Human and Applied Medical Genetics Final Exam Review Packet PDF Fall 2024
Document Details
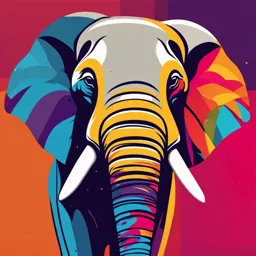
Uploaded by AmicableTsavorite
2024
BISC
Tags
Summary
This document is a review packet for a Human and Applied Medical Genetics final exam, covering material from Fall 2024. It details the mitochondrial and nuclear genomes, types of sequences, and epigenetic modifications. The document likely contains study materials and information on genetic concepts.
Full Transcript
Human and Applied Medical Genetics (BISC 4340/5340) - Fall 2024 Final Exam: The final will include all the new material after test 3 (X-linked, paternal and maternal Inheritance, Unit 5-Multifactorial (Complex Inheritance), and Unit 6- pharmacogenetics. In addition, for the cumul...
Human and Applied Medical Genetics (BISC 4340/5340) - Fall 2024 Final Exam: The final will include all the new material after test 3 (X-linked, paternal and maternal Inheritance, Unit 5-Multifactorial (Complex Inheritance), and Unit 6- pharmacogenetics. In addition, for the cumulative part of the final, you only have to study and understand the following packet. This review packet includes terms and concepts from the early units (the material up to and including test 3) Final Exam Review Packet Human Genome 1. The mitochondrial genome 2. Nuclear Genome 1- Mitochondrial Genome Each human cell contains hundreds of mitochondria; each one contains between 2-10 copies of 16.6 kb circular double-stranded DNA (mtDNA) It forms about 1% of the total mass of cellular DNA. It encodes only a few proteins with mitochondrial function, but most of the mitochondrial proteins are encoded by the nuclear genome. Mitochondria and their DNA are maternally inherited; the trait is transmitted from a mother to all of her children. 2- Nuclear Genome Half of the nuclear genome is inherited from the mother, and the other half from the father. The total genome in the nucleus of a diploid cell is 6 x 109 base pairs (bp) in size. It represents more than 99% of the cellular DNA. The haploid nuclear genome is distributed between 24 different types of DNA molecules. Each molecule consists of linear double-stranded DNA in a complex with histones and non-histone proteins forming highly organized chromosomes. In haploid cells, there are 22 autosomal chromosomes and one sex chromosome (X or Y) Organization of the Human Nuclear Genome The genome is approximately 99.9% the same between individuals of all nationalities and backgrounds. Less than 2% of the genome codes for proteins The vast majority of our DNA is non-protein-coding. The complexity of humans is a result of o The complexity of the regulation of gene expression o Alternative splicing o The evolution of non-coding sequences, such as LnRNA, microRNA, and tandem repeats, regulates gene expression and so mediate more complex processes in human, such as Brain processes. Type of sequences in the Human Nuclear genome A- Single-Copy DNA Sequences These sequences are represented only once in the genome, and only a small part of these sequences are protein-coding genes. Examples of single-copy DNA sequences: I. Protein-coding Genes II. Pseudogenes III. Non-coding RNA (micro-RNA, Long non-coding RNA) IV. Regulatory sequences I- Protein-coding genes: the final product is a functioning protein. Protein-coding genes can be grouped into: o Gene family: Members of a gene family share closely related DNA sequences and encode polypeptides with similar amino acid sequences. o Genes encode proteins with common recognizable functional domains. II- Pseudogenes: Pseudogenes are sequences that resemble known genes but cannot produce functional proteins. Identified function of pseudogenes, regulation of gene expression Different types of Pseudogenes: o Unprocessed 1. Unitary pseudogene 2. Duplicated pseudogene. They retain their characteristic intron-exon structure. They are often located next to their paralogous parent gene. o Processed 3- Retrotransposon or processed pseudogene. They constitute the majority of the pseudogenes in the human genome. They have no introns. Often, they contain a polyadenylation signal. III- Non-coding RNA (micro-RNA, long non-coding RNA) Genes produce a functional RNA as their end product. These noncoding RNA (ncRNA) are expressed as precursors that often undergo enzymatic cleavage to become mature products. RNAs play a wide variety of roles in the cell. Micro-RNA o Micro-RNAs are small single-stranded RNA molecules (about 21-23 nt in length) that regulate the expression of other genes. They are involved in the regulation of gene expression at the translational level. Long non-coding RNA o Nonprotein-coding RNA o They are generally longer than 200 nucleotides and constrained to the nucleus. o They regulate gene expression. o An example of long non-coding RNA is Xist, an RNA product that regulates the epigenetic process of X chromosome inactivation. Micro-RNA and Cancer For a cell to become cancerous, several events must occur, including activation of growth, resistance to signals that normally inhibit growth, and inactivation of programmed cell death (apoptosis). Collectively, these events allow the cell to escape the normal regulatory elements that prevent uncontrolled proliferation. Many miRNAs have been identified and connected to known cancer. Micro-RNA 16 has shown involvement in Chronic Lymphocytic Leukemia (CLL) Mutation in the Micro-RNA 16 coding gene can alter the levels of the micro-RNA, leading to dysregulation of the expression of several target genes involved in cell growth, apoptosis, or survival. The dysregulation of these genes leads to the development of cancer. B- Repetitive DNA Sequences: DNA sequences with multiple copies are distributed throughout the genome. Interspersed Repeats o Interspersed Repeat movement can cause genomic instability = genomic changes. o The two active sequences in humans are, § LINE (Long Interspersed Nuclear Elements) family § The SINE (Short Interspersed Nuclear Elements) family The Alu family is a member of the SINE family: Alu sequences are still active and have shown involvement in diseases due to the insertion in areas that cause genomic instability. Tandem Repeats o A tandem repeat is a DNA sequence of n# of bp repeated in tandem. (n= a range from 2 bp to as high as 2000bp). Genetic polymorphism When the sequence variation in a locus is common and found in more than 1% of chromosomes in the general population, the variant constitutes a Genetic Polymorphism. DNA polymorphism serves as the basis for DNA profiling. In contrast, variations with frequencies of less than 1% are called Rare Variants. Examples of polymorphic sequences in human 1- Variable number tandem repeats (VNTRs): large DNA sequences (10-100 nucleotide) repeated in tandem. 2- Short tandem repeat polymorphism (STRP): a stretch of 2-4 nucleotides repeated between one and a few dozen times. 3- SNP: It is a single nucleotide variation in a genetic sequence. SNPs usually have only two alleles corresponding to the two different bases occupying a particular location in the genome. It occurs at a significant frequency in the population. Genotype to Phenotype Regulation of gene expression at the transcription levels can be through: Genetic regulation of gene expression: Gene expression is regulated by the specific interactions of trans-acting products with cis-acting sequences. Each cell type expresses a restricted subset of genes. Gene expression depends on the availability of the trans-elements and their interaction with the cis-elements (promoters). Epigenetics Epigenetics Definition of epigenetics Epigenetics is the study of regulation of gene expression that is dependent not only on DNA sequence but also on chromatin confirmation. Epigenetic regulation of gene expression is important throughout development. Identical twins can be epigenetically different, which explains why they have different appearances and are sometimes discordant for a disease. Epigenetic Features: 1. Epigenetic modifications are mitotic heritable. Mitotic heritability 2. Epigenetic modifications are influenced by environmental modifications. 1- Epigenetics and Mitotic heritability Epigenetic modifications can be permanently transmitted from one cell generation to the next. Mitotic heritability allows: The epigenetic state is to be maintained in daughter cells. It ensures that the same sets of genes are expressed in daughter cells. It ensures tissue homogeneity. Chromatin structure and conformation: For any particular gene, the transcription rate will depend on its environment and chromatin confirmation. Changes in chromatin structure result in altered gene expression. Chromatin confirmation depends on modifying DNA and Histones, either a closed or relaxed Conformation. Therefore, gene expression depends on DNA sequence (promoters) and chromatin structure (epigenetics). Chromatin confirmation: Heterochromatin I. Constitutive heterochromatin: o Areas of the chromosomes that remain in a relatively high state of condensation. o Constitutive heterochromatin areas are always condensed and transcriptionally inactive, gene- poor areas. Examples of constitutive heterochromatin are areas near the centromeres, areas in the region of the telomeres, or areas on the top of the acrocentric chromosomes. II. Facultative heterochromatin: o It can be condensed in some cells/ tissues (transcriptionally inactive) or loosely packed (transcriptionally active) in other cells/ tissues. o An example of facultative heterochromatin is the X chromosome in Females. Euchromatin The open chromatin represents a large portion of the chromosomes that remain less condensed. Euchromatin contains rather loosely packed chromatid fibers and represents transcriptionally active DNA. Gene-rich area. Specific Epigenetic Modifications Post-translational histone modifications: chemical modification of residues in the N-terminal tails of histones. DNA methylation: methylation of DNA nucleotides. How does histone modification change chromatin Conformation? DNA Methylation In Mammals, DNA methylation occurs at CpG dinucleotides. DNA methylation is catalyzed by de novo methyltransferase (DNMT) enzyme. DNA methylation is maintained through cell division. Environmental stressors alter the DNA methylation status. This can occur in utero, and it could occur after birth. DNA methylation = silencing of gene expression Genomic imprinting A pattern of epigenetic inheritance in mammals Imprinted genes have a monoallelic expression. Some are only expressed if inherited from the father (paternally inherited), and others are only expressed if inherited from the mother (maternally inherited), an allele-specific expression. i.e., In genomic imprinting, there is a difference in gene expression between the allele inherited from the mother and the allele inherited from the father. Expression of imprinted genes is controlled by Imprint Control Regions (ICRs) Imprinted genes exist in clusters, and each cluster is controlled by its own ICR (cis-acting regulatory element) Imprint is associated with DNA methylation at the ICR Imprinting affects the expression of a gene but not its primary DNA sequence; no mRNA is produced from the imprinted gene Imprinting and epigenetic programming ICR methylation is established in primordial germ cells to achieve parent-of-origin-specific marks. These marks are maintained in the embryo after fertilization for normal development. However, the imprints are erased in PGCs before gamete formation to ensure sex-specific imprint renewal in sperms and eggs. Reprogramming of the imprinted genes only occurs in PGCs that drive the gametes (sperm and eggs) in the embryo; see the figure below. Cytogenetics I- Terminology: Homologous chromosomes: Chromosomes that encode similar genetic information with genes arranged in the same sequence at any specific locus. Locus: The place a gene occupies on a chromosome. Alleles: Alleles are different versions of a gene at a given locus. Telomere The ends of each chromosome, It consists of repetitive DNA sequences. Telomeres ensure the integrity of the chromosome during cell division. Telomeres shorten successively with each cell division. Short telomeres accumulate with aging. The centromere is a constriction point in each chromosome, The centromere divides the chromosome into two sections: “arms”: short arm (p) and long arm (q). The centromere location is characteristic of a given chromosome. II- Classification of the Human Chromosomes: A- Classification based on the location of the centromere; a normal karyotype will include all three different types. o Metacentric: Chromosome with central centromere and arms of equal length o Submetacentric: Chromosome with off-center centromere and arms of different length o Acrocentric: Chromosome with centromere near one end B- Chromosomes also can be classified based on the banding pattern. Differential staining along the chromosome depends on the composition of the DNA and chromatin structure. The Sex Chromosomes Most parts of the X and Y chromosomes are nonhomologous, except two regions located at the distal end of both the short and long arms, the so-called pseudoautosomal regions (PAR). The X and Y chromosome genes have a unique expression pattern outside the PAR. This evolutionary diversion results in a large X chromosome with approximately 813 -1000 genes and a small Y chromosome that in humans carries between 45-50 protein-coding genes on the male-specific region. The euchromatin of the present-day Male-Specific Region of the Y chromosome (MSY)contains the SRY locus (Sex-determining Region of the Y chromosome), which plays a role in sex determination during development. It encodes a transcription factor that directly affects the differentiation of the gonads. The role of the Y chromosome in sex determination: Sex determination of an embryo is a complex process determined in part by the sex chromosome complement of the developing embryo. (Genetic part of sex determination) Molecular events involving transcription factors promote the initiation and differentiation of the testis and ovaries. SRY transcription factor is expressed for a short time in the cells of the bipotential gonad area immediately before testicular differentiation occurs. DAX1 represses various genes that promote male differentiation. The immediate function of SRY protein is to antagonize the function of DAX1 protein. In the absence of SRY, DAX1 continues to repress these genes, and a female embryo is developed. The critical decision depends on a titration of SRY against DAX1 X- Inactivation Female mammals, including humans, inherit two X chromosomes; one X chromosome in each cell becomes inactive during embryonic development. It is an epigenetic dose compensation for females. X inactivation occurs early during embryonic development It is a random process, paternal vs maternal chromosome. i.e., the decision to inactivate the maternal or the paternal X is made randomly. Mitotically heritable: whichever of the parental X chromosomes is chosen for inactivation within a cell, that same X (Paternal or maternal) is inactivated in all daughter cells. An adult female is a mosaic of cell clones, each maintaining the pattern of X inactivation established in its progenitor cell early during embryonic development. X-Inactivation Center XIC is a region located on the X-chromosome § XIC is necessary for X inactivation. § XIC contains the XIST locus. Xist is required for X inactivation. § However, maintenance of X inactivation appears to be independent of XIC function and depends on a combination of epigenetic marks. How does the cell sense the presence of multiple X chromosomes? Via the expression levels of Rnf12 Rnf-12 coding gene (locus) is located upstream of XIC. Rnf-12 is a protein involved in the regulation of transcription. The process of X- inactivation It is an epigenetic process. The critical determinant for X inactivation is Xist. Xist is a 17 kb long-noncoding RNA, and its expression is the first detectable event in X inactivation. It is expressed only from one of the two X chromosomes and determines the chromosome that will become inactive. Xist RNA coats the inactive X in Cis. The accumulation of epigenetic marks condenses the targeted X chromosome and inactivates gene expression. The condensed X chromosome is called the Barr body. The inactivated X chromosome is an example of facultative heterochromatin. X-inactivation can influence the severity of X-linked disorders in females. For example, some heterozygous females will express symptoms of Hemophilia A. The Effect of X Inactivation on the Expression of X-linked Phenotype Depending on the pattern of random X inactivation of the two X chromosomes, two females heterozygous for an X-linked disease may have very different clinical presentations because they differ in the proportion of cells that have the mutant allele on the active X chromosome in a relevant tissue. Males are hemizygous for the X-linked genes; therefore, they will be severely affected, and for some disorders, affected males do not survive. Think about how a mother and her daughter may have the same X-linked dominant disease, but one expresses the condition more severely than the other. III- Chromosome abnormalities Chromosomal abnormalities are the leading cause of mental retardation and pregnancy loss. Chromosome abnormalities can alter the gene dosage and cause an imbalance in the level of gene products. It could be: A. Numerical or B. Structural abnormalities Haploinsufficiency: A single functional gene copy is insufficient to maintain normal function. A- Numerical abnormalities Euploid cells: (Normal cells): Somatic cells have a chromosome number 2n. The species' chromosome complement is the chromosome number that is an exact multiple of the haploid number (chromosome # in gametes, n). (ex. 46 chromosomes for human cells) i.e., a balanced set of chromosomes Cells with an abnormal number of chromosomes can be 1) polyploidy or 2) aneuploidy cells 1- Polyploidy: The presence of a complete set of extra chromosomes in a cell, (2n+n) It rarely occurs in humans. The polyploidy conditions observed in humans are triploidy (3n) and tetraploidy (4n). A cell with three sets of chromosomes is triploid, e.g., 69 chromosomes in humans. Triploidy in human It accounts for roughly 20% of all chromosome abnormalities identified in spontaneous miscarriages. The survival rate is very small. Children that are born alive usually die within the first few days. Chromosomal abnormalities are the leading cause of mental retardation and pregnancy loss. Cause of Triploidy 2- Aneuploidy: It occurs when there is a change in the chromosome number that involves less than a whole chromosome set. (Not an exact multiple of the haploid chromosome) e.g. (2n-1) monosomy or (2n+1) trisomy The most common cause of aneuploidy is nondisjunction. Nondisjunction is the failure of chromosomes to disjoin normally during meiosis. Be prepared to answer questions about nondisjunction in meiosis I and meiosis II, and make sure you can differentiate between the two events. Non-Disjunction in Meiosis II You are only responsible for the following clinical examples, which are numerical abnormalities. Down syndrome: an example of Aneuploidy; trisomy 21 (47, XY, +21 or 47,XX, +21) Edward Syndrome (18) 47, XY, +18 or 47, XX, +18 Patau syndrome (13) 47, XY, +13 or 47, XX, +13 Turner syndrome: an example of sex chromosome aneuploidy (45, X) Klinefelter syndrome, 47, XXY Uniparental Disomy (UPD) and Genetic Diseases Uniparental Disomy results from a fertilization event with two abnormal gametes. The zygote can be normal or affected by a disease, depending on if the chromosome that came from the one parent is normal or carries a mutation. Diseases examples that were observed in the population and were reported to be due to uniparental disomy: 1. Angelman syndrome: Two normal paternal chromosomes 15 and the absence of maternal chromosome. 2. Cystic fibrosis: with the two mutated chromosomes being inherited from one parent. Mosaicism and Chromosome Abnormality Mosaicisms designate the presence of two or more populations of cells with different chromosome complements in one individual who has developed from a single fertilized egg (zygote) Mosaicism arises after fertilization and during the early cleavage stage of development. Usually due to mitotic nondisjunction Think about how Mosaicism can sometimes rescue (make it less severe) the phenotype. B- Structural chromosome abnormalities 1- Chromosome deletion: Deletion is a chromosomal abnormality in which a portion of a single chromosome is lost. It results in a partial monosomy. The effects of deletions depend on their size (the phenotype depends on the genotype) Deletions may be inherited from a carrier parent or may occur as de novo rearrangement. Most detectable and severe cases were identified as de novo cases in which the deletion occurs during gamete formation. You are only responsible for the following Clinical examples Cri du chat syndrome (cat’s cry): Results from deleting the terminal part of the short arm of chromosome 5. (46, XX, del(5p)) DiGeorge Syndrome, interstitial deletion of chromosome 22 (46, XY, del(22( (q11)) Prader-Willi syndrome: paternal deletion of chromosome 15. An example of chromosome deletion and genomic imprinting Angelman syndrome: Maternal deletion of chromosome 15. An example of chromosome deletion and genomic imprinting Alpha-thalassemia: deletion of alpha globin gene. 2- Inversions: Inversions are intra-chromosomal structural rearrangements in which the genetic segment is turned 180 degrees within a chromosome. Inversions do not involve losing genetic information but rearranging the linear gene sequence. An inversion requires two breaks along the length of the chromosome and subsequent reinsertion of the inverted segment. Heterozygous for inversions may produce aberrant gametes with a major impact on their offspring. It may have a minimal impact on the carriers unless it disrupts gene expression Inversion can be: Paracentric Inversion: The 2 Breaks are in one arm. It doesn’t include the centromere. Pericentric Inversion: The 2 Breaks spanned the two arms. It includes the centromere. It results in changing the length of the arms. 3- Translocation: exchange of chromosomal part Translocation involves two non-homologous chromosomes in which an exchange of chromosomal material between the two non-homologous chromosomes occurs. Reciprocal Translocation It happens when breaks occur in two different (non-homologous) chromosomes, and the material is mutually exchanged. The total chromosome # is unchanged. No genetic information is gained or lost in the exchange, but genes are moved to new chromosomal locations. The resulting chromosomes are called derivative chromosomes. Example of Reciprocal translocation 46, XY, t(3;6) (p13;p22 In General, Carrier individuals will NOT show any physical phenotypic effects. Carriers may show reproductive abnormalities, infertility, spontaneous abortions, and abnormal offspring. Robertsonian Translocations Robertsonian translocations are unique types of whole-arm translocations. It involves any two acrocentric chromosomes and results from the centric fusion of the long arms of these two chromosomes. dsDNA Break near the centromeres Centric + Lost arms of the short Reduction in the 2 fusion of the long number of chromosomes by 1 1 arms 45,XX,t(14q;21q) Carrier individual will NOT generally show any physical phenotypic effects until reproduction. Be able to determine the carrier parent by examining karyotypes. In general, carrier individuals of inversion or translocation chromosome abnormality will NOT show any physical phenotypic effects. Carriers may show reproductive abnormalities, infertility, spontaneous abortions, and abnormal offspring. Population Genetics & Single-gene Diseases (Mendelian Disorders) Allele Frequencies in Populations and the Factors that Affect Them What is a biological population? A biological population is any group of members of the same species in a given geographical area that can mate and produce offspring. They potentially interbreed. In population genetics, Geneticists consider all the alleles of all the genes in a biological population. These alleles constitute the gene pool. Gene Pool: the set of genetic information carried by a population. The pool refers to the collection of gametes in the population; an offspring represents two gametes from the pool. Population Geneticists study genetic traits in any population using these three different frequencies: 1. Allele frequency 2. Genotype frequency 3. Phenotype frequency For any genetic trait or mutation, such as cystic fibrosis mutation, they look at: § The phenotypic frequency is observable and can be calculated based on the number of individuals in the population that express the phenotype. § The allele and genotype frequency can also be determined by genetic testing or Hardy-Weinberg Equilibrium. Hardy-Weinberg Equilibrium The equilibrium states a simple relationship between the allele frequency at a genetic locus and the genotype frequency resulting from the combination of these alleles. i.e., It is a simple mathematical relationship for calculating genotype frequency from allele frequency. P+q=1 P2 + 2pq + q2 = 1 Assumptions of Hardy-Weinberg Equilibrium 1. The population is large enough that there are no errors in measuring allele frequencies. 2. All genotypes have equal ability to survive and reproduce. 3. Random mating in the population 4. Mutations, migration, or other factors that change allele frequency are absent or rare events and can be ignored. Simply put, allele and genotype frequencies in a population remain constant, in equilibrium, from generation to generation unless specific factors influence the population and change the equilibrium state. Understand how to use H-W equilibrium to calculate allele and genotype frequencies for autosomal and X- linked recessive traits in populations where H-W assumptions are met. Factors that disturb Hardy-Weinberg Equilibrium 1. Small population or if the population has subgroups in isolation from the large population (random event can alter an allele frequency radically) 2. Stratification: subgroups in a large population remained relatively genetically separate during modern times. Ex. in the US, Whites, African American, Asian. In these subgroups, mating is restricted to members of one particular subgroup. 3. Consanguinity and genetic isolates. 4. Increase in the mutation rate. Genetic drift Random changes (fluctuation) in allele frequencies from generation to generation take place in a small population via chance. The degree of fluctuation increases as the population size decreases. v A population bottleneck can cause genetic drift. Genetic bottleneck develops when a large population undergoes a drastic but transient number reduction. The population may recover, but its genetic diversity has been reduced. v Genetic drift can be caused by the Founder effect. Founder effect: When a small number of individuals form a small population in an isolated area, the gene pool of all individuals in the next generations is derived from those of the founders. Mendelian Genetics and Single Gene Inheritance: Genetic analysis for different types of inheritance in humans (pedigree analysis) Genetic analysis in humans is observational and indirect rather than experimental. To establish the transmission pattern, we first collect information about the patient's family history and summarize the details as a pedigree. Once the pedigree is constructed for a trait, the information will show how the trait is inherited and which family members or members are at risk. Pedigree Analysis uses knowledge of Mendelian principles and can determine if the trait has a dominant or recessive inheritance pattern. The pedigree analysis also determines if the gene in question is on an X or Y chromosome or one of the other 22 autosomal chromosomes. Hemizygous, Although we are a diploid species, human males have two types of sex chromosomes, which differ in structure and gene content. Males are hemizygous for such loci. Allelic Heterogeneity Different mutations within a gene (locus) cause a single disorder or trait. There are five nuclear basic Mendelian inheritance patterns You will be asked to identify the pattern of inheritance from a pedigree. I- Autosomal recessive: Horizontal Pattern in a Pedigree. The disorder occurs only in one generation rather than successive generations. Most affected individuals have unaffected parents (recessive traits typically skip generations). Both males and females are affected equally, and both can be carriers and will transmit the disease. All the children of two affected individuals are affected. Consanguinity Consanguinity refers to the mating of related persons. Consanguinity is present more often in pedigrees involving autosomal recessive diseases than in other types of inheritance. Mutant alleles can be passed down in families for generations without the appearance of the disease. Examples of Autosomal diseases: 1) Cystic fibrosis is an inherited disorder that affects the glands that produce mucus, digestive enzymes, and sweat. It affects several body-systems o The gene responsible for CF was cloned, and the protein product was identified as the Cystic fibrosis Transmembrane conductance Regulator (CFTR). o DNA sequence analysis from affected individuals has identified more than 1400 mutations at the CFTR locus. Different mutations cause CF, which is an example of Allelic heterogeneity. Affected individuals can be compound heterozygotes if they carry two different mutations or true homozygotes if they are concerned and carry two of the same mutation. 2) Tay-Sachs Disease is an autosomal recessive disease that destroys the central nervous system (CNS). It is a lysosomal storage disease. o A mutation in Hexosaminidase A, a lysosomal enzyme, causes it. 3) Sickle Cell Anemia: It is the most frequent autosomal recessive heritable hemolytic condition worldwide. o A mutation in the beta-globin gene causes the disease. Genetic Isolates A population is considered a genetic isolate when the frequency of specific rare recessive genes is greater among its people than in the general population. Such populations may have become separated from their neighbors by geographical, religious, or linguistic barriers. These populations are not consanguineous. However, the chance of mating with another carrier of a particular recessive condition may be as high as that observed in cousin marriages. Example: 1. Ashkenazi Jewish community, 2. Finland is an excellent example of a genetic isolate, and there are a series of disorders that are uniquely or predominantly Finnish. II- Autosomal dominant Typical characteristics of autosomal dominant inheritance Every affected individual should have at least one affected parent Successive generations are affected Males and females are affected equally by autosomal traits. Both males and females can transmit the disease to subsequent generations. Complete Dominance: In autosomal dominant conditions, such as Huntington’s Disease, the phenotype of the heterozygote and homozygote are reported to be similar, indicating complete dominance of the disease- causing mutation. Incomplete Dominance: In other conditions, homozygosity for an autosomal dominant allele will result in a more severe phenotype than heterozygosity for that allele; incomplete dominance Obligate carrier: an individual who has an affected parent and affected children, and therefore, he/she must be a carrier of the mutation. You need to understand the concept of obligate carrier and be able to distinguish it from the carrier of an autosomal recessive mutation. Examples of Autosomal Dominant diseases: 1) Achondroplasia: It is a skeletal disorder with short-limbed dwarfism and large head o It Results from mutations in FGFR, trans-membrane tyrosine kinase receptors. o It has a 100% penetrance, and it is an example of incomplete dominance. 2) Marfan syndrome: Marfan Syndrome is a connective tissue disorder that affects the skeletal system, the eyes, the pulmonary system, the skin, and the cardiovascular system (pleiotropic phenotype). o It results from mutations in the fibrillin one gene (FBN1). Fibrillin is an extracellular matrix glycoprotein with wide distribution in connective tissue throughout the body. o Mutations are scattered across the gene, and each mutation is usually unique to a family (allelic heterogeneity) 3) Neurofibromatosis is an autosomal dominant disease of the nervous system, eye, and skin. o It has a pleiotropic phenotype; it is characterized by the growth of multiple benign tumors in different parts of the body, less frequently, mental retardation. o It has a 100% penetrance. o It is an example of variable expressivity. Even within a family, some individuals are severely affected, and some are mildly affected. o Approximately half the cases of Neurofibromatosis result from a new rather than an inherited mutation. 4) Huntington’s Disease: It is an autosomal dominant disorder that affects both males and females. It is a neurological disorder with progressive dementia, movement disturbances The molecular Basis o Huntington’s disease is caused by HD (Huntington’s disease) gene mutations. o The protein is ubiquitously expressed in the body. o Knockout of the HD gene is embryonic lethal, and reduced levels or mutations result in neuronal cell death and neurological dysfunction. o The mutation for HD is composed of an abnormal long expansion of trinucleotides CAG repeats that encode polyglutamine repeats (CAG code for glutamine). o Normal HD gene (with unknown function) carries between 9-35 CAG repeats (average being 18- 19). Affected individuals will have HD gene with 40 to more repeats o The age of the onset of HD is variable. The disease appears to develop earlier and earlier when it is transmitted through the pedigree. The number of repeats can change as the gene is passed from parent to child. Anticipation: The signs and symptoms of some genetic conditions tend to become more severe and appear at an earlier age as the disorder is passed from generation to generation. This phenomenon is called anticipation. Anticipation is most often seen with certain genetic disorders of the nervous system, such as Huntington’s Disease and Myotonic dystrophy. Anticipation typically occurs with disorders that are caused by a trinucleotide repeats expansion.