Fundamental Pharmacology 2020 PDF
Document Details
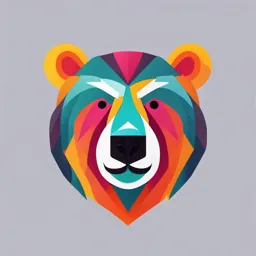
Uploaded by PanoramicBay
Faculty of Veterinary Science, Onderstepoort
2020
Adrian S.W. Tordiffe
Tags
Summary
This document is an outline for a veterinary pharmacology course offered at the Faculty of Veterinary Science in ONDERSTEPOORT, South Africa. It covers the fundamentals of veterinary pharmacology, including foundational knowledge, historical context, the scope of pharmacology, and veterinary pharmaceutics. Chapters delve into drug formulations, delivery systems, and the importance of active and inactive ingredients in drug efficacy. This is NOT a past paper but course notes.
Full Transcript
Copyright Reserved GENERAL VETERINARY PHARMACOLOGY 300 FUNDAMENTALS OF VETERINARY PHARMACOLOGY Prof Adrian S.W. Tordiffe Section of Pharmacology and Toxicology Department of Paraclinical Sciences Facu...
Copyright Reserved GENERAL VETERINARY PHARMACOLOGY 300 FUNDAMENTALS OF VETERINARY PHARMACOLOGY Prof Adrian S.W. Tordiffe Section of Pharmacology and Toxicology Department of Paraclinical Sciences Faculty of Veterinary Science ONDERSTEPOORT 2020 FUNDAMENTALS OF VETERINARY PHARMACOLOGY OUTCOME STATEMENT The student must know and be able to apply the principles and factors related to veterinary pharmaceutics, pharmacokinetics, pharmacodynamics, pharmacotherapy, and the legal requirements for registration and control of veterinary products in South Africa. 1. OVERVIEW OF VETERINARY PHARMACOLOGY KNOWLEDGE REQUIRED 1. Define the following: a. Pharmacology b. Pharmacy Pharmacology may be defined as the study of the effect drugs have on living things, and drugs as chemical substances which modify the functions of living things. It embraces the knowledge of the history, source, physical and chemical properties, compounding, biochemical and physiological effects, mechanism of action, absorption, distribution, biotransformation, excretion and therapeutic and other uses of drugs. Pharmacy is the preparation of drugs and their formulation into remedies. In earlier decades the comparable body of knowledge taught were Materia Medica and included elements of pharmacology, therapeutics and pharmacy. 1.1 HISTORY The earliest written compilation of drugs was the Chinese herbal, the Pen Tsao, which was written by Emperor Shennung in about 2700 BC. A scientific basis for medicine was begun by Aristotle (384 - 322 BC), where after Theophratus (380 - 287 BC), a student of Aristotle, systematically classified medicinal plants on the basis of their individual characteristics, rather than their recommended use in treatment. This formed the basis of the first Materia Medica by Dioscorides, and established the framework for pharmacopoeias (officially recognized books of drug preparations). The first, pharmacopoeia was compiled by the German, Valerius Cordus (1514 - 1544). The nineteenth century marked the development of chemistry and the advent of detailed characterization and experimental study of active principles derived from natural sources. Claude Bernard (1813 - 1878) and James Blake (1814 - 1893) established the foundations of modern pharmacology. They outlined the dose response relationships. However, Oswald Schmiedeberg (1838 - 1921) from the University of Strasbourgh led the establishment of pharmacology as an independent scientific discipline. One of his students John J Abel (1857 - 1938) is regarded as the "Father of Pharmacology" in the USA. Abel established departments of pharmacology at the University of Michigan and later at John Hopkins University. He also founded the Journal of Pharmacology and Experimental Therapeutics and was instrumental in the formation of the American Society of Pharmacology and Experimental Therapeutics. 2 Development of veterinary pharmacology was the same as that for humans. Throughout much of medical history little distinction was made between human and animal medicine. However, near the beginning of the 20th century a gap developed between human and veterinary medicine, with veterinary medicine lagging behind. The teaching of Materia Medica as a didactic course persisted in veterinary schools until the early 1950s. This included elements of pharmacology, therapeutics and pharmacy. Little about pharmacology of drugs was known or taught in domestic animals. Meyer Jones, the original editor of Veterinary Pharmacology and Therapeutics, was instrumental in shifting the emphasis in the veterinary curriculum from Materia Medica to the science of Veterinary Pharmacology. (Abstract from Veterinary Pharmacology and Therapeutics: 6th Ed, Booth and McDonald) 1.2 SCOPE OF PHARMACOLOGY The scope of the subject is very broad and can conveniently be divided into four main components. (a) Fundamentals of pharmacology, including the history, source, composition and formulary drugs, principles of pharmacology, pharmacokinetics and pharmacodynamics. (b) Functional and Systemic pharmacology is the pharmacology of drugs used in the various organ systems. The systems chosen are: Autonomic nervous system Central and Peripheral nervous systems Trophic hormones and Endocrine system Cardiovascular, Blood and Haemopoeitic system Respiratory system Gastro-intestinal and hepatic systems Urinary system Musculoskeletal system Reproductive system Peripheral body systems (c) Chemotherapy is the branch of pharmacology dealing with drugs that selectively inhibit or destroy specific agents of diseases such as bacteria, viruses, fungi and other parasites. The classes of chemotherapeutics include: antibacterial, antiprotozoal, antifungal, anthelmintics, insecticides, antiviral and antiseptics. (d) Clinical pharmacology is concerned with the making of rational decisions about drug use in prophylaxis and therapy. 3 2. VETERINARY PHARMACEUTICS KNOWLEDGE REQUIRED 1. Define the following terms/concepts: a. Drug b. Formulation c. Dosage form d. Generic products 2. List the different ways in which a drug may be named and give an example of each. 3. List different sources of drugs. 4. Explain the constituents of a drug dosage form and explain the various purposes of the inactive ingredients. 5. List and explain the different types of dosage forms, including modified release formulations. 6. List the important veterinary drug delivery systems and consider advantages and disadvantages for each. 7. Briefly discuss the factors that affect the: a. rate of release of the active ingredient from a formulation b. safety and efficacy of generic drugs c. stability and shelf-life of a compound Veterinary Pharmaceutics include the preparation, compounding and dispensing of drugs in suitable forms for administration in animals. It is also concerned with the development of veterinary products for registration purposes. 2.1 DRUGS AND DRUG COMPOSITION A drug is any chemical substance affecting living protoplasm, which includes all medicines and preparations of internal and external use intended for the cure, mitigation, prevention or diagnosis of disease in either man or animals. 2.1.1 DRUG NAMES Chemical name - describes the chemical structure or composition of the substance. Generic name (International non-proprietary name) - each chemical compound is given an approved or official name which appears in the Pharmacopoeia. Trade (proprietary, brand) name - These are usually registered trademarks of the drug manufacturer. 4 2.1.2 DRUG CLASSIFICATION Chemical structure e.g. steroids Drug action e.g. demulcents Sources of drug e.g. natural, synthetic Type of compound e.g. atropine-like Function e.g. parasympathomimetic 2.1.3 SOURCES OR ORIGIN OF DRUGS Inorganic (mineral drugs), e.g. Fe2SO4 NaCl Vegetable, e.g. castor-oil, digitalis Animal, e.g. hormones (Insulin, Thyroid extracts, PMSG & HCG) Microbial, e.g. antibiotics (Penicillins, Tetracyclines & Aminoglycosides) Synthetic eg. antimicrobials (Sulphonamides & Nitrofurans) Recombinant technology, e.g. Bovine somatotropin & Insulin 2.1.4 DRUG COMPOSITION OR FORMULATION A formulation is the recipe or manner in which a drug is compounded. The purpose of a formulation is to find a suitable vehicle in which to dissolve/mix the active ingredients to achieve a suitable dosage form, as well as to control the rate of release of the active(s) to achieve the desired effect of the drug. Drugs seldom consist of a single ingredient but are more commonly composed of a mixture of active and inactive ingredients. A drug may consist of one or more active ingredient which is responsible for the drug effect. Although drugs may contain the same active ingredient, their activity may differ due to differences in the formulation such as differences in; Particle size Purity Chirality Salt / Ester Types of an inactive ingredient(s) or vehicles (commonly known as excipients) that may be included: Diluents Stabilizers Preservatives Dispersants Colouring agents The rate of release or availability of the active ingredient from a particular dosage form is a function of the composite properties of the dosage form, the active ingredient and how these interact with the physiological variables associated with the particular route of administration. By controlling the rate of release, using formulation variables, it is possible to profoundly modify: - onset of action - intensity and duration of action - and the incidence and intensity of side effects 5 Factors affecting the rate of release: (a) Composition (b) Manufacturing process (c) Physical and chemical properties of ingredients Modified release formulations Sustained release - drug formulation which results in prolonged release of an active ingredient relative to a conventional dosage form. Controlled release - drug formulation in which the release of the active ingredient is controlled or modified within specified limits. Long-acting - drug formulation resulting in a longer duration of action relative to the conventional dosage form. Residual - Residual action refers to a product with sustained action but is not necessary associated with formulation release characteristics. 2.1.5 GENERIC PRODUCTS A generic product is a drug copy of an original active ingredient, or also known as a non- proprietary active ingredient, of which the patent has expired. Generic products are either pharmaceutical equivalents, i.e. are products that contain the same amount of an active drug (i.e. the same salt, or ester or chemical form), but not necessarily the same inactive ingredients but in the same (or comparable) dosage form or pharmaceutical alternatives, i.e. products that contain the same therapeutic moiety, but may differ in its salt, ester, dosage form and strength. Counterfeit medicines are illegally copied medicines. Requirements for registration Registration of generic product in South Africa is based on evidence of chemical equivalence and bioequivalence relative to the originator or proprietary product. Chemically the active ingredient of the generic product must have the same physico-chemical characteristics (eg. pKa, particle size, crystalline form etc.) as the original product as described in an approved pharmacopoeia, normally the USP. Since the generic product does not necessary contain the same inactive ingredients as the original product comparable bioavailability, i.e. bioequivalence, with the proprietary product needs to be demonstrated. Bioequivalence Pharmaceutical equivalent or pharmacological alternative formulations are considered to be bioequivalent when the bioavailability of the test product differs only within certain limits, under identical experimental conditions and when administered at the same molar dose(s), compared to the reference product, normally the proprietor product. Bioequivalence testing includes comparative bioavailability or blood level studies; pharmacological end-point studies; and clinical end-point (or chemotherapeutic efficacy) studies. The bioequivalence test necessary will depend upon the type of product, pharmacokinetic characteristics of the product, correlation between indirect and direct test methods and analytical method available. Bioequivalence testing should be conducted using the most appropriate method available for the specific use of the product. 6 Therapeutic equivalence Products that contain the same active ingredient(s) or therapeutic ingredient(s) are therapeutic equivalent when they exhibit similar efficacy and safety profiles. Although clinically, thera- peutic equivalence may imply similar therapeutic effect, irrespective of the type of agent used (e.g. paracetamol or aspirin for analgesia) or the dosage regime it is only applicable to products containing the same active or therapeutic ingredient(s). Bioequivalent products are likely to exhibit therapeutic equivalence. Substitution policy Substitution refers to the replacement of a prescribed product, normally the proprietary product, by a cheaper counterpart, normally the generic product. No formal substitution policy exists in South Africa for veterinary medicines. A pharmacist only has the authority to suggest an alternative bioequivalent if a veterinary prescribes on the generic name. Care must always be taken when substituting a drug with a narrow safety margin, in therapies that require patient individualisation and stabilization and in the case of formulations with known bioavailability aberrations. In veterinary medicine adequate care must also be given to human safety when using products in production animals. 2.2 DOSAGE FORMS AND DELIVERY SYSTEMS 2.2.1 DOSAGE FORMS A drug dosage form is a preparation compounded in such a manner as to provide a convenient means of administering a drug to a patient. (a) Solutions: (Oplossing) Solutions are defined as homogeneous mixtures of two or more components which can be gaseous, liquid or solids. Solutions meant for parenteral administration have to be aqueous solutions. By definition solution do not precipitate when left standing. (b) Suspensions: (Suspensie) In this case the drug is insoluble in water. The most common examples are: * An emulsion which is an aqueous suspension of insoluble liquid substances with an emulsifying substance added to increase the stability of the preparation. * A mixture which consists of either soluble solid drugs, insoluble solid drugs or fluid drugs, or a combination of these suspended in the vehicle with/without a stabilizing agent. When left to stand, the active ingredient will precipitate out. These formulations are always marked with the words “Shake well before use”. (c) Solid dosage forms Powder: (Poeier) A powder may have varying granule sizes. It is used for oral dosing either directly, in feed or in drinking water. Powder for mixing in food should either be tasteless or incorporate flavouring and disguising agents suitable for the species for which they are intended. Capsule: (Kapsule) A capsule contains a powder or occasionally liquid and is made from hard gelatin. The gelatin disintegrates rapidly in the stomach. Capsule may also be made from plant cellulose is also available for 7 vegetarians/vegans. Tablet: (Tablet) A tablet is prepared from dry powders by pressure. Tablets may be coated with sugars, chocolate or gelatin to improve their flavour. Enteric- coated tablets are coated with inert substances such as keratin or shellac. These substances do not dissolve in acid gastric juice but will dissolve in the alkaline intestinal juices. Enteric coated tablets may not be broken prior to administration. Palatable tablet: These are special tablets formulated for dogs and cats. In addition to having preferred tastes, the product break down rapidly when in contact with moisture to provide a sticky substance that sticks to the mucous membranes. Pill: (Pil) A pill is the result of mixing powdered drugs with sticky substances such as honey or glucose. Pills are round and usually sugar-coated. They have gradually been replaced by tablets. Bolus: (Bal) A ball or bolus was used exclusively for dosing horses and consists of solid drugs mixed into a massing agent of glutinous consistency. Dragee: (Glasuurpil) A dragee is a sugar-coated pill or medicated confection. Lozenge: (Suigpil) A lozenge is a tablet which is allowed to dissolve in the mouth. Electuary: (Strooppasta) An electuary is a semi-solid sticky mass usually with a base of malt extract, honey or glycerine and which is administered by smearing the preparation on the tongue and teeth. Spansule: A spansule is a capsule which contains a mass of minute time-release granules to produce sustained drug action. Pellet: (Implantpil) A pellet is a compressed cylinder of insoluble crystalline steroids for subcutaneous implantation. (d) External dosage forms Lotion: (Losie) A lotion is a watery preparation for external application without friction. Cream: (Room) A cream is essentially a semi-solid emulsion which spreads easily when applied to the skin. Creams are usually miscible with water. Liniment: (Smeermiddel) A liniment or embrocation is a solution of an irritant substance in alcohol, a fixed oil or a soap and which is 8 applied to the skin by inunction or rubbing. Collodion: (Kollodium) A collodion is prepared form the solution of pyroxylin (cellulose) and colophony (resin) in alcohol and ether and which forms a solid protective film when applied to the skin. Ointment: (Salf) An ointment is a semi-solid viscous preparation of a drug which may be applied to the skin or mucous membranes. Oitments may be solutions or suspension. There are a number of ointment bases available e.g. lard, lanolin, soft paraffin, propylene glycol, polyoxyethylene derivatives etc. Paste: (Pasta) A paste is similar to an ointment but the consistency is much firmer due to the higher proportion of powder. Poultice: (Warmpap) A poultice or cataplasma is a sticky moist preparation applied externally to provide heat or counter-irritation. Suppository: (Steekpil,Setpil) A suppository is a solid drug form for insertion into a body cavity. They are inserted into the rectum, vagina and urethra and are made of a base that melts at body heat, such as oil of Theobroma or glycerinated gelatin. Pessary: (Skedetablet) Pessary is similar to a suppository but is prepared in much the same fashion as a tablet. Pessaries are deposited in the uterus or vagina and dissolve in fluids present. Enema (Enema) An enema is a fluid preparation for administration into the rectum. (e) Inhalation Aerosol: (Aerosol) An aerosol is a drug dosage form for administration by inhalation. The drug is administered in conjunction with a propellant. Nebulization: Nebulization is the administration of drugs dispersed as (Nebulisering) very small droplets of fluid. Fluids are dispersed into micro- particles by ultrasound. Vapourization: When a drug is administered in a vapour by mean of vaporization and heat e.g. steam. 9 2.2.2 DELIVERY SYSTEMS Delivery systems are devices designed to administer drugs accurately and with ease to the patient to ensure drug compliance, convenience and safety to the user. Various delivery systems available include: (a) Oral devices Drenching gun Paste dispensers Balling gun Rumen lodging devices (b) Parenteral devices Syringes (single or multiple dispensers) Implanting devices Remote projection eg. dart guns (c) Topical devices Pour-on, spot-on applicators Dust-bags Spray race & dips Aerosol dispensers Flea and tick collars Sustained release transdermal patches e.g. fentanyl patches 2.3 DRUG STABILITY The stability of a drug is an inherent characteristic of its chemical and physical properties. Most inorganic substances are very stable whereas organic substances are generally less stable. The two parameters that describe the stability of a product, which is determined before registration approval include: Shelf-life - the duration for which stability is claimed following date of manufacture. For some drugs this applies to the unbroached vial only Expiry date - the date at which the shelf-life expires and is indicated on the label The stability of a product is influenced by a number of environmental factors including:. - Temperature - Humidity - UV radiation (Photosensitivity) - Oxidation Adequate care in the handling of any product is important to ensure that it is kept under the most suitable conditions to maintain stability. The optimum storage conditions for each product, to ensure the shelf-life as claimed, are indicated on the label. Other external factors that may contribute to the maintenance of drug stability are: - Transport care - Suitability of drug container - Dispensing procedure - Mixing of drugs 10 2.4 DRUG DEVELOPMENT AND SUPPLY 2.4.1 DEVELOPMENT OF DRUGS Veterinary drugs are developed mainly by veterinary pharmaceutical companies, but also by other Research Institutes, such as Universities and Contract Research Organisations (CRO). The development involves the initial screening or design of a drug which thereafter undergoes various phases of development, including preclinical, clinical and pharmaceutical research. The purpose of this development is to generate sufficient supporting data to satisfy the requirements of either local or international regulatory authorities. 2.4.2 MANUFACTURE AND SUPPLY OF VETERINARY PRODUCTS Drugs are manufactured by either proprietary pharmaceutical companies such as Bayer, Boehringer Ingeleheim, Ceva, Elanco, Merial, MSD Animal Health, Virbac and Zoetis or by generic companies such as Cipla. Further there is compounding companies like Kyron, V-tech and Wildlife Pharmaceuticals. Veterinary Products in South Africa are distributed and through a number of channels, including the veterinarian, pharmacist, veterinary supplier, Co-ops and retail shops. The types of products that may be distributed or supplied through the various channels and the requirements for each are described under the various Acts (see regulatory section). 2.4.3 REGISTRATION OF VETERINARY PRODUCTS All drugs sold for use in animals have to be registered in South Africa. Registration occurs in terms of two Acts: viz. Act 36/47 for Stock Remedies and Act 101/65 for Veterinary Medicines (see requirements for registration and control under the regulatory section). Approval for registration in terms of both Acts is based on the evaluation of safety, efficacy and quality of the product. 2.4.4 QUALITY CONTROL The quality of veterinary products manufactured and supplied in South Africa are controlled in terms of the Act under which the product is registered. Control procedures include: Good Manufacturing Procedures (GMP) Inspection procedures Laboratory testing 11 3. PHARMACOKINETICS KNOWLEDGE REQUIRED 1. Define the following pharmacokinetic terms/concepts and where applicable – Explain the processes involved Give the prerequisites for a drug to undergo this pharmacological process Discuss the pharmacological significance Discuss the various factors that affect each process o Absorption o Bioavailability o Biophase o Biological elimination half-life o Biotransformation o Diffusion barrier o Disposition o Dissolution o Distribution o Elimination o Enterohepatic circulation o Enzyme induction o Enzyme inhibition o First pass effect o First-order kinetics o Ion-trapping o Plasma protein binding o Pre-systemic elimination o Volume of distribution o Zero-order kinetics 2. Explain the differences between absorption, adsorption and penetration in terms of drug movement. 3. Draw an annotated graph illustrating the translocation process of a compound following administration. 12 4. Discuss the different processes by which drug molecules can cross cell membranes and the types of diffusion barriers in the mammalian body. 5. List the organs, in order of importance, which are responsible for drug excretion and briefly explain the processes. 3.1 TRANSLOCATION PROCESSES There are several events that constitute the uptake and movement of a drug within the body, including access to the site of action or biophase and removal of drugs from the body as indicated in Fig. 1. These are known as the translocation processes. After a drug has been administered it is released from its formulation and absorbed in solution from the different sites of application e.g. oral, intramuscular, subcutaneous, etc., and enters the blood stream. In the bloodstream some of the drug molecules may bind reversibly to various blood constituents e.g. plasma proteins while the remainder will undergo simultaneous distribution (i.e. movement out of the blood stream to various tissues including to the site of action) and elimination (i.e. both biotransformation and excretion processes). Drug disposition describes the simultaneous effects of distribution and elimination. Usually distribution is a much more rapid process than elimination. The fate of a compound refers to the pathways of biotransformation and routes of excretion. Biotransformation is the metabolic alteration of the structure of a drug and is a prerequisite for excretion of many drugs. The aim of administering a drug is to achieve a desired clinical response (therapeutic effect). Dose rate, formulation, route of administration and bioavailability determine the amount of 13 drug reaching the bloodstream (central compartment). The duration of action depends upon the relative rates of the various translocation processes and drug metabolic pathways. Absorption and distribution processes influence the drug concentration reached in the immediate vicinity, or the site of drug action (biophase), whereas biotransformation (i.e. metabolism) and excretion terminate the action of a drug in the body. The latent period of drug response is the period between the time of administration of the drug and onset of its effects. Pharmacokinetics explores what the body does to drugs by defining the qualitative, quantitative and temporal effects of the various translocation processes. 3.2 MOVEMENT OF DRUGS ACROSS BIOLOGICAL MEMBRANES All the translocation processes of drugs, including access to the site of drug action and either directly or indirectly, involve the movement of drugs across biological membranes. 3.2.1 Cellular membranes Review histology and physiology and composition of cellular membranes (Fig1). Figure 1: The fluid mosaic model of membrane structure. The membrane consists of a bi-lipid layer with proteins inserted in it or bound to the cytoplasmic surface. Those proteins that completely span the bilayer are called transmembrane proteins 3.2.2 Transmembrane movement of drugs Transmembrane movement of drug molecules occur either across the cell membrane (Fig. 2) or through gaps between cells (Fig. 3). 14 Fig. 2: Transmembrane movement of drugs Most free unbound molecules can move freely between cells. However, movement across a cellular membrane can only occur if the molecule is in a non-ionized or lipid soluble state, unless actively transported. The normal cell membrane is charged and repels ionised molecules. Drug ions are also usually too large to move via the aqueous channels in cell membranes. Fig. 3: Pores in cell membrane The processes by which ionized and non-ionized molecules move between body fluid compartments and through cell membranes include: (a) Passive processes Simple diffusion Filtration/bulkflow 15 (b) Specialized processes Facilitated diffusion Active transport Exchange diffusion Phagocytosis and Pinocytosis (Review physiology for description and requirements of these processes) 3.2.3 pH/pKa diffusion hypothesis and Henderson-Hasselbalch equation Most drugs are weak acids or weak bases and therefore exist at body pH partly in ionized and partly in the non-ionized state. The degree of ionization is dependent on the pKa of the drug and pH of the environment, or aqueous phase, in which the drug is dissolved. The relative concentration of non-ionized to ionized drug can be calculated using the Henderson-Hasselbach equation as follows for acidic (a) and basic (b) drugs, respectively: (a) pKa - pH = log [non-ionized] [ionized] (b) pKa - pH = log [ionized] [non-ionized] As a general rule acidic drugs, at a lower pH will be mainly in a non-ionized state and at a higher pH in an ionized state, whereas basic drugs will be mainly in an ionic state at a lower pH and in a non-ionized state at a higher pH. Ion-trapping is a consequence of pH differences between various body fluids. At diffusion equilibrium, as result of the pH-pKa partitioning effect, an unequal concentration of drug molecules will occur on either side of the cell membrane, with higher concentrations on the side where the degree of ionisation is greater. To understand this principle, refer to the diagram below. In the diagram non-ionized molecules are able to cross the lipid membrane by the process of simple diffusion i.e. area of high to low concentration. Once it enters the plasma the difference in pH results in the ionisation of the molecule. Since the ionized molecules cannot cross the membrane they accumulate on this side of the membrane. Secondly the ionised molecules do not change the concentration gradient. As such a large concentration of drug may collect selectively on one side of the membrane. 16 Ion-Trapping (Weak acid of pKa 4.4) Lipioid mucosal membrane Plasma Gastric Juice pH 7.4 pH 1.4 [U] = 1 1= [U] [I] = 1000 0.001 = [I] Total = 1001 1.001 = Total Weak acid [I] = [U]*10(pH-pKa) 3.2.4 Diffusion barriers Various intercellular diffusion barriers exist which restrict readily movement of drug molecules between cells. Important diffusion barriers which should be considered include: Blood-Gastrointestinal barrier Blood-Brain-barrier Blood Cerebro-spinal fluid barrier Blood-udder barrier Blood-testes and Blood-prostate barrier Blood-bronchus In general non-ionized, lipid soluble drug molecules can only pass these barriers. In cases of inflammation of the barrier, the barrier becomes disrupted and allows both lipid and water soluble drugs to cross the membrane. Once the barrier heals it will once again serve as a barrier to water soluble drugs. 3.3 DRUG ABSORPTION Drug absorption is the passage of a drug from the site of application or administration into the circulation or central compartment (The central compartment in considered the central blood supply that enters the aorta). Drugs that are given intravenously do not need to undergo the process of absorption. Drugs administered by any other route will have to undergo the process of absorption if it is to enter the central compartment. Penetration of the skin or gastrointestinal epithelium, without entering the central circulation, does not constitute absorption. 3.3.1 Prerequisites for absorption The following prerequisites are necessary for absorption to occur i.e. if these criteria are not met drugs will never be absorbed: (a) Release from dosage form Dissolution of the dosage form must occur for release of the active ingredient. Factors that affect the rate and extent of dissolution are described under the 17 Veterinary Pharmaceutical Section. Disintegration of solid dosage forms must occur before dissolution can take place. The physiological fluid in the body is water. As such for a drug to go into dissolution in the body it must dissolve in water. In other words purely lipid soluble drugs can never be absorbed. (b) Transmembrane movement Drug molecules must be able to move from the site of administration into the circulation. Gastrointestinal absorption of polar, water soluble drugs are generally restricted due to the presence of the gastrointestinal diffusion barrier. The keratin layer of the skin similarly serves as a barrier for percutaneous absorption i.e. for a drug to cross a barrier it must have a component of lipid solubility. If we are to consider the first condition for absorption this would imply that only amphoteric molecules may be absorbed from the gastrointestinal tract or when applied topically onto the skin i.e. the molecule needs to be sufficiently water soluble to undergo dissolution and sufficiently lipid soluble to pass through the epithelial membrane. For all other routes of administration, which bypass these barriers, drug needs only to be water soluble to enable it to go into solution e.g. a subcutaneous administered drug bypasses the keratin barrier in the skin and enters directly into the subcutaneous layer. From here the drug must go into solution to be absorbed. (c) Blood supply Adequate blood supply at the site of administration is necessary for absorption. 3.3.2 Factors affecting absorption (a) Physicochemical Crystalline size and shape Lipid solubility - required for gastro-intestinal and percutaneous absorption Particle size - micro ionized forms of mebendazole, nicloscanate and griseofulvin achieve better dissolution and therefore better absorbed Salt - the solubility of a drug may be influenced by the type of salt e.g. penicillin is released slower from the procaine salt. Erythromycin stearate is also more pH stable. (b) Pharmaceutical Absorption of a drug from the gastro-intestinal tract involves the release of the drug from its dosage form. For a solid dosage form it involves disintegration and dissolution. Occasionally dissolution, especially of sparingly soluble drugs, is so protracted that it controls not only the absorption process but also the overall rate of elimination (flip- flop pharmacokinetics). Here out drug is sparingly water soluble. Since dissolution is a pre-requisite for absorption, the slow rate at which this drug dissolves will result in a slow rate of absorption. Dissolution can be enhanced by the salt form of the drug or by decreasing the particle size (eg. griseofulvin). These salts are added onto the molecule by the pharmaceutical companies. Here the aim is to create an amphoteric molecule i.e. to allow both dissolution and translocation to occur. (N.B. Once the molecule dissolves, the salt is released and our molecule will revert to its original solubility inside the body.) 18 Drug absorption from a suspension is usually more rapid than from a tablet. Dosage form and manufacturing process will affect the rate and extent of dissolution. (c) Physiological (Intra-animal variables) (i) pH of gastro-intestinal contents. Differences in pH results in variable rates of absorption from the different portions of the gastro-intestinal tract e.g. acidic drugs are more rapidly absorbed from the stomach in comparison to the small intestine due to a greater portion of non- ionized, lipid soluble molecules. pH of the gastro-intestinal contents can be influenced by a number of factors such as diet, stress, species etc. (ii) Rate of gastric emptying. The largest portion of absorption of most drugs occurs in the small intestine due to the greater absorptive surface. An increase in the rate of gastric emptying will therefore generally increase the rate of absorption of drugs. Gastric emptying may be increased by the pro-kinetic drugs such as metoclopramide. (iii) Intestinal motility. Motility will influence both the rate of transport and mixing process and will determine the contact time for absorption. Gastro-intestinal absorption may therefore be delayed by both diarrhoea and constipation, respectively. (iv) Gastrointestinal (GIT) perfusion. In fear, stress etc. the blood to the GIT is reduced which may reduce absorption of drug. Although in theory the general systemic increase in blood supply during stress usually increases the rate of absorption from other sites this is not the case in the GIT. (v) Effect of food. - Chelation i.e. the binding of drugs to Ca++, Mg++, Al+++ etc. will reduce both the availability of these ions and the drug for absorption e.g. chelation of Ca++ and Mg++ to tetracyclines. Chelated drugs are too large to be absorbed. Chelates are also inactive. - Non-specific binding (NSB) between food and drugs may reduce the absorption of drugs e.g. ampicillin. - increased absorption of drugs may also occur in the presence of food e.g. griseofulvin absorption is promoted by fat in the gut. (vi) Presence of micro-organisms. Some drugs are broken down in the rumen e.g. chloramphenicol. (vii) First-pass effect (pre-systemic elimination). During the process of absorption drug molecules are exposed to enzymes in the intestinal mucosa, and molecules absorbed from the stomach and small intestine are conveyed in hepatic portal blood to the liver before reaching the general circulation. Biotransformation of a drug by enzymes in the gut mucosa or the liver or both, before reaching the 19 circulation (central compartment) is known as the first-pass effect or pre-systemic elimination and may significantly reduce the bioavailability of orally administered drugs. Examples of drugs which undergo significant pre-systemic elimination are: Propranolol (ß adrenergic blocker). Morphine (opioid analgesic). Lignocaine (local anaesthetic). Nitroglycerine (vasodilator). (d) Animal (Inter-animal variables) A number of animal factors may influence the rate and extent of absorption of drugs from the various routes of administration (Please note that these factors are not the same as physiological factors) e.g.: Species and Breed Age and body mass Sex Presence of disease (e) Drug administration May be influenced by: Route and site of administration Dose volume Rate of administration Concurrent therapy 3.4 DISTRIBUTION Drug distribution is the dissemination of a drug within the body, involving the transport of a drug via the bloodstream to the various parts of the body and the movement from the circulation (central compartment) into the tissue or other body fluids (peripheral compartment). The relative rates of distribution and elimination (biotransformation and excretion) which proceed simultaneously, once the drug enters the blood stream determines the concentration of unchanged drug attained at the site of action (biophase) or otherwise referred to as biophasic availability. The extent (or magnitude) of distribution largely determines the amount of drug which must be administered to produce a concentration within a therapeutic range of plasma levels. Only drug molecules which are present in the water phase of blood plasma (i.e. the unbound or free drug) are available to enter the peripheral compartment at a rate determined by the blood flow to the various organs, the ability of the molecules to penetrate the capillary endothelium and diffusion into the intracellular fluid. Bound drugs are too large to leave the blood vessels. In general a dynamic equilibrium becomes established very rapidly between the blood/lymph and tissues or body fluids. However, differences in regional perfusion may account for variations in the time required for drug concentrations in various tissues to equilibrate with blood concentration or concentration in the central compartment. The movement of drugs from the central compartment to the peripheral compartment involves the penetration of drug molecules through the capillary endothelium into the extracellular fluid and the diffusion of the 20 drug molecules across all types of membranes into intracellular or transcellular (eg. CSF) fluids. Capillaries, except in the brain, are quite permeable to drugs, compared to cell membranes. All drug molecules of small or intermediate molecular mass (size) that are free in the circulation gain access to the extracellular interstitial spaces of most tissues in rather a short time. Most drugs are not distributed equally throughout the body but tend to become sequestered at particular sites e.g. highly lipophilic substances such as DDT in fat, tetracyclines and certain heavy metals in bone etc. Transcapillary movement may thus occur either between endothelial cells, for which molecular size is the major determinant, or move across the capillary endothelium, which is determined by the degree of lipid solubility. An initial phase of distribution may be distinguished which reflects cardiac output and regional perfusion. Differences in the equilibration rates between various tissues e.g. between well perfused areas such as the CNS, heart, kidney and liver as compared to adipose tissue and other tissue with poorer blood supply may, kinetically speaking, divide the body into different peripheral compartments. The intensity and duration of pharmacological effect is related to extent (magnitude) and pattern of distribution. Any factor therefore, which may affect either the extent or pattern of distribution of a drug may affect its pharmacological effect. 3.4.1 Physicochemical factors affecting drug distribution (a) Lipid solubility. Lipid soluble drugs are normally widely distributed as they can accumulate in the body’s fat: cell membranes and fat stores. (b) Dissociation constant (pKa). In general basic drugs tend to accumulate in regions of low pH in the body and conversely acidic drugs tend to accumulate in regions of high pH (refer to ion trapping.) (c) Molecular mass and shape. Usually not a limiting factor since most drugs are small and the blood vessels in the body are very permeable due to the presence of large intercellular gaps. It is only when internal barriers are present, like the blood-brain-barrier, that the size and charge of the molecule has an influence on its distribution. (d) Chirality. Many drugs occur as racemic mixtures i.e. are mixtures of stereo- isomers. Stereo-specific distribution has recently been observed with various drugs e.g. ketoprofen and albendazole and may vary between different animals species. 3.4.2 Physiological factors influencing distribution (a) Regional perfusion. Since tissues of the central nervous system and heart are well perfused, higher concentrations of highly lipid soluble substances, e.g. thiopentone will initially be found in these tissues relative to less well perfused tissues e.g. adipose tissue as they get here first. Differences in blood flow rates within an organ may reflect differences in distribution pattern within the organ. 21 (b) Tissue mass or size relative to body mass. (c) pH of body fluids. Although large pH differences exist between body compartment e.g. plasma (7,4) and gastric juice (1,4) it is more common to contend with rather small pH differences e.g. intracellular fluid (7,0) and extracellular fluid (7,4). (d) Capillary permeability. Capillaries in the various organ systems display a wide variation in their permeability. Factors which may affect the capillary permeability may therefore also affect the distribution pattern of drugs e.g. blood brain barrier is not effective in cases of encephalitis. (e) Body fluid compartments. Body water constitutes 45 - 75% of body mass. Differences in the size of the various fluid compartments is responsible for differences in the distribution pattern e.g. lipid soluble drugs will not be able to dissolve in these aqueous compartments and as such will not be well distributed to these areas. (f) Active transport. A drug which is transported across a membrane by a specialized transport process will become unequally distributed across the membrane. Cells capable of this function include renal tubular epithelium, hepatocytes and gastro-intestinal epithelium. (g) Macromecular binding. Drugs often become bound to various macro- molecular components. While in this bound state, a drug cannot freely cross a body barrier and is thus usually pharmacologically inactive although it does remain in dynamic equilibrium with unbound drug. This binding is usually reversible. Drug molecules may become attached to protein or they may form non- diffusable compounds, with other substances. They may also become attached to specific binding sites in particular tissues. The degree of binding may vary considerably between different drugs and between different tissues, respectively. This situation often leads to an unequal drug distribution between the tissues and fluid compartments. In circulating blood, drugs may be associated with different blood constituents including albumin, globulins, lipoproteins and erythrocytes. Albumin binding is the most important for acidic drug and may significantly influence the distribution and rate of drugs when extensively (> 80%) bound. Basic drugs are bound mainly to α- glycoproteins. Generally it appears that acidic drugs are more highly bound than basic drugs. The interaction of a drug with a binding site on albumin may be considered a reversible reaction obeying the law of mass action. [Free drug] + [Albumin] [Drug albumin complex] Since the binding interaction is readily reversible the drug-albumin complex serves as a circulating drug reservoir which provide free drug as the concentration in plasma declines by elimination (biotransformation and excretion) processes. Examples of drugs which are highly plasma bound: Nitrophenol Halogenated salicylanalides Doxycycline etc. 22 Plasma protein binding may be influenced by the following factors (i) pH - normally constant at 7,4. Certain of the NSAIDs such as flunixin are 99% plasma protein bound. When the pH of the plasma drops the drug is released and free to enter the tissues to have an effect. As such we can cause severe side effects in animals as more free drug is available in the system. (ii) Competition between similar molecules e.g. warfarin is 97% bound and only 3% is free and available for pharmacological effect (anti- coagulant). Phenylbutazone, may compete with warfarin so that less warfarin is bound and more becomes free. (iii) Slight structural modification of a molecule may significantly alter the extent of the drug-albumin interaction. With a change in the molecular shape of the protein it may longer be able to bind to the drug (iv) Disease conditions e.g. uraemia and hypoproteinaemia. In uraemia we have a large amount of circulating toxin in the body. This can alter the shape of the albumin molecule. With hypoproteinaemia there is less protein available to bind to the drug (v) Distribution barriers 3.4.3 Redistribution of drugs This is the movement or distribution of a drug molecule from one peripheral compartment via the central compartment to another peripheral compartment. It is affected by the relative perfusion rates and mass of tissue e.g. the recovery of an animal from thiopentone anaesthesia is as result of the redistribution of the drug from the well perfused areas of the body, in particular the CNS, to the less perfused areas e.g. muscle and fat. 3.4.4 Consequences of uneven drug distribution Uneven drug distribution pattern due to mechanism as previously described have an influence on: Tissue residue and withdrawal periods e.g. DDT Drug dosage e.g. thiopentone bolus Disfigurement e.g. yellow staining of teeth by nitrofurantion Forensic value e.g. arsenic and heavy metals in hair 3.5 DRUG ELIMINATION Drug elimination refers to all processes that are responsible for the reduction of drug concentration in the body fluids. Hepatic metabolism and renal excretion are the principle mechanisms by which drugs are eliminated. Less important mechanisms include biliary and salivary excretion, loss in milk, sweat and via lungs. The efficiency of drug elimination is mostly determined by the accessibility of the drug to the sites of elimination. Consequently, the magnitude of the dosage and pattern of distribution can control the persistence of a drug in the body. A drug may be eliminated by more than one route simultaneously. 23 3.5.1 Biotransformation Biotransformation or drug metabolism is the metabolic transformation and/or synthesis of a drug to make the drug more accessible for excretion. In general drugs that are water soluble and polar are not metabolized and are excreted unchanged. Lipid soluble, non-polar compounds are generally biochemically altered to more polar metabolites and then excreted. The principle aim of biotransformation is therefore to change a drug to a water soluble substance so that it can be excreted by one or other of the several routes available. The biotransformation reactions are divided into 2 phases. Phase 1 reactions are the metabolic transformation by oxidation, reduction and hydrolysis to introduce into the drug molecule groups such as OH, C00H and NH2. The second phase consists of a synthetic reaction in which conjugates are formed with endogenous substances such as acetate, glycine, sulphate or glucuronic acid. Conjugated products are water soluble and are invariably inactive. Phase 1 Phase 2 Transformation Synthetic reaction Drug Intermediate metabolite Conjugated product (water soluble) If the drug already contains a suitable chemical group it can undergo conjugation directly, without the necessity of a phase 1 reaction. (a) Metabolic (Phase 1) reactions Phase 1 metabolic reactions are carried out by enzymes that occur mainly in the liver, although some metabolism can also occur in certain extrahepatic tissues such as the intestine, kidney, lung and blood plasma. Some metabolic reactions of drugs are carried out by the gut microflora, and certain drugs undergo spontaneous reactions under appropriate physical conditions, such as pH. Several enzymatic systems may be involved in the transformation reactions of which the microsomal mixed function oxidases are the most important. Liver microsomes are segments of hepatic endoplasmic reticulum and are obtained by ultracentrifugation of liver homogenate. Mixed function oxidases require oxygen, NADPH and cytochrome P450. Some oxidation and reduction reactions are mediated by enzymes present in the soluble and mitochondrial fractions of the liver, plasma, kidneys, placenta, intestinal 24 mucosa and gut flora. (b) Synthetic (Phase 2) reactions Synthetic reactions occur mainly in the liver but also in other tissues, particularly the kidney. These reactions occur when the drug or phase 1 metabolite contains a suitable chemical group (-OH, -COOH, -NH2 or -SH) which can be combined with a conjugating agent (glucuronic acid, glycine, methionine, sulphate, acetic acid and thiosulphate). In most cases drugs are inactivated during phase 2. Occasionally an active drug is incorporated into a normal body biochemical process which becomes disrupted. This is described as lethal synthesis and leads to intoxication eg. fluoroacetate. (Please refer to toxicology) (c) Changes in drug activity following metabolic transformation During metabolic transformation drug activity may be changed in the following ways: (i) Inactivated e.g. pentobarbitone, procaine, chloramphenicol etc. (ii) Converted to a different activity which may more or less active than the original drug e.g.: Propranolol to 4-OH propranolol Digitoxin to digoxin Phenylbutazone to oxyphenbutazone Primidone to phenobarbitone (iii) Activated during which an inactive drug is converted to an active metabolite. Drugs which become activated are known as prodrugs e.g.: Chloralhydrate to trichloroethanol Parathion to paroxon Febantel to fenbendazole (d) Rate of drug metabolism While most metabolic reactions have adequate capacity (i.e. first order reactions) at the usual tissue or body fluid concentrations of the drug obtained after administration of a therapeutic dose, some metabolic pathways have a limited capacity (i.e. zero order reactions) above a certain concentration of substrate. In the case of zero order reactions the removal of the drug from the body is disproportionately prolonged with increasing dose. Variations in the velocities of phase 1 metabolic reactions and in hepatic blood flow contribute significantly to species differences in drug biotransformation. Marked unpredictable interspecies differences in the rate and degree of phase 1 reactions occur e.g. lignocaine. Variation in conjugation reactions also exist among species with certain reactions either absent or deficient in a particular species e.g. glucuronyl transferase deficiency in cats, absence of acetylation in dogs and a low level of sulphate conjugation in pigs. 25 A drug can be a suitable substrate for more than one enzyme and for this reason may give rise to several metabolites, each of these which can undergo further reactions. The sum total of these reactions constitutes the drug's degradation pathway. Thus even if an enzyme pathway is deficient in an animal species the body will still be able to metabolise the drug. These secondary pathways are however, usually slower and less efficient. (e) Factors affecting biotransformation Enzyme induction. Certain drugs have the ability to increase the amount of microsomal enzymes in the liver after repeat treatment. Associated with the increase in amount of microsomal enzymes is an increase in liver mass and size of endoplasmic reticulum. Increased activity or induction of liver microsomal enzymes lead to an accelerated biotransformation and alteration (normally a decrease) in the intensity and duration of pharmacological response of the inducing substrate or of a other concurrently administered drug which also can serve as a substrate for the same biotransformation reaction. A wide variety of drugs are microsomal inducers e.g. the antiepileptic drugs (phenobarbitone, diphenylhydantoin and primidone), most of the chlorinated hydrocarbons (DDT), phenylbutazone, griseofulvin etc.. Any drug that is lipid soluble at physiological pH should be considered being capable of inducing microsomal enzymes. The physiological significance of enzyme induction is the development of tolerance and the reduction of therapeutic effect unless the dose rate is increased or given more frequently e.g. phenobarbitone. Enzyme induction develops only after continued use of a drug over a prolonged period (weeks) and in many cases it may take 3 - 4 months for microsomal enzyme activity to return to pretreatment activity after discontinuation of treatment. Enzyme inhibition. If drug metabolizing systems, in particular the liver microsomal enzyme, are depressed or inhibited a prolonged response may occur to ordinary doses of drugs. The exception will be for those drugs which require activation to be either effective or toxic. In this case the reduction in enzyme activity will reduce the effect of the drug. Drugs that inhibit microsomal enzyme activity in animals include: organophosphor insecticides, quinidine, carbon tetrachloride, erythromycin and chloramphenicol. Inhibition of drug metabolizing enzymes may also impair the metabolism of endogenous steroids. Some drugs inhibit non-microsomal enzymes that are responsible for the inactivation of other drugs and some endogenous substances (e.g. catecholamines). Plasma pseudocholinesterases is also irreversibly inactivated by organophosphors. Species differences. Difference in the degradation pathways and deficiency in conjugation reactions occur amongst different species that account for differences in the pharmacokinetics and pharmacological response or toxicity of drugs in these species e.g. paracetamol toxicity in cats due its deficiency in the glucoronyl transferase enzyme. Age. Drug metabolizing microsomal enzymes are either absent or present in negligible amounts in the immature liver of a newborn animal. Thus the neonate is more sensitive to drugs than is the adult of any species. Most domestic mammal species require 3 26 months for metabolic functioning to reach its maximum physiological levels. Sex. Difference in the rate of drug metabolism have been demonstrated in a few instance between sexes. The hexobarbitone sleeping time in certain strains of rats and mice is very much longer in the females than in the males. Physiological conditions. During pregnancy the activity, especially of conjugating reactions decrease. The endocrine status of an animal may influence drug metabolism to some degree. Nutritional status. The availability of adequate liver glycogen, protein for enzyme synthesis and vitamins and minerals as biochemical cofactors, is essential for optimum biotransformation. Liver disease. Depression of the microsomal drug metabolizing system and other biotransformation enzymes can be expected in pathological states where there is a deleterious effect on the liver function. Examples of such conditions include hepatitis, liver cirrhosis, hepatocellular damage due to poisons and toxins etc. 3.5.2 Drug excretion The kidney is the principle organ of excretion but the liver, gastro-intestinal tract and lungs may also play important roles in some cases. Milk, saliva and sweat are in most instances of lesser importance, although the presence of an active principle in milk may produce an effect on the suckling young and be responsible for residues which may be harmful for human consumption. Excretory organs can be arranged in the following decreasing order of importance 1. Kidney and urine 2. Liver and bile 3. GI-tract (ie. through the gut wall and excluding bile) 4. Lung (important for volatile anaesthetics) 5. Milk 6. Saliva 7. Sweat 8. Skin, hair and hooves (a) Renal excretion Renal excretion of drugs involves glomerular filtration, tubular secretion and tubular reabsorption. (i) Glomerular filtration. All unbound molecules (including lipid soluble molecules), smaller than plasma albumin, will be readily filtered through the porous glomerular capillary membrane into the tubular lumen. The amount of drug filtered through the glomerulus is dependent on the degree of plasma protein binding and glomerular filtration rate. 27 Renal clearance of drugs can be markedly influenced by changes in glomerular filtration rate eg. with renal failure or with pharmacological substances that lowers blood pressure or constricts renal arterioles. (ii) Tubular secretion. Tubular secretion of both acidic and basic drugs takes place mainly at the proximal end of the proximal convoluted tubule by carrier mediated transport processes. The active transport mechanisms are relatively non-specific with independent processes for organic acids and for organic bases. These systems are saturable but are in most instances not saturated. Competitive inhibition by agents with similar molecules can occur eg. penicillins and probenecid. Active transport is also energy dependent. Plasma protein binding does not usually hinder tubular excretion of drugs because of the dynamic equilibrium which exists between free and bound drug. As free drug is removed and transported across the tubular epithelium, immediate dissociation of the drug-albumin complex occurs. Passive tubular secretion is not very important. Lipid soluble molecules in the peritubular capillaries can be transferred into the tubular fluid and be excreted in urine. This occurs very seldom and is dependent on pH / pKa relationships, concentration gradients and lipid solubility of the drug. (iii) Tubular reabsorption. The passive reabsorption of drug molecules from the renal tubule plays an important role in the overall rate of renal drug excretion. Reabsorption can occur anywhere along the tubule although it occurs mainly in the proximal convoluted tubule due to the larger surface area. Whether a drug is reabsorbed or not will depend on the factors which govern the transmembrane passage of molecules including: Lipid solubility. pH / pKa relationship. Highly ionized or polar agents will not be reabsorbed and the degree of ionization will depend on the pKa of the compound, whether it is an acid or a base and the pH of the glomerular filtrate and urine. Acidification or alkalinization of the urine may potentially alter the rate of excretion of some unchanged drugs. Species differences due to differences in urinary pH may also affect tubular reabsorption e.g. herbivores under normal conditions have an alkaline urine and carnivores an acidic urine. Please note that only lipid soluble non- ionised drugs will be absorbed passively as the renal tubular epithelium forms a natural barrier Active tubular reabsorption is not very important in the case of drugs. 28 (b) Liver and biliary excretion Although the principle route of excretion of most drugs and their metabolites is through the kidney some compounds are excreted partly or mainly through the liver into bile. Biliary excretion of compounds may take place by passive diffusion or active transport of drug molecules from hepatic sinusoids through the parenchymal cells into the bile. Compounds excreted in bile are conveyed ultimately into the small intestine and excreted in the faeces. Glucuronide conjugates, both of drugs and endogenous substances (e.g. bilirubin) may undergo an enterohepatic circulation, whereby the parent compound may be liberated by hydrolysis from the conjugate and be reabsorbed from the intestine. The pharmacological significance of enterohepatic circulation depends on the fraction of the dose excreted in bile. If this is substantial e.g. digitoxin, the effects of a single dose of the drug will be prolonged. (c) Excretion of drugs into the intestinal tract (excluding biliary excretion) The factors which determine the absorption and distribution of drugs in the body are also concerned in the excretion of drugs and metabolites in the faeces. The important considerations are the relative proportion of lipid soluble molecules in the plasma and gut and active transport into the gut. Very lipid soluble drugs like doxycycline, which undergoes minimal metabolism, are excreted directly across the intestinal wall. Considerable variation in this route of excretion between various compounds may occur. Furthermore, the anatomical and functional differences in the digestive tract of domesticated animal species will also lead to species differences. (d) Pulmonary excretion Gaseous and volatile anaesthetics are eliminated from the body in the same way as they are absorbed; by diffusion through the alveolar epithelium in accordance with their concentration gradients. For other drugs this route is insignificant. (e) Drug excretion in milk Important only in lactating animals. The epithelium of the mammary gland behaves as a lipoid membrane separating blood of pH 7,4 from milk of a somewhat lower value (pH 6,6). When drugs are administered to lactating animals, basic compounds appear in the milk in a concentration greater than that in plasma and acidic drugs in a concentration lower than that in plasma. Completely non-ionized substances like ethanol and urea distribute evenly between milk and plasma. (f) Drug excretion in saliva Transfer of drugs from the circulation into saliva is determined by the molecular size, lipid solubility and degree of ionization of the compound. Large species differences occur in the amount and pH of saliva excreted. (g) Drug excretion in sweat Drugs may be excreted in sweat by passive transfer of the non-ionized molecules but 29 this route of drug excretion is of little importance. (h) Factors affecting the overall excretion rate of drugs (i) Renal disease. Renal ischemia, glomerular involvement, tubular damage, impaired intrarenal perfusion, functional disabilities of the tubular cells and obstructive lesions in the tubules, collecting ducts or even the ureters or urethra, may all influence the renal clearance of drugs. Changes in the pH of the filtrate will also alter the excretion rates of drugs with appropriate pKa values. The excretion of unchanged, lipid insoluble, polar drugs, which rely mainly on renal excretion, e.g. aminoglycosides are, significantly affected by renal failure. For example streptomycin normally has as T1/2ß of 2 hours. With renal failure the T1/2ß can be increased to as much as 24 hours. (ii) Reduction of glomerular filtration rate. Any pharmacological active compound that lowers blood pressure or constricts renal arterioles will reduce the glomerular filtration rate (GFR). The effect of reduced GFR on renal excretion of a drug which is "secreted" by the renal tubules will be considerably less than that for drugs excreted by filtration alone. Under conditions of severe exercise, shock etc. GFR may also be decreased or inhibited. (iii) Liver disease. Although of less clinical importance impairment of the excretory functions of the hepatocyte or obstruction of bile flow due to any cause will interfere with the biliary excretion of drugs. The enterohepatic cycle of a drug may also be affected by liver disease or may be modified by disruption or elimination of the intestinal flora. (iv) Drug and nutritional interactions (v) Physical condition of the animal 3.6 PHARMACOKINETIC DESCRIPTION AND QUANTIFICATION 3.6.1 Assumptions about biological fluid and tissue concentration, response and time The intensity of drug response and duration of drug effect is influenced by the concentration and length of time a drug is present in the biophase. Unfortunately it is not practical to monitor drug concentration versus time at the site of action for control of drug response. However, since the concentration and time frame of a drug in the biophase is in equilibrium with that found in body fluids elsewhere it is possible to predict changes in drug concentration at the site of action by measuring blood concentrations. 30 3.6.2 Pharmacokinetic parameters (a) Bioavailability Bioavailability is defined as the rate and extent of drug which enters the central compartment unchanged and is therefore a measurement of absorption. The main pharmacokinetic parameters used to determine bioavailability of a drug is area under the drug blood concentration versus time curve (AUC), maximum drug blood concentration (Cmax) and time to Cmax (Tmax). AUC represents the extent of drug absorption whereas Cmax and Tmax are measurements of rate of absorption. Since drugs administered i/v are placed directly into the central compartment bioavailability is 100% or the fraction absorbed bioavailability (F) is equal to 1. Absolute bioavailability is the amount of drug reaching the central compartment unchanged when given by either enteral or parenteral routes (e.g. orally, i/m, s/c, etc.) as compared to the amount of unchanged drug in the central compartment when given i/v at the same dose rate. The bioavailability is calculated as the ratio of the area under the drug level versus time curve of a drug administered by a specific route e.g. i/m relative to the area under the plasma curve of the i/v route. AUC i/m* F = ________ x 100 AUC i/v Relative bioavailability is when the bioavailability of different drugs are compared or routes other than the i/v route are used for comparison. AUC i/m* ________ AUC s/c* * or any other route e.g. s/c, oral etc. Examples of drug with excellent bioavailabilities are: Theophylline Digitoxin Doxycycline Drugs with poor bioavailability (F < 0,1) are: Aminoglycosides, when given orally Nystatin given orally Drugs with a high first pass effect 31 (b) Volume of distribution Volume of distribution (Vd) is a measurement derived from drug plasma (serum) concentration and provides some idea of the magnitude of distribution. It can be defined as that volume of fluid which would be required to contain the amount of drug in the body if it were uniformly distributed at a concentration equal to that in the plasma. Volume of distribution is a hypothetical volume and does not represent an actual volume or physiological space within the body. It serves as a proportionality constant relating the plasma concentration of a drug to the total amount of drug in the body at any time after distribution equilibrium or pseudoequilibrium has been attained. Amount of drug in body (mg) Vd = ____________________________ Plasma concentration (mg/L) Therefore the larger the plasma concentration the smaller the volume of distribution. The apparent volume of distribution provides no clue as to whether distribution of the drug is uniform or restricted to certain tissues. A large apparent volume of distribution (>1L/Kg) implies wide distribution or extensive tissue binding, or both. Volume of distribution is a useful pharmacokinetic term and is essential for calculating dose of a drug to achieve the desired plasma drug concentration. (c) Biological elimination half-life (T1/2ß) The time required for the concentration of a drug to decrease one-half of its initial value in the blood is known as the half-life (T1/2ß) of a drug. The parameter applies in the case of first order kinetics and is a constant, being independent of concentration. It is clinical useful to know or to calculate the plasma half-life of a drug for calculation of multiple dosage regimens and residues. One can readily calculate the amount of a dose remaining in the body at any multiple of the half-life interval following administration: No. of half-lives % of dose remaining in the body 1 50 2 25 3 12.5 4 6.25 5 3.13 Practically 90 percent of an administered dose is removed after 4 - 5 half lives. If a drug is administered at the same dose to a patient on or before its half-life results, steady state is achieved after five half-lives. By this it is implied that the plasma levels fluctuate at a constant level. 32 No of T1/2 Dose Administered Plasma Conc. before half life Plasma Conc. after half-life 1 20 mg 20 10 2 20 mg 30 15 3 20 mg 35 17.5 4 20 mg 37.5 18.75 5 20 mg 39 Steady 19.5 6 20 mg 40 State 20 7 20 mg 40 Achieved 20 An estimate of the half-life of a drug may be obtained graphically from a semilogarithmic plot of plasma drug concentration (on logarithmic scale) versus time (on a linear scale) following intravenous administration of a single dose of the drug. The half-life is found by measuring the time required for a given plasma level of the drug to decline by 50% during the terminal exponential (ß) phase of the curve. (d) Clearance (Cl) Total body clearance is the volume of blood (ml) cleared of drug per unit of time and can be calculated according to the following formula: Cl = Dose/AUC It is a parameter which is commonly used to calculate dosage interval or rate of infusion to maintain steady state. 3.6.3 Pharmacokinetic analysis Pharmacokinetics is the study of the time course of drug absorption, distribution to body tissues, metabolism and ultimately elimination from the body of a drug following administration. Mathematical approaches have been employed to describe and quantify these processes from drug serum or plasma concentration or urinary excretion data measured over time. Models in pharmacokinetic data analysis are to obtain parameter estimates that can be employed for interpolation and extrapolation. Numerous mathematical models used in pharmacokinetics have been described. This includes the use of linear and non-linear compartmental models, non-compartmental models, physiologically based models, population pharmacokinetic models and pharmacokinetic-pharmacodynamic models. A detailed description of these models does not fall into the scope of this course. 33 4. PHARMACODYNAMICS KNOWLEDGE REQUIRED 1. Define the following pharmacodynamic terms/concepts and where applicable, use graphical illustrations or formulas to explain the effect: o Agonist o Dose response relationship o Drug-receptor interaction o Dualist (mixed agonist-antagonist) o Inverse agonist o Median effective dose (ED50) o Median lethal dose (LD50) o Partial agonist o Pharmacological antagonist o Physical-chemical drug effects o Physiological antagonist o Potency (low vs. high) and vs. efficacy o Safety factor o Safety margin o Second messengers o Tachyphylaxis o Therapeutic index Pharmacodynamics is the study of biochemical and physiological effects of drugs and their basic mechanism of action. Drugs produce an effect by one or more of a variety of actions. Most drugs produce an effect by interacting with a receptor. It is also possible for a drug to produce an effect by a direct physical or chemical interaction which does not involve a receptor. A drug is usually described by its most prominent effect, or by the action which thought to be the basis for that effect. It should be noted, however, that no drug is limited to producing a single effect. Effects from a single drug may be produced by interaction with several types of receptors in different tissues, interaction with similar receptors in different tissues or by a combination of interactions with receptors and direct chemical or physical interactions. This is the main reason why every drug has a side effect. It is important to note that effects considered side effects in one animal species may be a beneficial effect in another species e.g. the opioid analgesics like morphine is known to cause catatonia and severe sedation in people being treated for pain. These effects are unwanted and considered side effects of the drug. However, 34 these effects are desirable in animals being relocated and as such some of the opioids are important game capture drugs. 4.1 MECHANISM OF ACTION OF DRUGS Drug action refers to the method by which the drug influences a cell. Classes of action Local/General/Remote Primary/Indirect Immediate/Delayed Drug effect Drug effect or response is a sequel to drug action. The effects are the detectable changes which follow an alteration in cellular function, initiated by the interaction of the drug with cellular components or contents. Types of drug effects Stimulation Depression Irritation Replacement Chemotherapeutic effect 4.2 PHYSICAL-CHEMICAL ACTION Structural non-specific action Caustics - cause cell death and lysis e.g. silver nitrate Counter-irritants - induce a local inflammatory response but not cell death e.g. liniments Surfactants - e.g. soap and detergents Astringents - provide a protective layer by precipitating proteins Adsorbents - have an ability to bind poisons and toxins Osmotic effects - e.g. saline purgatives Membrane stabilization – the corticosteroids are known to stabilise cell membranes and reduce cell death in time of neuronal injury Membrane fluidity: Certain general anaesthetics interfere with the fluidity of the cell membrane of the neurons. Once this occur the receptors and ion channels no longer function and result in the patient losing consciousness. Structural - Dependent action Chemical reactions e.g. Pb & MgSO4 Chelation e.g. penicillamine Neutralisation e.g. acids aid basis 35 4.3 RECEPTOR MEDIATED DRUG ACTION The majority of drugs produce their effect by operating on a specific receptor macromolecule. This is in essence a chemical reaction, with the drug and receptor molecule acting as reagents. The result is usually an alteration in rate at which a bodily function occurs, i.e., most drug effects are the result of a modulation of the rate of an on-going function and do not create new effects. Some drugs, however, act by creating an abnormal action (some antimicrobials, anticancer agents). 4.3.1 DRUG RECEPTOR COMPLEX (a) Receptor theory A lock-and-key model is the original model used to describe drug-receptor interaction. The key corresponds to the drug and the lock to the receptor. The model illustrates the specificity of the reaction and that small changes in the molecular structure can significantly alter drug potency. However, the implied rigidity of the model does not take into account the flexibility of drug molecules. The current hypothesis is that drug molecules spin in random fashion in the vicinity of the receptor and become stabilized as they approach closely enough to enter the force zone generated by the receptor. The most powerful association will occur when the spatial relationship of the groups, comprising the drug molecule, is matched by an arrangement of groupings at the receptor which maximises the opportunities for the formation of bonds. (b) Receptor binding The active groups on receptors are usually carboxyl, amino, sulfhydryl or phosphate and are spatially orientated in a pattern complementary to that of the drug with which it reacts. All types of bonds are involved: electrostatic; hydrogen; hydrophobic; van der Waals and, in some cases, even covalent. In most interactions a combination of forces are involved. Drug molecules escape from receptor binding when its kinetic energy is raised by random thermal collisions to a level which exceeds the bonding energy. The drug molecule returns to the biophase once the complex is broken. Allosterism describes a change of shape induced in an enzyme molecule (e.g. dihydrofolate reductase inhibition by trimethoprin) following complexation at a site other than its active site, with the substrate other than its customary substrate. Combination with a reagent at this allosteric site may give activation or inhibition of the enzyme by modifying access to its active site. Drug molecules similarly may modify the action of another at its receptor site by allosterism. (c) Cellular sites of drug action Outside of cell Plasma membrane Inside of cell 36 (d) Types of receptors There are two general types of receptors viz. (i) enzymes and other macromolecules eg. acetylcholinesterase, and (ii) membrane associated receptors eg. adenylcyclase. The former is easy to identify and usually can be isolated. On the other hand, there is less known about membrane receptors other than enzymes which are bound to membranes. Receptors may be cytosolic, pore limited or enzyme limited. Receptors may be classified based on their: Pharmacological action Biochemical / Biophysical effects Molecular / Structural form etc.; Anatomical location; Cellular site Isoreceptors are receptors that show differences in the apparent sensitivity range of activating drugs eg. α and ß receptors of adrenaline Drug acceptors or silent receptors are sites at which drugs bind but with which no action is associated eg. plasma binding. Binding is characterised by large-capacity, low affinity type. Suicide inhibitors are drugs which require conversion by their target enzymes into compounds which then irreversibly inhibit an enzyme eg. monofluoroacetate (e) Second messengers Binding of a drug molecule to a membrane-located receptor may be followed by the entry or synthesis of an intracellular second messenger which is responsible for drug action eg. adrenaline after binding to adrenergic receptors activates the membrane- located adenylcyclase. Adenylcyclase catalyses the transformation of adenosine triphiosphate (ATP) to cyclic adenosine monophosphate (c-AMP) within the cell. Increase in c-AMP regulates enzymes via protein kinase which mediates the characteristic cellular response. 4.3.2 AGONISTS AND ANTAGONISTS A drug which can combine with a receptor and elicit a response from the receptor in is called an agonist. An agonist is therefore a drug or substance with affinity for a receptor and which shows intrinsic activity. An antagonist is a drug which, if administered previously or simultaneously with the agonist will diminish or abolish the anticipated agonist response. A dualist is a drug with both agonistic and antagonistic properties, depending on the dose administered eg. mixed opioid antagonists/agonists. An inverse agonist has the potential to bind to a receptor and subsequently stimulates 37 the receptor to become inactivated. In all senses the latter class of drug prevents endogenous ligands from functioning as in the case of a true antagonist (see below). A major difference to the proper antagonist (receptor blocker) is that these drugs promote a response for the receptor (i.e. receptor inactivation), and thus must be considered agonists. Antagonism may be permanent, irreversible or non-competitive if its intensity remains unaffected in the presence of increasing agonist concentration. Non- permanent, reversible or competitive antagonism is overcome by increasing the concentration of the agonist. Competitive or non-competitive reduction or inhibition of agonistic responses are both types of pharmacological antagonism. Physiological antagonism is elicited when a drug reduces the effect of another by activation (agonism) of a second species of receptors with opposing response eg. bronchospasm induced by histamine can be alleviated by the ß2-agonist, clenbuterol. Chemical antagonism eg. neutralization or chelation results in structural changes and inactivation of the agonist. Adsorbents such as activated charcoal cause physical antagonism by physically binding the agonist, thereby are making it unavailable for action, without any structure changes. Tachyphylaxis is a form of desensitization or tolerance of receptors with reduced response occurring with increasing drug concentration. It differs from tolerance in that it occurs more rapidly and may be caused by either pharmacological allosterism, by depletion of endogenous neurotransmittors eg. amphetamine or by induction of destructive enzymes eg. diphosphoesterase. Desensitization usually refers to a specific loss of receptor responsiveness while tolerance and tachyphylaxis define whole animal responses. 4.4 QUANTITATIVE ASPECTS OF DRUG-RECEPTOR INTERACTIONS 4.4.1 Dose-response relationships There are several theories which apply to the relationship between the amount of drug at a receptor site and magnitude of the response obtained. The three most useful are: (a) Occupancy theory (b) Rate theory (c) Spare receptor theory All theories agree that drugs react reversibly with receptors and that one drug molecule reacts with one receptor molecule. No single theory explains all known situations. The occupancy theory is the basis for all of the other theories. According to this theory the extent to which a tissue responds depends on the proportion of its receptor population which has become occupied by a drug. The reaction between drug and receptors may be written as: K1 R + D RD K2 38 where R = receptor, D = Drug, K1 & K2 are the rate constants of association and dissociation of the reversible reaction. The drug-receptor interaction is expected to obey the laws of mass action. At equilibrium the rate of association and dissociation of the complex is regarded as equal to: K1[D][R] = K2[RD] or K1/K2 = [RD]/[D][R] = Kaff Kaff is an affinity constant which characterizes the tendency of the drug to complex with receptors. The rate theory refers to the rate at which receptors are occupied rather than the number of receptors occupied. Inconsistencies between drug behaviour predicted on the basis of receptor occupancy and observed response e.g. in the case of partial agonists, led to the spare receptor theory. On the basis of this theory a drug of high efficacy or intrinsic activity elicits a maximal response after occupying only a small portion of the receptor population and thus leaving a number of spare receptors. 4.4.2 Types of dose-response relationships Two types of dose-response relationships exist viz.: (a) Graded (b) Quantal The graded dose-response relationship refers to the dose response of a drug within an animal on a particular tissue e.g. cardiac. Increasing tissue response, e.g. cardiac rate, is observed with increasing dose. In the quantal dose-response relationship the response is an event, the frequency of whose occurrence in a population is dose dependent. The measurement is an all-or-none response e.g. in establishing the lethal dose of a drug. 4.4.3 Graphical illustration of dose-response relationships A hyperbolic curve is obtained following the graphically representation of data of either a graded or quantal dose response relationship. When the response is expressed as a percentage of the maximum instead of units, and is plotted against the logarithm of the dose, the curve adopts the sigmoid characteristic of a log-percent response curve. The central portion of such curves is more or less linear. This is used for quantitative analysis of dose response data to describe or compare the pharmaco