coupled reaction, there are two reactions that take place: 1. A phosphate group is transferred from ATP to glucose, forming a phosphorylated glucose intermediate (glucose-P). This is an energetically favorable reaction or exergonic reaction. 2. The glucose-P intermediate reacts with fructose to form sucrose. Because glucose-P is relatively unstable, this reaction also releases energy and is spontaneous. Lesson 2: Pigments A Summary of Gen Bio 1 - Lesson 2 and 2.-Pigments.pptx The importance of chlorophyll and other pigments Chlorophyll Chlorophyll is any of several related green pigments found in cyanobacteria and in the chloroplasts of algae and plants. Chlorophyll a is the core pigment that absorbs sunlight for light dependent photosynthesis. All organisms that perform photosynthesis have chlorophyll A, but not all organisms contain chlorophyll B. What Are Accessory Pigments? Accessory pigments have a slightly different molecular structure than chlorophyll a that facilitates absorption of different colors on the light spectrum. Chlorophyll b and c reflect varying shades of green light, which is why leaves and plants are not all the same shade of green. Chlorophyll a masks the less abundant accessory pigments in leaves until fall when production stops. In the absence of chlorophyll, the dazzling colors of accessory pigments hidden in the leaves are revealed. Types of accessory pigments: 1. Chlorophyll b It is structurally slightly different from chlorophyll a but its absorption spectrum is somewhat different. It absorbs more in the blue and orange-red ranges. It looks yellowish green. Captured energy is handed over to chlorophyll a, which is a smaller but more plentiful molecule in the chloroplast. Chlorophyll B absorbs blue light. Chlorophyll B’s central role is to expand the absorption spectrum of organisms. The presence of chlorophyll B in cells helps organisms convert a wider range of the energy from the sun into chemical energy. Having more chlorophyll B in chloroplasts of cells is adaptive. Plants that receive less sunlight have more chlorophyll B in their chloroplasts. An increase in chlorophyll B is an adaption to the shade, as it allows the plant to absorb a broader range of wavelengths of light. Chlorophyll B transfers the extra energy it absorbs to chlorophyll A. 2. Carotenoids They absorb light from violet to the greenish-blue range. They appear in various shades of yellow or yellow-orange to our eyes. They cluster next to chlorophyll a molecules to efficiently hand off absorbed photons. They are usually found attached to proteins or membranes in the chloroplasts. 3. Anthocyanins They do not participate in photosynthesis and may appear red, purple, or blue. They occur widely among higher plants. They are pigments that generally give color to flowers but also occur in leaves and fruits. In leaves, these pigments often help to protect against excessive sunlight that can damage some leaf tissues. This is one reason why a young, newly developing leaf is often redder than when it reaches its mature size. 4. Xanthophylls They pass along light energy to chlorophyll a and act as antioxidants. The molecular structure gives xanthophylls the ability to accept or donate electrons. Xanthophyll pigments produce the yellow color in fall leaves. Function of Photosynthetic Pigments Photosynthesis occurs within stacks of flat disks called grana located in the stroma of plant cell organelles. Accessory photosynthetic pigments trap photons missed by chlorophyll a. Photosynthetic pigments can also inhibit photosynthesis when energy levels within the cell are too high. The concentration of photosynthetic and antenna pigments in plant cells varies depending on the light needs of the plant and access to sunlight during the light dependent cycle of photosynthesis. Chlorophyll degradation Chlorophyll degradation is an important catabolic process that is catalyzed by a multistep pathway and occurs during leaf senescence and fruit ripening. Degreening, due to the net loss of chlorophyll, is the most prominent symptom during the processes of leaf senescence, fruit ripening, and seed maturation. Senescence Senescence refers to the endogenously-controlled deteriorative changes, which are natural causes of death in cells, tissues, organs or organisms. Ready-to-eat salads (chlorophyll → colorless compounds); induced by ethylene Stored cabbage heads (chlorophyll → colorless compounds); induced by ethylene A vital during leaf senescence and fruit ripening, as it allows for recycling of nitrogen and other nutrients and for protection from buildup of phototoxic chlorophyll intermediates. Example of Degradation (Fruit maturation) Preservation (Freezing and Blanching) What Are Antenna Pigments? Photosynthetic pigments like chlorophyll b and carotenoids bond with protein to form a tightly packed antenna-like structure to capture incoming photons. Antenna pigments absorb radiant energy, somewhat like solar panels on a house. Antenna pigments pump photons into reaction centers as part of the photosynthetic process. Photons excite an electron in the cell that is then handed off to a nearby acceptor molecule and ultimately used in making ATP molecules. Where do the pigments occur in plants? The leaves of plants have mesophyll cells, the photosynthetic cells. These cells possess specialized structures called chloroplasts where photosynthetic pigments are located. Other pigments that are not involved in photosynthesis are stored in the vacuole, a large cellular structure that also serves as storage place of water and nutrients. The presence of accessory pigments explains why not all leaves are green. Accessory pigments are important since they help absorb light and then pass the energy to chlorophyll a, a primary pigment. Chlorophylls appear green because the pigments absorb light on all of the color ranges, and only green is transmitted to our eyes. Chlorophyll a is the core pigment that absorbs sunlight for light-dependent photosynthesis. It readily absorbs violet/blue and red light but not much of the lighter blue, and green and yellow light. It looks bluish green. Lesson 3: Chromatography A Summary of Gen Bio 1 - Lesson 3 and 3.-Chromatography.pptx Chromatography Chromatography is a process for separating components of a mixture. It is an important biophysical technique that enables the separation, identification, and purification of the components of a mixture for qualitative and quantitative analysis. Two phases of chromatography: a. mobile phase - where the molecules can move. b. stationary phase - where the molecules can’t move. In the case of paper chromatography, the stationary phase is the filter paper, and the mobile phase is the solvent (water). What is a stationary phase? the phase that is either a solid or liquid particle attached to a glass or a metal surface on which the components of the mixture to be separated is absorbed selectively. Most substances used as stationary phases are porous, thus allowing the attachment of components during chromatography. The stationary phase to be selected for a chromatographic process depends on the nature of the components to be separated and the type of chromatography. What is the mobile phase? The mobile phase in chromatography is the phase that is either liquid or gas that is passed through a chromatographic system where the components of the mixture are separated at different rates by adsorbing them to the stationary phase. Alcohol, water, acetic acid, acetone, or some gases are the commonly used mobile phase in different chromatographic techniques. Types of chromatography: 1. Paper Chromatography Principle: Separation occurs because different substances have different affinities for the stationary phase (paper) and the mobile phase (solvent). Example: Separation of pigments from a leaf extract. Real-Life Application: Used in forensic science for analyzing inks, dyes, and drugs. It’s also commonly used in schools and labs to separate plant pigments or check the purity of compounds. 2. Adsorption Chromatography different compounds are adsorbed to different degrees based on the absorptivity of the component. Here also, a mobile phase is made to move over a stationary phase, thus carrying the components with higher absorptivity to a lower distance than that with lower absorptivity. Real-Life Applications of Adsorption Chromatography: a. Pharmaceuticals to separate & purify drugs, ensuring the removal of impurities. b. Environmental Analysis: to detect and quantify pollutants in air, water, and soil by separating and analyzing complex mixtures of contaminants. c. Food Industry: helps in the analysis & identification of food additives, contaminants, and natural ingredients. d. Forensic Science: used to analyze samples like drugs, toxins, and explosives from crime scenes. e. Biochemical Research: Used for the separation of biomolecules like proteins, lipids, & nucleotides based on their adsorption properties. 3. Thin Layer Chromatography (TLC) Principle: Similar to paper chromatography, but here a thin layer of an adsorbent (silica or alumina) is coated on a plate. Compounds are separated based on their movement up the plate with the solvent. Example: Separation of essential oils. Real-Life Application: TLC is widely used in the pharmaceutical industry for purity testing, identification of chemicals, and monitoring reactions. In forensic labs, it’s used for drug analysis. 4. Column Chromatography Principle: A column is filled with a solid adsorbent (stationary phase), and a solvent (mobile phase) carries the mixture through the column. The components separate based on their adsorption rates. Example: Separation of caffeine from a coffee extract. Real-Life Application: used in drug formulation, purification of natural compounds, and separating components of perfumes and essential oils. It is also widely used in organic synthesis for purifying products. 5. Partition chromatography In this process, a continuous differential partitioning of components of a mixture into a stationary phase and mobile phase takes place. The example of partition chromatography can be seen in paper chromatography where paper is used as a stationary phase which is suspended in a mixture of solvents that act as a mobile phase, the components are carried to different degrees depending upon their retention on the paper. The components are thus separated at different heights. Real-Life Applications of Partition Chromatography: a. Pharmaceutical Industry (Purification of Drugs) Partition chromatography is commonly used to separate and purify complex mixtures of organic compounds in the pharmaceutical industry. For example, during the development of new drugs, scientists often need to separate the active ingredients from impurities or other closely related compounds. Example: In the production of antibiotics, liquid-liquid partition chromatography is used to separate different antibiotics or to purify them from fermentation broths. b. Food and Beverage Industry In the food industry, partition chromatography can be used for quality control by identifying and quantifying different components in food products such as flavors, preservatives, or nutritional additives. Example: The analysis of essential oils or food colorants often involves gas-liquid chromatography (GLC) to separate different volatile components to ensure quality and authenticity. 6. Gas Chromatography (GC) Principle: A gas (helium or nitrogen) is used as the mobile phase, while the stationary phase is a liquid or solid inside a column. Components are separated based on their volatility. Example: Separation and analysis of alcohol levels in blood samples. Real-Life Application: Gas chromatography is crucial in environmental monitoring for detecting pollutants, testing food and beverages for quality control, and forensic toxicology for drug analysis. It's also used in the petrochemical industry for analyzing gasoline. What is the Rf value in chromatography? The Rf value (Retention factor or Ratio of fronts) is a quantitative measure used to describe the movement of a solute (or compound) relative to the movement of the solvent front during a chromatography experiment. The Rf value is a critical parameter in chromatography that helps in identifying compounds and comparing their behavior under specific experimental conditions. How to Calculate the Rf Value; The Rf value is calculated using the formula: Rf = Distance traveled by the solute (compound) / Distance traveled by the solvent front Distance traveled by the solute: This is the distance from the baseline (where the sample was initially applied) to the center of the spot representing the compound after development. Distance traveled by the solvent front: This is the distance from the baseline to the furthest point the solvent reaches during the experiment. Significance of the Rf Value: - Comparison: The Rf value allows for comparison between different compounds in the same solvent and experimental conditions. Different compounds have different affinities for the stationary and mobile phases, leading to unique Rf values. - Identification: By comparing the Rf value of a compound to standard Rf values obtained from known substances, the identity of the compound can be inferred. - Purity Check: Multiple spots on the chromatogram with different Rf values can indicate the presence of impurities or multiple components in a mixture. Factors Affecting the Rf Value: 1. Nature of the Solvent: The choice of solvent can affect how far a compound travels. 2. Type of Stationary Phase: The affinity of the compound for the stationary phase will influence its movement. 3. Temperature: Changes in temperature can alter the solvent's behavior and the solubility of the compound. 4. Sample Size: Applying too much sample can distort the spots, leading to inaccurate Rf values. ggs