Final Review PDF - School of Science and Technology
Document Details
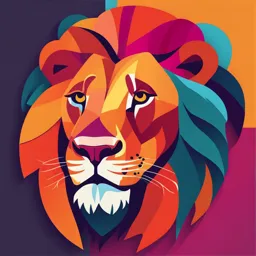
Uploaded by UnboundMaracas
HKMU
Dr. Sophie SHI Ling
Tags
Summary
This document is a final exam review for the School of Science and Technology, focusing on lectures 6-11. It details the exam structure which includes multiple choice questions, short answer questions, and long answer questions and includes example questions on metabolic processes, carbohydrates, and other relevant topics.
Full Transcript
Final Review Dr. Sophie SHI Ling [email protected] School of Science and Technology 1 Final Exam Information ❑ Duration 2 hours ❑ Closed-book Exam ❑ Bring the non-programmable calculators according to HKMU approved list ❑ Topics C...
Final Review Dr. Sophie SHI Ling [email protected] School of Science and Technology 1 Final Exam Information ❑ Duration 2 hours ❑ Closed-book Exam ❑ Bring the non-programmable calculators according to HKMU approved list ❑ Topics Covered: Lecture 1 – Lecture 11 (Mainly focus on lecture 6-11) ❑ Weight of your overall grade: 50% 2 Final Exam Structure Multiple Choice Questions (20%) 20 questions Each question carries 1 mark Short Questions (40%) 7 questions Each question carries different marks Long Questions (40%) Total 5 questions, each question carries 10 marks Select 4 out of 5 to answer 3 Example of Short Questions 1. Name the metabolite or enzyme from 1 to 6 4 Example of Short Questions 2. Name five important enzymes in oxidative phosphorylation. Give one disorder related to dysfunction of oxidative phosphorylation. 3. What are the overall products of TCA cycle? How do these products contribute to energy production? 4. Describe the four levels of protein structure. 5 Example of Long Questions ❑ The format of long questions will be similar to quiz A 45-year-old male presents to the emergency department after several days of severe fasting and significant weight loss. He has a history of type 2 diabetes and reports fatigue, weakness, and confusion. Blood tests reveal elevated ketone levels and a high blood urea nitrogen (BUN) ratio. a) Please identify two metabolic processes regarding the elevated ketone levels and high blood urea nitrogen in this patient. (2 marks) b) How will the activity of acyl-CoA dehydrogenase change in this patient? Why? (4 marks) c) For patients with type 2 diabetes, insulin levels often become insufficient to effectively manage blood glucose utilization. What is the most likely disorder this patient will develop if his diabetic condition is not well-controlled? Why? (4 marks) 6 Lecture 1 Water and Aqueous System 7 Basic properties of water Structural properties Chemical formula: H2O Consists of one atom of oxygen (O) and two atoms of hydrogen (H) A polar molecule ‒ the oxygen has a large nucleus that attracts electrons (strong bond) - ‒ causing the oxygen atom to be negatively charged ‒ whereas the hydrogens are positively charged + (weak bond) Allows water molecules to interact with each other by hydrogen bonds ‒ attraction between positive H in one H2O molecule to negative O in another H2O ‒ weak bond 8 Important properties of water Cohesion and adhesion Cohesion: water molecules stick to each other Example: drop a tiny amount of water onto a very smooth surface, the water molecules will stick together and form a droplet, rather than spread out over the surface Adhesion: water molecules stick to other molecules or substances ‒ Example: the water droplets are stuck to the end of the pine needles 9 Important properties of water High boiling point Water’s boiling point (100°C) is higher than the boiling points of similar substances due to the hydrogen bonds High specific heat More energy to increase the temperature of water Water plays a very important role in temperature regulation This property helps to maintain homeostasis Solvent for polar molecules The polarity and ability of water to form hydrogen bonds makes it an excellent solvent Most of the chemical reactions important to life take place in a watery environment inside of cells Water's capacity to dissolve a wide variety of molecules is key in allowing these chemical reactions to take place 10 Ionization of water Due to the polar structure, water can act as either an acid or a base Acid: can donate a proton (H+) to a base Base: can use a lone pair of electrons to accept a proton (H+) Autoionization of water 11 Ionization of water Equilibrium constant (Kw) for the autoionization of water: Kw = [H+][OH-] or [H3O+][OH−] [H+]: Concentration of H+ [H3O+]: Concentration of H3O+ [OH−]: Concentration of OH- At 25 ℃, the experimentally determined value of Kw in pure water is 1.0×10−14 Kw = [H+][OH−] = 1.0×10−14 In a sample of pure water, the concentrations of H+ and OH− are equal to one another [H+] = [OH−] = 1.0×10−7 No matter whether the aqueous solution is an acid, a base, or neutral, the Kw remains constant For acidic solutions, [H+] > [OH−] For basic solutions, [OH−] > [H+] For neutral solutions, [H+] = [OH−] = 1.0×10−7M 12 What is buffer? Definition An aqueous solution that resists changes in pH upon the addition of an acid or a base Adding water to a buffer or removing water from the buffer does not change the pH of a buffer significantly Constituent a pair of a weak acid and its conjugate base, or a pair of a weak base and its conjugate acid Preparation of buffer mixing a large volume of a weak acid with its conjugate base (eg. acetic acid – acetate ion, CH3COOH – CH3COO-) mixing a large volume of weak base with its conjugate acid (eg. ammonia – ammonium ion, NH3 – NH4+) Example of buffer——Phosphate buffer Consists of a weak base (HPO42-) and its conjugate acid (H2PO4-) The pH of a phosphate buffer is usually maintained at a physiological pH of 7.4 13 Blood as a buffer Human blood contains a buffer of carbonic acid (H2CO3) and bicarbonate anion (HCO3—) maintain blood pH between 7.35 and 7.45 a value higher than 7.8 or lower than 6.8 can lead to death Blood buffer is: HCO3- + H+ H2CO3 With the following simultaneous equilibrium H2CO3 CO2+ H2O When acidic substance enters blood: HCO3- + H+ H2CO3 When basic substance enters blood: H2CO3 + OH- HCO3- + H2O 14 Disorders of acid-base balance Acidosis Acidosis occurs when the blood pH falls below 7.35 It can be classified into two main types: ❑ Respiratory acidosis Caused by an accumulation of carbon dioxide (CO₂) due to respiratory issues Can result from anything that interferes with respiration, such as pneumonia, asthma and chronic obstructive pulmonary disease (COPD) Symptoms may shortness of breath, confusion, drowsiness, and cyanosis (a bluish-purple color of the skin) ❑ Metabolic acidosis Results from an excess of acids or a loss of bicarbonate (HCO₃⁻) Common causes include diabetic ketoacidosis, renal failure, and lactic acidosis Symptoms may include rapid breathing or long, deep breathing, fatigue, confusion or dizziness 15 Disorders of acid-base balance Alkalosis Alkalosis occurs when the blood pH rises above 7.45 It can be classified into two main types: ❑ Respiratory alkalosis Caused by excessive loss of CO₂ Often due to hyperventilation from anxiety, pain, or respiratory diseases Hyperventilation is breathing that is deeper or more frequent than normal Symptoms may include dizziness, tingling in the extremities, and muscle cramps ❑ Metabolic alkalosis Results from an excess of bicarbonate (HCO₃⁻) or a loss of acids Causes can include prolonged vomiting, diuretic use, or excessive antacid consumption Symptoms can include muscle twitching and hand tremors 16 Lecture 2 Carbohydrates, Protein, and Lipids 17 Carbohydrates 18 Classification of carbohydrates——Monosaccharides Functions of monosaccharides Glucose is the main and important source of energy in human, central to energy consumption Galactose is an essential carbohydrate for cellular metabolism, contributing to energy production and storage, can be obtained from milk sugar (lactose) Mannose is a constituent of mucoproteins and glycoproteins required for the proper functioning of the body Fructose exists in fruits and honey; can be metabolized by liver to produce mainly glucose, and minor amounts of glycogen, lactate and a small amount fatty acids in human Ribose is a component of the ribonucleotides from which ribonucleic acid (RNA) is built, and so this compound is necessary for coding, decoding, regulation and expression of genes Deoxyribose, along with phosphate, makes up the sugar-phosphate backbone in deoxyribonucleic acid (DNA), which is the genetic materials for living organisms 19 Classification of carbohydrates——Disaccharides Sources of disaccharides Maltose naturally exists in fruit, such as apples, oranges, peaches, blueberries and cranberries Cellobiose occurs naturally as a basic component of cellulose, a substance produced by plants Sucrose can be found in sugarcane, sugar beets, sugar maple sap, dates, and honey Lactose naturally occurs in milk Functions of disaccharides Maltose is an important intermediate in starch and glycogen digestion Cellobiose is a product of cellulose breakdown and plays a role in the metabolism of certain organisms that can utilize it Sucrose is a product of photosynthesis, providing essential carbon and energy for growth and development in plants Lactose is a source of energy in animals, primarily serving as a carbohydrate 20 found in milk Classification of carbohydrates——Oligosaccharides Oligosaccharides (“Oligo” means a few) compounds that yield 3 to 10 molecules of the same or different monosaccharides on hydrolysis linked to either lipids or amino acid side chains in proteins by N- or O-glycosidic bonds known as glycolipids or glycoproteins N-linked oligosaccharides: ✓ It involves the attachment of oligosaccharides to asparagine O-linked oligosaccharides: ✓ It involves the attachment of oligosaccharides to threonine or serine Functions of Oligosaccharides Glycoproteins are carbohydrates attached to proteins, function as cell-surface receptors, cell- adhesion molecules, immunoglobulins, and tumor antigens Glycolipids are carbohydrates attached to lipids that are important for cell recognition and modulate membrane proteins that act as receptors Cells produce specific carbohydrate-binding proteins called lectins, which facilitate cell adhesion to oligosaccharides 21 Classification of carbohydrates——Polysaccharides Functions of polysaccharides Starch is an energy storage polysaccharide found in plants Glycogen is an energy storage polysaccharide formed in the liver in animals Cellulose is a structural polysaccharide that is found in the cell wall of plants Other polysaccharides Chitin provides important structural stability to fungal cell walls Peptidoglycan is an essential component of bacterial cell walls. It provides strength to the cell wall and participates in binary fission during bacterial reproduction Agarose provides a supporting structure in the cell wall of marine algae Heparin acts as a natural anticoagulant that prevents blood from clotting Chondroitin is an essential component of cartilage that provides resistance against compression 22 Proteins 23 Amino acids——Classification Amino acids with non-polar side chains (hydrophobic) 9 amino acids fall in the category of non-polar side-chain amino acids alanine (A), valine (V), leucine (L), glycine (G), isoleucine (I), methionine (M), tryptophan (W), phenylalanine (F) and proline (P) Alanine (Ala) 24 Amino acids——Classification Amino acids with polar side chains 6 amino acids fall in the category of uncharged polar side-chain amino acids serine (S), threonine (T), cysteine (C), tyrosine (Y), asparagine (N) and glutamine (Q) 25 Amino acids——Classification Positively charged amino acids (Basic) 3 amino acids fall in the category of positively charged amino acids lysine (K), arginine (R), histidine (H) NH2 group in the residue, ionized into NH3+ at a cellular pH Negatively charged amino acids (Acidic) 2 amino acids fall in the category of negatively charged amino acids aspartic acid (D), glutamic acid(E) COOH group in the residue, ionized into COO- at a cellular pH 26 Proteins——Structure Primary structure defined as the linear sequence of amino acids linked together to form a polypeptide chain determined by the encoding sequence of nucleotides in the gene (DNA) The sequence of codons in DNA, copied into messenger RNA, specifies a sequence of amino acids in a protein 27 Proteins——Structure Secondary structure As protein synthesis progresses, interactions between amino acids close to each other begin to occur, giving rise to local patterns called secondary structure Include the well known α-helix and β-strands α-helix A coiled structure, with 3.6 amino acids per turn of the helix (5 helical turns = 18 amino acids) In the α-helix, hydrogen bonds form between C=O groups and N-H groups in the polypeptide backbone that are four amino acids distant These hydrogen bonds are the primary forces stabilizing the α-helix 28 Proteins——Structure β-strands A flat, side-by-side arrangement of polypeptide chains linked together by hydrogen bonds Rather than coils, β-strands have bends and these are sometimes referred to as pleats can be organized to form elaborately organized structures, such as sheets and barrels Higher order β-strand structures are sometimes called super-secondary structures, stabilized by hydrogen bonds In higher order structure, strands can be arranged parallel or anti-parallel amino to carboxyl orientations the same amino to carboxyl orientations opposite of each other 29 Proteins——Structure Tertiary Structure The tertiary structure of proteins represents overall folding of the polypeptide chains, further folding of the secondary structure H-bonds, electrostatic forces, disulfide linkages, and Vander Waals forces stabilize this structure Gives rise to two major molecular shapes called fibrous and globular c c 30 Proteins——Structure Quaternary Structure The spatial arrangement of various tertiary structures gives rise to the quaternary structure composed of two or more smaller protein chains, referred to as subunits describes the number and arrangement of multiple folded protein subunits in a multi-subunit complex In contrast to the first three levels of protein structure, not all proteins will have a quaternary structure since some proteins function as single units Examples of proteins with quaternary structure: hemoglobin, DNA polymerase 31 Lipids 32 Phospholipids Classification Glycerophospholipids: phospholipids with a glycerol backbone Sphingolipids: phospholipids with a sphingosine backbone The main lipid component of cell membranes and are important in the cells semi-permeability Biological membranes usually involve two layers of phospholipids, called a phospholipid bilayer Each phospholipid has a hydrophilic head and a hydrophobic tail The hydrophobic tails face inward , ensuring their protection from the aqueous environment Glycerophospholip Sphingolipid id 33 Glycolipids Classification ❑ Glycoglycerolipid composed of glycerol, fatty acids, and carbohydrates, more in plants Examples: galactolipids, sulfolipids ❑ Glycosphingolipids composed of sphingosine, fatty acids, and carbohydrates; predominant in human and animals Examples: cerebrosides, gangliosides galactolipid galactose sulfolipid sulfated 34 glucose Fatty acids A carboxylic acid with an aliphatic chain, which is either saturated or unsaturated important dietary sources of fuel for animals important structural components for cells ❑ Saturated fatty acids Fatty acids whose hydrocarbon chains do not contain any double bonds Associated with high levels of total plasma cholesterol, increased risk of heart disease and stroke Rich sources of dietary saturated fatty acids include butter fat, meat fat, and tropical oils ❑ Unsaturated fatty acids Fatty acids whose hydrocarbon chains contain one or more double bonds Monounsaturated fatty acids: contain one unsaturated or double bond Polyunsaturated fatty acids: have more than one unsaturated carbon bonds in the molecule Can be found in avocado, fish oil involved in many body functions, including neuro-protective, 35 antioxidant, anti-inflammatory effects and cardiovascular health Polyunsaturated Fatty acids (PUFA) Classification ❑ Omega-3 fatty acids have a terminal carbon-carbon double bond in the omega three-position, the third bond from the methyl end of the acid important constituents of animal lipid metabolism, play an important role in the human diet and physiology Three important types: α-linolenic acid (ALA), eicosapentaenoic acid (EPA) and docosahexaenoic acid (DHA) DHA and EPA are found in algae and fish, while ALA can be found in plants Intake of omega-3 fatty acids levels are associated with lower incident risk for cardiovascular disease ❑ Omega-6 fatty acids Methyl end have a terminal carbon-carbon double bond in the omega six-position, the sixth bond from the methyl end of the acid help stimulate skin and hair growth, maintain bone health, regulate metabolism, and maintain the reproductive system Foods like nuts, seeds, eggs, and vegetable oils are all excellent sources of omega-6 fatty acids Examples: linoleic acid, arachidonic acid 36 Lecture 3 Nucleic Acids 37 DNA Structure DNA is made of two linked strands that wind around each other to resemble a twisted ladder — a shape known as a double helix Each strand has a backbone made of deoxyribose (sugar) and phosphate groups Attached to each sugar is one of four bases: adenine (A), cytosine (C), guanine (G) or thymine (T) The two strands are connected by hydrogen bonds between the paired bases Adenine (A) forms two hydrogen bonds with Thymine (T), and Guanine (G) forms three hydrogen bonds with Cytosine (C) The 5' carbon has a phosphate group attached to it and the 3' carbon a hydroxyl (-OH) group The two DNA strands are antiparallel to each other One strand runs in the 5' to 3' direction, the complementary strand runs in the 3' to 5' direction 38 https://biomodel.uah.es/en/model3/adn.htm Functions of DNA Storage of genetic information DNA stores information in the sequence of its bases The information stored in the order of bases is organized into genes: each gene contains information for making a functional product In eukaryotes, DNA stores genetic information mainly in the nucleus Transmit genetic materials through replication DNA replication is the biological process of producing two identical replicas of DNA from one original DNA molecule Essential for cell division during growth and repair of damaged tissues Ensures that the genetic information of an organism is accurately passed on to its offspring during cell division Instruction of protein synthesis In the first step, the information in DNA is transferred to a messenger RNA (mRNA) molecule by way of a process called transcription During the second step called translation, mRNA is "read" according to the genetic code, which relates the DNA sequence to the amino acid sequence in proteins The mRNA sequence is thus used as a template to assemble—in order—the chain of amino acids that form a protein 39 Ribonucleic acid (RNA) Major types of RNA mRNA (messenger RNA) rRNA (ribosomal RNA) tRNA (transfer RNA) Properties of RNA RNA, containing a ribose sugar, is more reactive than DNA and is not stable in alkaline conditions RNA strands are continually made, broken down and reused RNA’s mutation rate is relatively higher RNA is more versatile than DNA, capable of performing numerous, diverse tasks in an organism 40 RNA structure Characteristics RNA is usually a single-stranded helix The strand has a 5′ end (with a phosphate group) and a 3′ end (with a hydroxyl group) Made of ribonucleotides that are linked by phosphodiester bonds The nitrogenous bases that compose the ribonucleotides include adenine (A), cytosine (C), guanine (G), and uracil (U) A pair with U, C pair with G Structural difference between RNA & DNA Thymine (T) in DNA is replaced by uracil (U) in RNA The sugar in RNA is ribose rather than deoxyribose as in DNA RNA is usually single-stranded, while DNA is double-stranded helix 41 Function of RNA ❑ mRNA Carries information specifying amino acid sequences of proteins from DNA to ribosomes Code for proteins ❑ tRNA Transports amino acids to site of protein synthesis Serve as adaptors between mRNA and amino acids during protein synthesis ❑ rRNA Form the core of the ribosome’s structure & catalyze protein synthesis ❑ miRNA, siRNA & shRNA Regulate gene expression 42 Lecture 4 Metabolism and Energy I: Tricarboxylic Acid (TCA) Cycle 43 Pyruvate Oxidation in Cellular Respiration Pyruvate oxidation is a key metabolic step linking glycolysis and the TCA cycle Converting pyruvate into Acetyl-CoA Pyruvate Oxidation Occurs in the mitochondrial matrix TCA cycle Enabling the complete oxidation of glucose Critical for ATP production and overall cellular metabolism 44 Acetyl coenzyme A (Acetyl-CoA) Definition A metabolic intermediate that is involved in many metabolic pathways in an organism Composed of an acetyl group (CH₃CO−) linked to Coenzyme A Acetyl-CoA is produced during the breakdown of glucose, fatty acids, and amino acids Function Energy Production: A key molecule in the tricarboxylic acid (TCA) cycle, which is a series of chemical reactions that occur in the mitochondria of cells and is responsible for generating energy in the form of ATP Biosynthesis: Precursor for fatty acids, cholesterol and ketone bodies Amino Acid Metabolism: Participates in the metabolism of certain amino acids Structure Acetyl group 45 Acetyl coenzyme A (Acetyl CoA) Sources of Acetyl CoA 46 Acetyl coenzyme A (Acetyl CoA) Fates of acetyl CoA Complete oxidation of the acetyl group in the TCA cycle for energy generation Conversion of excess acetyl CoA into the ketone bodies, acetoacetate and β-hydroxybutyrate in liver Transfer of acetyl as citrate to the cytosol with subsequent synthesis of long-chain fatty acids and sterols 47 Pyruvate Definition Pyruvate is the conjugate base of pyruvic acid, a key intermediate in many biological processes Chemical Formula: C₃H₄O₃ Pyruvate is produced during glycolysis, it’s the end product of glucose breakdown Pyruvate is also formed by the degradation of amino acids Function Energy Production ✓ Can be converted to acetyl-CoA for entry into the TCA cycle ✓ Reduced to lactate in anaerobic conditions (e.g., during intense exercise) Metabolic Hub: Links carbohydrate metabolism to fat and protein metabolism Structure 48 Pyruvate Metabolic Sources and Fates of Pyruvate 49 Summary of TCA cycle For each acetyl-CoA molecule, the products of the TCA cycle are: Two CO2, three NADH molecules, one FADH2 molecule, and one GTP/ATP molecule 50 Roles of TCA cycle 1. Energy Production ATP Generation: Produces NADH and FADH₂ for ATP synthesis in the electron transport chain Substrate-Level Phosphorylation: Directly generates GTP (or ATP) 2. Metabolic Intermediates (Metabolic Hub) Sources of Biosynthesis: Supplies intermediates for amino acids and nucleotides Anaplerotic Reactions: Replenishes cycle intermediates for other pathways 3. Linking Pathways Integration with Glycolysis: Connects carbohydrate metabolism (via pyruvate) to energy production Fatty Acid Oxidation: Acetyl-CoA from fat metabolism enters the cycle, integrating lipid and carbohydrate metabolism 4. Regulation of Metabolism Regulated by substrate availability; sensitive to energy needs (ATP, ADP, NADH) Feedback Mechanisms: Ensures homeostasis based on cellular energy levels 5. Removal of Metabolic Wastes CO₂ Production: Facilitates removal of carbon waste, maintaining acid-base balance 51 Lecture 5 Metabolism and Energy II: Oxidative Phosphorylation 52 Oxidative Phosphorylation What is oxidative phosphorylation? The process by which ATP is produced through the transfer of electrons in the electron transport chain (ETC) and the creation of a proton gradient Oxidative: The oxidation reactions that take place in the electron transport chain (ETC) Phosphorylation: The addition of a phosphate group (Pi) to ADP to form adenosine triphosphate (ATP) Location Occurs in the inner mitochondrial membrane Components 1. Electron Transport Chain (ETC) Transfers electrons from electron carriers (NADH and FADH₂) to oxygen Composed of four main protein complexes (I, II, III, IV) and mobile carriers Coenzyme Q (ubiquinone) and cytochrome c Electrons move through the protein complexes, releasing energy This energy is used to pump protons (H⁺ ions) from the mitochondrial matrix into the intermembrane space, creating a proton gradient 2. Chemiosmosis (ATP Synthesis) Utilizes the proton gradient established by the ETC to synthesize ATP ATP synthesis is catalyzed by the enzyme ATP synthase 53 Function of Oxidative Phosphorylation 1. ATP Production Primary Role: Generates approximately 26-28 ATP molecules per glucose molecule, providing energy for cellular processes 2. Energy Conversion Converts energy from reduced cofactors (NADH and FADH₂) into chemical energy stored in ATP 3. Cellular Respiration Integration Completes the process of cellular respiration by utilizing oxygen as the final electron acceptor, producing water 4. Metabolic Regulation Regulates key metabolic pathways by responding to changes in cellular energy demands; ATP levels influence various biochemical reactions 5. Heat Generation Some energy is dissipated as heat, aiding in thermoregulation and maintaining body temperature in humans 54 Electron Transport Chain Steps Step 1: Transfer of electrons from NADH to Coenzyme Q NADH, produced during glycolysis and the citric acid cycle, donates electrons to complex I Complex I transfers the electrons to Coenzyme Q while pumping protons across the inner mitochondrial membrane, creating a proton gradient Step 2: Transfer of electrons from FADH2 to Coenzyme Q The oxidation of succinate to fumarate results in the reduction of FAD to FADH2 The electrons from FADH2 enter the electron transport chain catalyzed by complex II Complex II doesn’t pump any protons across the membrane Step 3: Transfer of electrons from CoQH2 to cytochrome c Complex III receives electrons from Coenzyme Q and transfers them to cytochrome c As electrons are transferred in complex III, protons are pumped across the inner mitochondrial membrane. Cytochrome c accepts electrons from complex III and shuttles them to complex IV Step 4: Transfer of electrons from cytochrome c to molecular oxygen Complex IV receives electrons from cytochrome c and transfers them to molecular oxygen (O 2) The transfer of electrons to oxygen leads to the formation of water (H 2O) Complex IV also pumps protons across the inner mitochondrial membrane https://www.youtube.com/watch?v=nCr3iCzX4lc 55 Summary of Electron Transport Chain Electron Transfer NADH and FADH₂ donate electrons ✓ NADH → Complex I two electrons transfer ✓ FADH₂ → Complex II two electrons transfer Proton Pumps Energy from electron transfer drives proton pumping ✓ Complex I: Pumps 4 protons (H⁺) ✓ Complex III: Pumps 4 protons (H⁺) ✓ Complex IV: Pumps 2 protons (H⁺) NADH: 10 protons pumped into the intermembrane space per 2 electrons transferred FADH2: 6 protons pumped into the intermembrane space per 2 electrons transferred Role of Oxygen Final Electron Acceptor ✓ Oxygen is reduced at Complex IV ✓ Accepts 2 electrons and combines with protons to form water (H₂O) ✓ Requires 4 electrons to reduce 1 O₂ molecule 56 Proton Motive Force (PMF) Definition The proton motive force is the electrochemical gradient of protons (H⁺ ions) across a biological membrane, primarily the inner mitochondrial membrane in eukaryotes Components Difference in H⁺ concentration (more H⁺ outside than inside) Difference in charge (more positive outside due to H⁺ accumulation) Formation Active transport of protons by complexes I, III, and IV in the ETC Function Drives ATP synthesis via ATP synthase 57 Mechanism of ATP Synthesis Process 1. Proton Gradient Formation Electrons are transferred through the electron transport chain (ETC) Protons (H⁺) are pumped from the mitochondrial matrix to the intermembrane space, creating a proton gradient 2. Proton Flow through ATP Synthase Protons flow back into the matrix through the F₀ component of ATP synthase This flow is driven by the gradient and electrochemical potential 3. Rotation of F₀ The influx of protons causes the c-ring in the F₀ component to rotate This mechanical energy is transmitted to the F₁ component 4. ATP Formation The rotational energy induces conformational changes in the F₁ component, facilitating the binding of ADP and inorganic phosphate (Pi) This leads to the synthesis of ATP from ADP and Pi 58 Lecture 6 Carbohydrate metabolism 59 Part I: Glycolysis 60 Cellular respiration 61 Introduction to carbohydrate metabolism ❑ The major pathways of carbohydrate metabolism begin or end with glucose ❑ Glucose is the major form in which carbohydrate absorbed from the intestinal tract is presented to the cells of the body ❑ Glycolysis —— Catabolic Process A pathway used by all body cells to extract part of the chemical energy inherent in the glucose molecules Converts glucose to pyruvate and sets the stage for complete oxidation of glucose to CO2 and H2O, produces ATP ❑ Gluconeogenesis —— Anabolic Process A metabolic pathway that results in the biosynthesis of glucose from certain non-carbohydrate carbon substrates Requires ATP, reactions catalyzed in the reverse direction of glycolysis 62 Glycolysis ❑ Glycolysis means degradation of glucose Glyco- means sugar (e.g. glycoprotein, glycolipid); Lysis means breakdown (e.g. hydrolysis) ❑ Location Glycolysis primarily occurs in the cytoplasm of the cell ❑ Glycolysis is a preparatory pathway for aerobic metabolism of glucose Glycolysis is possessed by all cells of the human body, in which anaerobic degradation of glucose to lactate occurs Capable of producing 2 molecules of ATP from 1 molecule of glucose in the absence of oxygen In the presence of oxygen, the end product of glycolysis is pyruvate rather than lactate Pyruvate is then completely oxidised to CO2 and H2O (pyruvate oxidation + TCA cycle) with much more ATP produced 63 Glycolysis pathway Glycolysis occurs in two phases Preparatory phase (step 1-5) Payoff phase (step 6-10) 64 Step 1: Phosphorylation of glucose into glucose-6-phosphate Glucose is activated by phosphorylation Catalyzed by hexokinase Requires ATP acts as phosphoryl donor Produces glucose 6-phosphate This step traps glucose inside the cell Irreversible reaction Acts as a key regulatory point in glycolysis Hexokinase requires Mg2+ for activity 65 Step 2: Isomerization of glucose-6-phosphate Glucose is isomerized to fructose Glucose 6-phosphate is reversibly isomerized to fructose 6-phosphate Catalyzed by glucose-6-phosphate isomerase or phosphoglucoisomerase Converts an aldose into a ketose C-1 is easier to phosphorylate by kinase in step 3 Allow symmetric cleavage by aldolase in step 4 66 Step 3: Phosphorylation of fructose-6-phosphate Fructose 6-phosphate is phosphorylated Fructose-6-phosphate is converted into fructose-1,6-bisphosphate Catalyzed by phosphofructokinase-1 (PFK-1) Requires ATP acts as phosphoryl donor This step is the further activation of glucose PFK-1 is inhibited by ATP and citrate, and activated by AMP and fructose-2,6-bisphosphate Acts as a major regulatory point in glycolysis, responding to the energy status of the cell 67 Step 4: Cleavage of fructose 1, 6-diphosphate Fructose 1,6-phosphate is cleaved Catalyzed by aldolase Yields two triose phosphates: ✓ Dihydroxyacetone phosphate, an aldose ✓ Glyceraldehyde 3-phosphate, an ketose The remaining steps in glycolysis involve three-carbon units, rather than six carbon units 68 Step 5: Isomerization of dihydroxyacetone phosphate Dihydroxyacetone phosphate is converted to glyceraldehyde 3-phosphate Dihydroxyacetone phosphate cannot be degraded in the subsequent reactions Thus, it is isomerized into glyceraldehyde 3-phosphate Catalyzed by triose phosphate isomerase This reaction completes the first phase of glycolysis 69 Step 6: Oxidative phosphorylation of glyceraldehyde 3-phosphate Glyceraldehyde 3-phosphate is oxidized Glyceraldehyde 3-phosphate is converted into 1,3-bisphosphoglycerate Catalyzed by glyceraldehyde 3-phosphate dehydrogenase NAD+ is reduced to coenzyme NADH Since two molecules of glyceraldehyde 3-phosphate are formed from one molecule of glucose, two NADH are generated in this step This step is the preparation for ATP production 70 Step 7: Transfer of phosphate from 1, 3-biphosphoglycerate to ADP Phosphoryl group transfers from 1,3-bisphosphoglycerate to ADP The ATP-generating step of glycolysis Catalyzed by phosphoglycerate kinase Produces ATP and 3-phosphoglycerate Two ATPs are generated in this step 71 Step 8: Isomerization of 3-phosphoglycerate The 3-phosphoglycerate is converted into 2-phosphoglycerate Due to the shift of phosphoryl group between C-3 and C-2 of glycerate Catalyzed by phosphoglycerate mutase Reversible isomerization reaction 72 Step 9: Dehydration 2-phosphoglycerate Removal of a molecule of water The 2-phosphoglycerate is dehydrated into phosphoenolpyruvate Catalyzed by enolase (phosphopyruvate hydratase) Two water molecules are lost Generate a high-energy phosphate compound Prepare to produce ATP ❖ Phosphoenolpyruvate has a very high standard free energy of hydrolysis (-62 kJ/mol) 73 Step 10: Transfer of phosphate from phosphoenolpyruvate Phosphoryl group transfers from phosphoenolpyruvate to ADP This is the second energy-generating step of glycolysis Phosphoenolpyruvate is converted into an enol form of pyruvate The enol pyruvate, rearranges rapidly and non-enzymatically to yield the keto form of pyruvate Catalyzed by pyruvate kinase Two ATPs are produced Irreversible reaction Serving as a key regulatory point in glycolysis 74 Regulation of glycolysis Key Regulatory Enzymes Hexokinase —— Step 1 Phosphofructokinase-1 (PFK-1) —— Step 3 Pyruvate kinase —— Step 10 Substrate Availability Concentrations of glucose and its metabolites influence enzyme activity, adjusting glycolytic flux Feedback Inhibition Accumulation of end products (e.g., ATP) inhibits earlier steps, preventing overproduction of ATP and maintaining balance 75 Summary of glycolysis The overall process of glycolysis results in the following events: 1) Glucose is oxidized into pyruvate 2) NAD+ is reduced to NADH 3) ADP is phosphorylated into ATP Overall reaction Glucose + 2 NAD+ + 2 ADP + 2 Pi 2 Pyruvate + 2 NADH + 2 H+ + 2 ATP ✓ Used 1 glucose and 2 ATP ✓ Produced 2 pyruvates, 2 NADH and 4 ATP ✓ Net ATP Production: 2 ATP 76 ✓ Glycolysis is heavily regulated, producing ATP only when needed Significance of glycolysis All tissues employ the glycolytic pathway for the breakdown of glucose to provide energy in the form of ATP An important pathway for the production of energy, especially under anaerobic conditions It is crucial for the generation of energy in cells without mitochondria It forms products that are intermediates for other metabolic pathways Glycolysis interfaces with glycogen metabolism, the pentose phosphate pathway, the formation of amino sugars, triglyceride synthesis, the production of lactate, and transamination with alanine 77 Disorders of Glycolysis Pyruvate Kinase Deficiency Description: Genetic deficiency in pyruvate kinase Symptoms: Hemolytic anemia, jaundice, fatigue Impact: Reduced ATP production leads to red blood cell destruction Phosphofructokinase Deficiency Description: Deficiency in phosphofructokinase (PFK) Symptoms: Exercise intolerance, muscle cramps, myoglobinuria Impact: Impaired glycolytic flux during exertion Hexokinase Deficiency Description: Genetic condition affecting hexokinase enzyme Symptoms: Hemolytic anemia, muscle weakness, lactic acidosis Impact: Decreased glucose phosphorylation, affecting glycolysis Lactic Acidosis Description: Accumulation of lactic acid due to anaerobic metabolism Causes: Impaired mitochondrial function or excessive glycolysis Symptoms: Nausea, rapid breathing, muscle pain Diabetes Mellitus Type 1 Diabetes ✓ Insufficient insulin disrupts glycolysis and increases gluconeogenesis Type 2 Diabetes ✓ Insulin resistance alters glycolytic pathway, contributing to hyperglycemia 78 Part II: Pentose phosphate pathway 79 Pentose phosphate pathway (PPP) Definition ❑ A metabolic pathway parallel to glycolysis ❑ Generates NADPH and pentoses (five-carbon sugars) as well as ribose 5-phosphate, a precursor for the synthesis of nucleotides ❑ especially important in red blood cells (erythrocytes) Location Occurs exclusively in the cytoplasm In humans, it is found to be most active in the liver, mammary glands, and adrenal cortex Processes Oxidative phase ‒ Generate NADPH Non-oxidative phase ‒ Synthesis of five-carbon sugars 80 NADPH regulates pentose phosphate pathway Glucose 6-phosphate has two routes: ✓ Glycolysis for ATP ✓ Pentose phosphate pathway for NADPH and pentose NADPH regulates partitioning between glycolysis and pentose phosphate pathway ✓ If NADPH is quickly used by cell: increased flux of glucose 6-phosphate through PPP ✓ If NADPH accumulates: PPP slows and increases the utilization of glucose 6-phosphate in glycolysis 81 Function of pentose phosphate pathway Redox balance and protection against oxidative stress NADPH is particularly important in protecting cells against oxidative stress Reactive oxygen species (ROS), are natural byproducts of cellular metabolism and can cause damage to cells if not properly neutralized NADPH plays a vital role in maintaining the cellular redox balance, ensuring that the ratio of reduced to oxidized species remains optimal for cellular function Red blood cells heavily rely on the PPP for NADPH production and redox balance maintenance Nucleotide synthesis The production of ribose 5-phosphate (R5P) through the non-oxidative phase of the PPP ensures a steady supply of this precursor for nucleotide biosynthesis 82 Regulation of PPP Pathway Key Regulatory Enzymes ❑ Glucose-6-Phosphate Dehydrogenase (G6PD) Activation: ✓ NADP+: High levels stimulate G6PD, promoting NADPH synthesis Inhibition: ✓ NADPH: High concentrations inhibit G6PD, reducing PPP activity ✓ Citrate: Signals sufficient energy supply, inhibiting G6PD Substrate Availability Glucose-6-Phosphate (G6P) ✓ Increased levels stimulate the PPP, while low levels indicate reduced need Cellular Demand for NADPH Increased demand for NADPH (e.g., during fatty acid synthesis) activates the PPP to meet metabolic needs 83 Part III: Gluconeogenesis 84 What is gluconeogenesis? ❑ Gluconeogenesis means “new formation of sugar” ✓ Gluco- means “glucose” ✓ Neo- means “new” ✓ Genesis means “production” ✓ The metabolic pathway that synthesizes glucose from non-carbohydrate sources, primarily occurring in the liver ❑ Conversion of Pyruvate to Glucose Pyruvate is derived from lactate, amino acids, and other precursors ❑ Importance Maintaining Blood Glucose Levels ✓ Essential for supplying glucose to vital organs, especially the brain and red blood cells Energy Regulation ✓ Helps maintain energy balance during periods of low carbohydrate intake 85 Gluconeogenesis Pathway 86 Lecture 7 Protein and Lipid Metabolism 87 Class of Biomolecules for Energy Step 1: Production of acetyl-CoA ❑ Glucose To pyruvate via glycolysis To acetyl-CoA by pyruvate dehydrogenase ❑ Fatty acid To acetyl-CoA via β-oxidation ❑ Amino acid To acetyl-CoA via oxidation Step 2: Oxidation of acetyl-CoA via TCA cycle Generation of NADH and FADH2 Step 3: ATP production from NADH and FADH2 via oxidative phosphorylation 88 Part I: Protein Metabolism 89 Transamination Removal of amino groups from amino acids and transfer to keto acids Catalyzed by aminotransferase or transaminase Requires coenzyme pyridoxal phosphate (PLP, the active form of vitamin B6) Found in high concentrations in the liver The original alpha-keto acid loses its keto group and gains an amino group, becoming a nonessential AA α-ketoglutarate is a general acceptor of amino group, glutamate is a temporary storage of amino group carbon skeletons amino group carbon skeletons amino group 90 Oxidative deamination Glutamate formed by transamination is deaminated Catalyzed by glutamate dehydrogenase Produce α-ketoglutarate and ammonia (NH4+) Ammonia (NH4+) processed to urea for excretion Urea cycle TCA cycle 91 Excreted fate of amino group —— Urea cycle The nitrogen of amino acids, converted to ammonia, is toxic to the body Thus, it is converted to urea and detoxified Urea is synthesized in liver and transported to kidneys for excretion in urine Urea has two amino (NH2) groups, one derived from NH3 and the other from aspartate Carbon atom is supplied by CO2 Urea synthesis is a five-step cyclic process, with five distinct enzymes The first two reactions take place in mitochondria, while the rest occur in cytosol 92 Excreted fate of amino group —— Urea cycle 1. Synthesis of carbamoyl phosphate Enzyme: Carbamoyl Phosphate Synthase I (CPS I) Rate-limiting step Consume ATP 2. Formation of citrulline Enzyme: Ornithine Transcarbamoylase 3. Synthesis of arginosuccinate Enzyme: Arginosuccinate Synthase Consume ATP 4. Cleavage of arginosuccinate Enzyme: Arginosuccinase Product: Arginine & Fumarate Fumarate link with TCA cycle, gluconeogenesis 5. Formation of urea Enzyme: Arginase Product: Urea and Ornithine Ornithine enters mitochondria and reused in urea cycle 93 Significance of Urea Cycle Toxic NH4+ is converted into harmless urea via urea cycle Helps maintain nitrogen homeostasis in the body by managing excess nitrogen TCA cycle intermediates are recycled Amino acids and keto acids are recycled Ornithine is precursor of prolines and polyamines 94 Regulation of urea cycle Enzyme activity The first reaction catalyzed by carbamoyl phosphate synthase I (CPS I) is rate-limiting reaction in urea cycle CPS I is activated by N-acetylglutamate (NAG) The rate of urea synthesis in liver is correlated with the concentration of N-acetylglutamate High concentrations of arginine increase NAG, the consumption of a protein-rich meal increases the level of NAG in liver, leading to enhanced urea synthesis Substrate concentrations The urea cycle is regulated in part by the availability of substrates Large quantities of citrulline, arginine, or urea help prevent excessive urea formation High concentrations of ammonia and ornithine promote the advancement of the urea cycle 95 Urea cycle disorders Hyperammonemia A metabolic condition characterized by the raised levels of ammonia, leading to toxicity Caused by metabolic defects associated with the five enzymes Individual Disorders Defect Enzyme involved Hyperammonemia type I Carbomoyl phosphate synthase I Hyperammonemia type II Ornithine transcarbamoylase Citrullinemia Arginosuccinate synthase Arginosuccinic aciduria Arginosuccinase Hyperargininemia Arginase Symptoms Lethargy, poor eating, vomiting, seizures, and developmental abnormalities are among the signs of these illnesses that frequently manifest 96 Fate of carbon skeletons —— Gluconeogenesis Carbon skeleton metabolism Amino group is removed by transamination Remaining carbon skeleton (α-keto acid) is catabolized by a pathway unique to that acid Glucogenic amino acids Form any of the intermediates of carbohydrate metabolism Can subsequently be converted to glucose via gluconeogenesis Metabolized to pyruvate or metabolites of the TCA cycle: ✓ Pyruvate ✓ Succinyl-CoA ✓ Oxaloacetate ✓ α-Ketoglutarate ✓ Fumarate 13 amino acids are exclusively glucogenic 97 Fate of carbon skeletons —— Ketogenesis Ketogenic amino acids Can be converted to acetoacetyl-CoA or acetyl-CoA Used for the synthesis of ketone bodies but not glucose Enter 1 of 3 metabolic pathways: ✓ Enter the TCA cycle to produce ATP/energy ✓ Ketogenesis (production of ketone bodies) ✓ Synthesis of fatty acids or cholesterol Only 2 amino acids are exclusively ketogenic 98 Glucogenic and Ketogenic Amino Acids Glucogenic & Ketogenic Metabolized to intermediates of both gluconeogenesis and ketogenesis pathways 5 amino acids are both glucogenic and ketogenic 99 Part II: Lipid Metabolism 100 Process of β-Oxidation Activation of Fatty Acids Fatty acids are converted to fatty acyl-CoA in the cytoplasm using ATP Transport into Mitochondria Fatty acyl-CoA is transported into mitochondria via the carnitine shuttle Four Main Steps of Beta Oxidation 1. Oxidation (Dehydrogenation) 2. Hydration 3. Second Oxidation (Dehydrogenation) 4. Thiolysis Energy sources Each cycle of β-oxidation produces: 1 molecule of Acetyl-CoA 1 molecule of FADH₂ 1 molecule of NADH 101 Fatty Acid Oxidation —— β-Oxidation 102 Step 1: Oxidation (Dehydration) by FAD Substrate acyl-CoA, the activated form of the fatty acid Enzyme The enzyme that catalyzes this reaction is acyl-CoA dehydrogenase Reaction The acyl-CoA undergoes a dehydrogenation reaction where two hydrogen atoms are removed This process converts the acyl-CoA to trans-Δ²-enoyl-CoA The removed electrons are transferred to FAD, reducing it to FADH₂ Significance This reaction introduces a double bond between the second and third carbon atoms of the fatty acid chain The formation of FADH₂ is important because it can enter the electron transport chain to generate ATP 103 Step 2: Hydration Substrate trans-Δ²-enoyl-CoA, which was produced in the previous step Enzyme The enzyme that catalyzes this reaction is enoyl-CoA hydratase Reaction Water (H₂O) is added to the double bond of trans-Δ²-enoyl-CoA This hydration converts the double bond into a hydroxyl group Resulting in the formation of L-3-hydroxyacyl-CoA Significance The addition of water adds a hydroxyl group (–OH) to the β-carbon of the fatty acid chain, preparing it for the next oxidation step L-3-hydroxyacyl-CoA is a crucial intermediate that will undergo further processing in the subsequent steps of β-oxidation 104 Step 3: Second Dehydration Substrate 3-hydroxyacyl-CoA, which was produced in the previous step Enzyme The enzyme that catalyzes this reaction is 3-hydroxyacyl-CoA dehydrogenase Reaction The hydroxyl group (–OH) on the β-carbon of L-3-hydroxyacyl-CoA is oxidized to a carbonyl group (C=O) NAD⁺ is reduced to NADH Resulting in the formation of 3-ketoacyl-CoA Significance This oxidation step is crucial for preparing the substrate for the next reaction in beta-oxidation The production of NADH is important for cellular energy metabolism, as NADH can enter the electron transport chain to generate ATP 105 Step 4: Thiolysis Substrate 3-ketoacyl-CoA, which was produced in the previous step Enzyme The enzyme that catalyzes this reaction is thiolase Reaction 3-ketoacyl-CoA is cleaved by the addition of a CoA molecule The β-keto group is hydrolyzed Resulting in the production of acetyl-CoA and a new, shorter acyl-CoA Significance Thiolysis is a critical step that not only produces acetyl-CoA, but also regenerates a fatty acyl-CoA that can continue to be oxidized This step allows for the continuous breakdown of fatty acids, ultimately leading to energy production 106 Regulation of β-Oxidation 1. Substrate Availability Free Fatty Acid Levels: Higher concentrations promote beta-oxidation 2. Hormonal Regulation Insulin: Inhibits beta-oxidation; promotes fatty acid storage Glucagon: Stimulates beta-oxidation during fasting; promotes lipolysis 3. Enzyme Regulation CPT I (Carnitine Palmitoyltransferase I) Inhibited by malonyl-CoA; controls entry of fatty acids into mitochondria Acyl-CoA Synthetase: Regulated by substrate availability and energy status 4. Energy Status NADH/FADH₂ Levels: High levels inhibit the electron transport chain ATP Levels: Elevated ATP signals sufficient energy, inhibiting oxidation 5. Nutritional Status Fasting State: Upregulates beta-oxidation for energy Fed State: Downregulates beta-oxidation; favors carbohydrate metabolism 107 Introduction to Ketone Bodies Definition Ketone bodies are water-soluble molecules produced in the liver from fatty acids during periods of low carbohydrate availability Three main types: acetoacetate, beta-hydroxybutyrate, and acetone Ketone bodies are formed in the liver from fatty acids through a process called ketogenesis Production Occurs during fasting, prolonged exercise, or low-carbohydrate diets Fatty acids are converted to acetyl-CoA via β-oxidation, which is then transformed into ketone bodies Function Serve as an alternative energy source for tissues, especially the brain, when glucose is scarce Help maintain energy balance and metabolic flexibility 108 Regulation of Ketogenesis 1. Substrate Availability Free Fatty Acid Levels: Higher concentrations enhance ketone body formation Acetyl-CoA Levels: Increased acetyl-CoA from beta-oxidation drives ketogenesis 2. Hormonal Regulation Insulin: Decreases during fasting; inhibits ketogenesis by suppressing fatty acid release and promoting glucose utilization Glucagon: Increases during fasting; stimulates ketogenesis by promoting lipolysis and fatty acid mobilization 3. Enzyme Regulation CPT I (Carnitine Palmitoyltransferase I) Inhibited by malonyl-CoA; controls entry of fatty acids into mitochondria HMG-CoA Synthase: Key regulatory enzyme in ketogenesis 4. Energy Status NADH/NAD⁺ Ratio: High NADH levels favor the conversion of acetoacetate to beta-hydroxybutyrate ATP/ADP Ratio: Low energy status (high ADP) stimulates ketogenesis 5. Nutritional Status Fasting State: Elevated ketogenesis due to increased fatty acid oxidation and high acetyl-CoA levels Low-Carbohydrate Diets: Promotes ketogenesis by reducing glucose availability and increasing fatty acid mobilization 109 Disorders Associated with Lipid Metabolism 1. Hyperlipidemia Description: Elevated lipids (cholesterol and triglycerides) in the blood Types: ✓ Primary: Genetic disorders (e.g., familial hypercholesterolemia) ✓ Secondary: Related to conditions (e.g., diabetes, obesity) 2. Fatty Liver Disease Non-Alcoholic Fatty Liver Disease (NAFLD): Fat accumulation in the liver unrelated to alcohol Alcoholic Fatty Liver Disease: Caused by excessive alcohol consumption 3. Ketoacidosis Ketoacidosis: High ketone bodies in the blood, often seen in uncontrolled diabetes Symptoms: Nausea, vomiting, abdominal pain, confusion 4. Lipid Storage Disorders Gaucher Disease: Accumulation of glucocerebrosides due to enzyme deficiency Fabry Disease: Accumulation of globotriaosylceramide due to enzyme deficiency 110 Lecture 8 Hemoglobin 111 Part I: Introduction to Hemoglobin 112 What is hemoglobin? ❑ Definition Hemoglobin is a type of globular protein in red blood cells (erythrocytes) responsible for transporting oxygen in human body through blood. ❑ Basic components Globin Protein: characterized by its globular structure, protein subunit of hemoglobin Heme Group: a ring-like structure called porphyrin, which holds an iron atom at its center Iron Atom (Fe): The iron atom in the heme group can bind to oxygen ❑ Function Oxygen Transport ✓ Hemoglobin binds to oxygen in the lungs and carries it through the bloodstream to tissues and organs, where it is released for cellular use Carbon Dioxide Transport ✓ It also helps transport some carbon dioxide (a waste product of metabolism) from the tissues back to the lungs to be exhaled 113 Quaternary Structure of Hemoglobin ❑ Globin Chains Four protein subunits: 2 alpha (α) and 2 beta (β) chains Delta (δ) chains are present in a small amount in adults Gamma (γ) chains are found in fetal hemoglobin ❑ Heme Group Porphyrin Ring ✓ Each globin chain contains a heme group, which is a ring-like structure called porphyrin Iron Atom ✓ The iron atom in each heme group is essential for oxygen binding ✓ Each iron atom can bind one molecule of oxygen (O₂) ✓ One hemoglobin molecule, with four heme groups, can bind up to four oxygen molecules ❑ Oxygen Binding Oxygen-Binding Sites ✓ The binding sites on hemoglobin are specifically the iron atoms within the heme groups ✓ This binding is reversible, allowing hemoglobin to pick up oxygen in the lungs Cooperative Binding ✓ Binding of one oxygen molecule increases the affinity of the remaining binding sites for oxygen 114 Roles of Hemoglobin 1. Oxygen Transport Binds to oxygen in the lungs and transports it to tissues throughout the body Each hemoglobin molecule can carry up to four oxygen molecules 2. Carbon Dioxide (CO2) Transport Transports CO2 from tissues back to the lungs for exhalation About 20-25% of carbon dioxide binds to hemoglobin as carbaminohemoglobin 3. pH Regulation (Buffering) Hemoglobin helps maintain blood pH by binding to protons (H⁺ ions) Reduce changes in acidity during metabolic processes 115 Part II: Heme Synthesis 116 What is Heme Synthesis? ❑ Definition The biochemical process of producing heme, an iron-containing compound essential for various physiological functions Heme is a crucial component of hemoglobin, myoglobin, cytochromes, and other heme-containing proteins ❑ Importance of Heme Synthesis Oxygen Transport ✓ Heme is important for oxygen binding in hemoglobin and myoglobin Electron Transport ✓ Heme plays a key role in electron transport chains in mitochondria, crucial for cellular respiration Enzymatic Functions ✓ Heme-containing enzymes are involved in various metabolic processes, including drug metabolism and detoxification 117 Heme Synthesis Pathway https://www.youtube.com/watch?v=Q_WkjA4ZcjY 118 Regulation of Heme Synthesis Pathway 1. Aminolevulinic Acid Synthase (ALAS) Rate-limiting Enzyme: First step in heme synthesis Regulated by Heme: Negative feedback inhibition when heme levels are sufficient Iron Influence: Low iron levels can enhance ALAS activity to increase ALA production 2. Aminolevulinic Acid Dehydratase (ALAD) Inhibition by Lead (Pb2+): Sensitive to lead, disrupting heme synthesis 3. Porphobilinogen Deaminase Feedback Mechanisms: Influenced by the accumulation of pathway intermediates 4. Uroporphyrinogen Decarboxylase Substrate Availability: Activity dependent on the availability of uroporphyrinogen III 5. Ferrochelatase Iron Availability: Regulated by the availability of iron for incorporation into protoporphyrin IX Inhibition by Lead (Pb2+): In cases of lead poisoning, lead inhibits ferrochelatase activity 119 Part III: Heme Degradation 120 What is Heme Degradation? ❑ Definition Heme degradation is the biochemical process by which heme, an iron-containing compound, is broken down into simpler molecules This process is essential for recycling iron and eliminating toxic byproducts produced from heme metabolism ❑ Importance of Heme Degradation Iron Recycling ✓ This process recycles iron for the synthesis of new heme-containing proteins Detoxification ✓ It helps eliminate excess heme and prevent toxicity Physiological Role ✓ Heme will be broken down into bilirubin; Bilirubin has antioxidant properties, contributing to cellular protection 121 Overview of Heme Degradation Damaged red blood cells release free heme Free heme is converted to biliverdin by heme oxygenase-1 (Hmox1) Generates carbon monoxide (CO) and iron (Fe²⁺) Biliverdin is in turn converted to bilirubin by biliverdin reductase A (BLVR-A) 122 Key Steps of Heme Degradation 1. Conversion of Heme to Biliverdin Catalyzed by heme oxygenase Heme is cleaved by heme oxygenase to produce: ✓ Biliverdin: A green pigment ✓ Carbon Monoxide (CO): A gas that has signaling functions in the body ✓ Free Iron (Fe²⁺): Released for recycling 123 Key Steps of Heme Degradation 2. Reduction of Biliverdin to Indirect Bilirubin Catalyzed by biliverdin reductase Biliverdin is reduced to indirect bilirubin (unconjugated bilirubin), a yellow pigment Indirect bilirubin is lipid-soluble and not water-soluble Indirect bilirubin can cross cell membranes but difficult for the body to excrete Indirect bilirubin binds to albumin in the bloodstream for transport to the liver (Indirect Bilirubin) 124 Key Steps of Heme Degradation 3. Conjugation of Indirect Bilirubin in the Liver In the liver, indirect bilirubin undergoes conjugation with glucuronic acid Facilitated by the enzyme UDP-glucuronosyltransferase This forms direct bilirubin (conjugated bilirubin or bilirubin diglucuronide), making it more water-soluble 4. Excretion into Bile Direct bilirubin is excreted into bile stored in the gallbladder, and eventually released into the intestines 125 Key Steps of Heme Degradation 5. Intestinal Metabolism In the intestines, direct bilirubin is further metabolized by gut bacteria into: ✓ Urobilinogen: Reabsorbed into the bloodstream and excreted in urine, giving urine its yellow color ✓ Stercobilin: Contributes to the brown color of feces 126 Regulation of Heme Degradation 1. Enzyme Regulation Heme Oxygenase (HO) ✓ Heme Oxygenase 1 (HO-1) can be induced by various factors such as heme itself, heavy metals, and cytokines, enhancing heme degradation during stress Biliverdin Reductase ✓ Activity can be influenced by the availability of biliverdin and other substrates 2. Substrate Availability The availability of heme serves as a primary regulator Increased levels of heme can stimulate its own degradation through feedback mechanisms The concentration of glucuronic acid affects the conjugation process of bilirubin in the liver 3. Hormonal Regulation Hormones like cortisol can influence the expression of heme oxygenase in response to stress 127 Part IV: Hemoglobin Abnormalities 128 Anemia ❑ Definition A condition characterized by a deficiency of red blood cells (RBCs) or hemoglobin in the blood ❑ Common Types of Anemia Sickle Cell Anemia ✓ Genetic disorder caused by mutation in β-globin gene, leading to the production of abnormal hemoglobin (HbS) Thalassemia ✓ Genetic disorder caused by genetic mutations in the alpha or beta-globin genes Iron-Deficiency Anemia ✓ Caused by insufficient iron Vitamin Deficiency Anemia ✓ Often due to lack of vitamin B12 or folate Chronic Disease Anemia ✓ Associated with chronic illnesses (e.g., cancer, kidney disease) Aplastic Anemia ✓ Bone marrow fails to produce enough RBCs Hemolytic Anemia ✓ RBCs are destroyed faster than produced 129 What is Jaundice? A medical condition characterized by the yellowing of the skin, mucous membranes, and the whites of the eyes due to elevated levels of bilirubin in the blood ❑ Causes Hemolysis ✓ Increased breakdown of red blood cells Liver Dysfunction ✓ Impaired conjugation and excretion of bilirubin Biliary Obstruction ✓ Blockage of bile flow ❑ Symptoms Yellowing of skin and eyes Dark urine Pale stools 130 Types of Jaundice 1. Pre-hepatic Jaundice Cause: Excessive hemolysis of red blood cells (RBCs) Mechanism: Increased production of unconjugated (indirect) bilirubin Examples: Hemolytic anemia, infections (e.g., malaria) 2. Hepatic Jaundice Cause: Liver dysfunction affecting bilirubin processing Mechanism: Impaired conjugation or reduced excretion into bile Examples: Hepatitis, cirrhosis 3. Post-hepatic Jaundice Cause: Obstruction of bile flow from the liver to the intestine Mechanism: Accumulation of conjugated (direct) bilirubin in the blood Examples: Gallstones, bile duct strictures, pancreatic cancer 131 How Does Hemolysis Cause Jaundice? ❑ Hemolysis Hemolysis is the destruction of red blood cells (RBCs), resulting in the release of hemoglobin into the bloodstream Can be caused by various factors, including autoimmune disorders, infections, certain medications, and genetic conditions ❑ Breakdown of Hemoglobin When RBCs are destroyed, hemoglobin is released and broken down into: ✓ Heme: Converted to biliverdin and then to unconjugated bilirubin ✓ Globin: Broken down into amino acids ❑ Increased Bilirubin Production Excessive hemolysis leads to increased production of unconjugated bilirubin The liver may become overwhelmed and unable to process all the bilirubin efficiently ❑ Bilirubin Accumulation Elevated levels of unconjugated bilirubin in the bloodstream occur when the liver cannot conjugate and excrete it quickly enough ❑ Development of Jaundice The accumulation of bilirubin results in jaundice 132 Lecture 9 Enzyme I: Introduction to Enzymes 133 Part I: Introduction to Enzyme 134 What is Enzyme? ❑ Definition Enzymes are proteins that act as biological catalysts by accelerating chemical reactions Enzymes are known to catalyze more than 5,000 biochemical reaction types Not all biological catalysts are enzymes Some RNAs can also serve as catalysts and are known as ribozymes ❑ Importance Speed up biochemical reactions by lowering activation energy Highly specific to substrates, ensuring precise metabolic control Control metabolic pathways, responding to cellular needs Play essential roles in various biological processes Serve as diagnostic markers for diseases 135 Substrate and Product Substrate A substrate is the molecule that an enzyme acts on, the reactant of biochemical reaction It is the starting material that gets changed during a chemical reaction Product A product is the end result of an enzymatic reaction It is the molecule(s) formed after the substrate has been transformed by the enzyme Enzyme Speed up the chemical reactions 136 Structure of Enzyme Proteins Most enzymes are proteins made up of long chains of amino acids Active Site This is a specific region on the enzyme where substrate molecules bind The active site has a unique shape and chemical environment that facilitates the conversion of substrates into products 137 Part II: Mechanism of Enzyme 138 How Does Enzyme Work? ❑ Formation of Enzyme-Substrate Complex When a substrate binds to the active site, it forms an enzyme-substrate complex This binding can induce changes in the enzyme's structure (induced fit), enhancing the reaction ❑ Catalysis Enzymes lower the activation energy required for a reaction to occur Making it easier for substrates to be converted into products, this may: ✓ Stabilize the transition state ✓ Bring substrates closer together in the correct orientation ✓ Provide an optimal environment for the reaction 139 How Does Enzyme Work? ❑ Product Release After the reaction occurs, the products are released from the active site, regenerating the enzyme for subsequent reactions 140 Factors Affecting Enzyme Activity Temperature ✓ Each enzyme has an optimal temperature range at which it exhibits maximum activity ✓ For many human enzymes, this is around 37°C ❑ Effects of Low Temperature ✓ Lower temperature usually reduces enzyme activity, resulting in slower reaction rates ✓ Enzymes may become less effective but typically remain stable ❑ Effects of High Temperature ✓ Higher temperatures initially increase the enzyme activity ✓ However, beyond the optimal temperature, enzymes can denature ✓ Denatured enzymes cannot catalyze reactions effectively 141 Factors Affecting Enzyme Activity pH ✓ Enzymes also have an optimal pH range, and deviations can affect their activity ✓ Too high or too low pH cause enzymes to denature and lose their activities ✓ Enzyme activity increases to a peak at the optimal pH, then declines on either side as conditions become unfavorable 142 Factors Affecting Enzyme Activity Substrate Concentration 1. Initial Increase in Activity As substrate concentration increases, the reaction rate also increases More substrate molecules lead to more opportunities for the enzyme to interact with them 2. Saturation Point (Maximum Rate Reached) Eventually, all enzyme active sites become occupied At this point, adding more substrate does not increase the reaction rate Maximum (Unchanged) Increases 143 Factors Affecting Enzyme Activity Ionic Strength ✓ Influences enzyme stability and substrate binding; optimal ionic strength enhances activity Cofactors ✓ Many enzymes require additional cofactors to be active Activators and Inhibitors ✓ Activators are molecules that increase enzyme activity ✓ Inhibitors are molecules that decrease enzyme activity Allosteric Regulation ✓ Regulatory molecules bind to sites other than the active site, influencing enzyme activity either positively or negatively 144 Part III: Classification of Enzyme 145 Classification of Enzymes The International Union of Biochemistry and Molecular Biology (IUBMB) categorizes enzymes into six main classes: 1. Oxidoreductase 2. Transferase 3. Hydrolase 4. Lyase 5. Isomerase 6. Ligase 146 Oxidoreductase Definition Oxidoreductases are a class of enzymes that catalyze oxidation-reduction (redox) reactions Function Oxidoreductases facilitate the transfer of electrons from a donor (reductant) to an acceptor (oxidant) This process can involve the removal of hydrogen atoms (oxidation) or the addition of oxygen (reduction) 147 Oxidoreductase Main Types of Oxidoreductases ❑ Dehydrogenase Catalyze the removal of hydrogen atoms (or electrons) from substrates Example: NADH Dehydrogenase, Succinate Dehydrogenase ❑ Oxidase Transfer electrons to molecular oxygen, producing water or hydrogen peroxide Example: Cytochrome c Oxidase, Galactose Oxidase ❑ Reductase Catalyze the reduction of substrates, often involving the addition of electrons or hydrogen Example: NADPH Reductase, Cytochrome P450 Reductase ❑ Peroxidase Catalyze the reduction of peroxides, particularly hydrogen peroxide, using electron donors Example: NADH Peroxidase, Horseradish Peroxidase (HRP) ❑ Catalase Decompose hydrogen peroxide (H2O2) into water and oxygen, protecting cells from oxidative damage 148 Transferase Definition Transferases are a class of enzymes that catalyze the transfer of specific functional groups (such as methyl, phosphate, or amino groups) from one molecule to another Function Facilitate the transfer of functional groups, which can alter the structure and function of substrates Play critical roles in metabolic pathways Example aminotransferase 149 Transferase Main Types of Transferases ❑ Aminotransferase (Transaminase) Transfers amino groups (–NH₂) from amino acids to α-keto acids Example: Alanine aminotransferase (ALT) ❑ Phosphotransferase Transfers phosphate groups from one molecule to another Example: Kinases, which transfer of phosphate groups from high-energy, phosphate-donating molecules to specific substrates ❑ Glucosyltransferase Transfers glucose or other sugar moieties to substrates Example: glycogen synthase, responsible for synthesis of glycogen ❑ Methyltransferase Transfers methyl groups (–CH₃) to various substrates Example: DNA methyltransferase, involved in gene regulation ❑ Acyltransferase Transfers acyl groups (RCO–) from donor molecules to acceptors Example: Acetyl-CoA transferase, involved in fatty acid metabolism ❑ Transketolase Transfer of a ketol group from a ketose donor to an aldose acceptor ❑ Transaldolase transfer of an aldol group from a ketose donor to an aldose acceptor 150 Hydrolase Definition Hydrolase is a class of enzyme that catalyzes the hydrolysis of chemical bonds, meaning it facilitates the breakdown of larger molecules into smaller ones by adding water Function Hydrolases are involved in digestion, metabolism, and various biochemical pathways They break down proteins, lipids, nucleic acids, and carbohydrates Hydrolase 151 Hydrolase Main Types of Hydrolase ❑ Lipase Catalyzes the hydrolysis of lipids into fatty acids and glycerol Example: Pancreatic lipase, Lysosomal lipase ❑ Phosphatase Removes phosphate groups from molecules, including proteins and nucleotides Example: Pyruvate dehydrogenase phosphatase, Protein Phosphatase 1 (PP1) ❑ Nuclease Hydrolyzes nucleic acids (DNA and RNA) into nucleotides Example: Ribonuclease (RNase), Deoxyribonuclease (DNase) ❑ Protease Breaks down proteins into smaller peptides or amino acids Example: Trypsin, Pepsin, Caspase ❑ Glycosidase Hydrolyzes glycosidic bonds in carbohydrates, breaking them down into simpler sugars Example: Amylase (Hydrolyzes starch), Lactase (Breaks down lactose into glucose and galactose) 152 Lyase Definition Lyase is a class of enzyme that catalyzes the breaking (an elimination reaction) of various chemical bonds using mechanisms other than hydrolysis and oxidation Function Lyases catalyzes the breaking (an elimination reaction) of various chemical bonds by means other than hydrolysis (a substitution reaction) and oxidation Often forming a new double bond or a new ring structure Some lyases can also add functional groups to double bonds, forming new compounds Example 153 Lyase Main Types of Lyase ❑ Decarboxylase Catalyze the removal or addition of a carboxyl group (–COOH) from substrates Example: Pyruvate decarboxylase, Histidine decarboxylase ❑ Dehydratase Cleave carbon–oxygen bonds, catalyze the removal of water (H₂O) from a substrate Example: Serine dehydratase, 3-Hydroxyacyl-CoA Dehydratase ❑ Cyclase Catalyzes a chemical reaction to form a cyclic compound Example: Adenylyl cyclase, forms cyclic AMP from ATP 154 Isomerase Definition Isomerase is a class of enzymes that catalyze the conversion of a molecule into one of its isomers Function Isomerases catalyze reactions that involve the rearrangement of atoms within a molecule Result in the formation of structural isomers or stereoisomers Example 155 Isomerase Main Types of Isomerase ❑ Isomerase General category for enzymes that facilitate structural rearrangements without specifying the type of isomerization Example: Enoyl-CoA isomerase, Glucose-6-phosphate isomerase, Topoisomerase ❑ Mutase Transfer a functional group from one position to another within the same molecule Example: Phosphoglycerate Mutase, Phosphoglucomutase ❑ Racemase and Epimerase Convert one stereoisomer into another Example: Lactate Racemase, Glucose-6-Phosphate Epimerase 156 Ligase Definition Ligase is a class of enzyme that catalyzes the joining of two molecules through the formation of a new chemical bond Function Plays a crucial role in the continuity of nucleic acids and in various metabolic processes Important for maintaining genetic integrity and facilitating numerous biochemical reactions Example 157 Ligase Main Types of Ligase ❑ DNA Ligase Catalyze the joining of DNA strands by sealing nicks in the sugar-phosphate backbone Example: DNA Ligase I ❑ RNA Ligase Join RNA fragments, which is important for certain RNA processing events Example: T4 Ligase ❑ Aminoacyl-tRNA Synthetase Catalyze the attachment of amino acids to their corresponding tRNAs, forming aminoacyl- tRNA complexes Example: Phenylalanyl-tRNA synthetase, joins phenylalanine to its corresponding tRNA ❑ Other Ligase Catalyze the joining of various biomolecules in metabolic pathways Example: Glutamine Synthetase, Succinyl-CoA Synthetase 158 Part IV: Cofactor 159 Cofactor Definition Cofactors are non-protein chemical compounds that assist enzymes in catalyzing biochemical reactions Types ❑ Coenzyme ❑ Metal Ion ❑ Prosthetic Group Function ✓ Provide catalytic support ✓ Stabilize enzyme structure ✓ Facilitate electron transfer ✓ Enhance substrate binding ✓ Carriers for functional groups ✓ Regulate enzyme activity 160 Coenzyme Definition Coenzyme is a type of non-protein organic molecule that binds to an enzyme and is essential for its activity Key Characteristics of Coenzymes Organic Molecules ✓ Coenzymes are usually small organic compounds, often derived from vitamins (e.g., NAD⁺ from vitamin B3, FAD from vitamin B2) Temporary Association ✓ Temporary Association: Coenzymes typically bind to the enzyme temporarily and may be released after the reaction, often being regenerated in the process Role in Catalysis ✓ They assist in catalyzing reactions by participating in the transfer of electrons, atoms, or functional groups between substrates Examples of Coenzymes NAD⁺: Involved in redox reactions, crucial for cellular respiration FAD: Electron carrier in various metabolic reactions Coenzyme Q: Plays critical roles in cellular metabolism and energy production 161 Metal Ion Definition Metal ions are inorganic and can be essential for the activity of many enzymes Role of Metal Ions as Cofactors Catalytic Role ✓ Stabilize negative charges on substrates or intermediates, facilitating the formation or breaking of chemical bonds Structural Role ✓ Help maintain the three-dimensional structure of enzymes, contributing to the enzyme's stability and active site configuration Electron Transfer ✓ Some metal ions can participate in redox reactions, acting as electron carriers during enzymatic processes Function Zinc (Zn²⁺) ✓ Acts as a structural stabilizer and is involved in catalytic activity in enzymes like zinc-dependent proteases Magnesium (Mg²⁺) ✓ Essential for the activity of many kinases and polymerases, stabilize phosphate groups in ATP and nucleic acids Iron (Fe²⁺/Fe³⁺) ✓ Involved in oxygen transport (hemoglobin), electron transfer (cytochromes), and catalysis (iron- containing enzymes like catalase) 162 Prosthetic Group Definition A prosthetic group is a non-polypeptide unit that is tightly or covalently bound to an enzyme, essential for its biological activity Prosthetic groups remain attached throughout the enzyme's catalytic process, unlike coenzymes that can be released Key Characteristics ❑ Tight Binding ✓ Firmly attached to the enzyme, often via covalent bonds, ensuring they remain part of the enzyme's structure during reactions ❑ Function ✓ Play essential roles in the enzyme's activity, often participating directly in the catalytic process or stabilizing the enzyme's structure Examples ❑ Heme ✓ A prosthetic group found in hemoglobin and myoglobin, crucial for oxygen transport ❑ Flavin Mononucleotide (FMN) ✓ Involved in various redox reactions, particularly in the electron transport chain 163 Part V: Proenzyme and Isoenzyme 164 Proenzyme (Zymogen) Definition Proenzymes, also known as zymogens, are inactive precursors of enzymes that require activation to function Key Characteristics ❑ Activation Mechanism ✓ Converted to active forms through proteolytic cleavage (removal of specific peptide segments) or chemical modifications ❑ Purpose ✓ Regulate enzymatic functions ✓ Ensures that enzymes are activated only when needed Examples ❑ Trypsinogen ✓ The inactive form of the enzyme trypsin, activated in the small intestine ❑ Pepsinogen ✓ The inactive precursor of pepsin, activated in the acidic environment of the stomach ❑ Procaspase ✓ Inactive precursors of caspases, activated through proteolytic cleavage, often in response to apoptosis 165 Isoenzyme ❑ Definition Isoenzymes are enzymes that differ in amino acid sequence but catalyze the same chemical reaction They may arise due to variations in gene expression, alternative splicing of mRNA or chemical modification of proteins after translation ❑ Key Characteristics Isoenzymes may vary in amino acid sequence, which can affect their physical and chemical properties They can have different affinities for substrates and different rates of reaction Isoenzymes may respond differently to inhibitors, activators, and allosteric regulators ❑ Significance Enable different tissues to perform specific functions based on metabolic needs Provide flexibility in regulating metabolic pathways in response to physiological changes Expressed at various developmental stages, satisfying the organism's changing requirements 166 Lecture 10 Enzyme II: Enzyme Kinetics and Inhibitors 167 Turnover Number or Catalytic Constant (kcat) Reaction The enzymatic reaction can be generally expressed as follows: kcat 𝐸 + 𝑆 ⇌ 𝐸𝑆 ⇀ 𝑃 + 𝐸 E = Enzyme S = Substrate ES = Enzyme-substrate complex P = Product kcat = catalytic constant Turnover Number or Catalytic Constant (kcat) Represents the maximum number of substrate molecules converted to product by one enzyme molecule per second when the enzyme is fully saturated Units: Expressed in reciprocal seconds (s⁻¹) Important of measure enzyme efficiency 𝑽𝒎𝒂𝒙 𝒌𝒄𝒂𝒕 = [𝑬𝟎] Vmax : Maximum reaction velocity, when enzyme is saturated with substrate [𝑬𝟎]: Total enzyme concentration 168 Michaelis-Menton Equation Describes the rate of enzymatic reactions by relating reaction velocity (V) to substrate concentration [S] Vmax [𝑺] V= 𝑲𝒎 + [𝑺] V: Reaction velocity, rate of product formation Vmax : Maximum reaction velocity, when enzyme is saturated with substrate [𝑺]: Substrate concentration Vmax 𝑲𝒎: Michaelis constant , substrate concentration when V = 𝟐 Vmax If 𝑲𝒎 = 𝑺 , then V = , 𝟐 Vmax So 𝑲𝒎 is also the substrate concentration at the point when V = 𝟐 169 Enzymatic Reaction Velocity (V) vs. Substrate Concentration [S] Low Substrate Concentration Reaction velocity increases linearly with substrate concentration High Substrate Concentration Reaction velocity approaches Vmax as the enzyme becomes saturated 170 Enzyme Efficiency Definition Enzyme efficiency refers to the effectiveness with which an enzyme converts a substrate into a product Efficiency Measurement Catalytic efficiency is calculated as: 𝒌𝒄𝒂𝒕 𝑲𝒎 Higher values indicate higher catalytic efficiency of enzymes Factors Influencing Efficiency Substrate Concentration Temperature & pH Inhibitors 171 Classification of Enzyme Inhibitor Reversible Inhibitor Reversible inhibitors bind to enzymes non-covalently and can be removed, restoring enzyme activity ❑ Main Types of Reversible Inhibitor Competitive Inhibitors Non-Competitive Inhibitors Uncompetitive Inhibitors Irreversible Inhibitor Irreversible inhibitors bind covalently to the enzyme, permanently inactivating it 172 Competitive Inhibitors Definition A competitive inhibitor is a molecule that competes with a substrate for binding to the active site of an enzyme Mechanism of Inhibition Bind to the active site of the enzyme Prevent substrate binding Inhibition can be reversed by increasing substrate concentration 173 Competitive Inhibitors Effect on Enzyme Kinetics Km : Increases, indicating higher substrate concentration is needed Vmax : Remains unchanged 174 Non-Competitive Inhibitors Definition Non-competitive inhibitors are substances that bind to an enzyme at a site other than the activ