Electronic Structure of the Atom PDF
Document Details
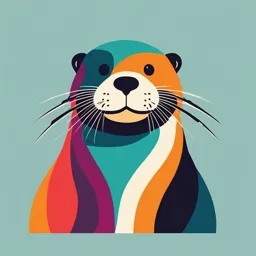
Uploaded by ImportantArtInformel495
Sutton Park School
Tags
Summary
This document is a presentation about the arrangement of electrons in atoms. Topics discussed include Bohr's model, energy levels, spectra, and atomic orbitals.
Full Transcript
Arrangement of Electrons in the Atom Chapter 3 Learning Outcomes Understand Bohr’s Study of Spectra Understand continuous, absorption and emission spectra and be able to distinguish between them Understand the flame tests Be able to define and understand; Energy Lev...
Arrangement of Electrons in the Atom Chapter 3 Learning Outcomes Understand Bohr’s Study of Spectra Understand continuous, absorption and emission spectra and be able to distinguish between them Understand the flame tests Be able to define and understand; Energy Levels, Ground State, Excited State Distinguish between the Lyman, Balmer and Paschen series Be able to define and understand; Energy sublevels, Heisenberg’s Uncertainty Principal, Atomic orbitals Know about Erwin Schrodinger Bohr’s Study of Spectra Neils Bohr was one of the first people to provide an insight into the arrangement of electrons in atoms. His model came from a study of spectra. Spectra: - When white light is passed through a glass prism, the white light is broken up into an array of colours called a spectrum. - The spread of colours is called a continuous spectrum, e.g. a rainbow. If you replace the source of white light with a hydrogen discharge tube (a glass tube filled with Hydrogen gas at low pressure) and pass electricity through it, instead of seeing a continuous spectrum an Emission Line Spectrum is observed. Emission Line Spectrum: Series of narrow coloured lines against a dark background Spectrometer Spectra are usually studied using an instrument called a spectrometer. Spectrometers are used to measure wavelengths of light Do not need to know this diagram Spectroscopes Simply used to observe spectra Do not need to know this diagram White light is a mixture of light of all wavelengths, and a spectroscope manages to separate these colours from white light. When white light is analysed using a spectroscope, a continuous band of colours or a ‘continuous spectrum’ is seen, ranging from red to violet. Note: Each element has its own unique emission line spectrum. Line spectrums are often used to identify elements. We use this application in our everyday lives, e.g. sodium street lamps are yellow, neon advertising signs glow red. When light emitted by other elements, such as sodium, mercury or neon, was studied, it was found that these elements also produced emission line spectra. https://www.youtube.com/watch?v=Ki3C3lO sj6E To carry out flame tests with salts of lithium, sodium, potassium, barium, strontium and copper Mandatory Experiment Brief introduction to flame tests When certain elements are heated they give out coloured light; - potassium = Lilac - strontium = red. Other elements that are gases or those that are easily vaporised also give out a characteristically coloured flame / light when electricity is passed through them, e.g. sodium street lights. Results Metal Colour Lithium Crimson Potassium Lilac Barium Green Strontium Red Copper Blue-green Sodium Yellow Explaining the evidence: Bohrs Theory It was emission spectra that led Neils Bohr to an understanding of how electrons are arranged inside the atom. Bohr realised that any model of the atom must explain: i) Why emission spectra are line rather than continuous spectra ii) Why the emission spectrum of each element is unique to that element. Bohr’s Theory Up to the time when Bohr proposed his theory, scientists believed that electrons could have any energy value. Bohr introduced the idea of quantisation of energy, i.e. that an electron can only have a fixed amount of energy (or an electron is restricted to having only certain energy values or levels of energy). This fixed amount of energy is called a quantum of energy. Bohr suggested a way to relate the emission line spectrum of hydrogen to the structure of the atom of hydrogen. He provided ‘spectroscopic evidence for the existence of energy levels’. Bohr’s Theory may be summarised as follows…. - Electrons move around the nucleus in a series of fixed paths, called orbits. - Electrons in an orbit have a fixed amount of energy. (These orbits are also called energy levels). - Energy levels are represented by the letter ‘n’. - The lowest energy level is n=1 (this is closest to the nucleus). - The energy of an electron in a particular orbit is quantised. When atoms receive energy, electrons jump to higher energy levels and drop back down emitting light. Energy Levels in Atoms An energy level is a region of definite energy within the atom that electrons can occupy. - Electrons occupy energy levels. - Each energy level has a different energy value. - The inner energy level (n=1), holds 2 electrons. As long as an electron is in a particular energy level, it neither gains or loses energy. This explains why electrons do not lose energy and fall into the nucleus. Atoms normally exist in the ground state, i.e. the electrons occupy the lowest available energy levels. The ground state of an atom is one in which the electrons occupy the lowest available energy levels. When an atom in its ground state is provided with energy (by electricity or heat), a specific amount of this energy is absorbed by the electrons and they jump to higher energy levels. The electrons are now in an excited state. Definition: The excited state of an atom is one in which the electrons occupy higher energy levels than those available in the ground state. Note: In some atoms of hydrogen, the electron may only be excited to the 2nd energy level, in others it may move to the third energy level. The energy absorbed is equal to the difference in energy between the lower energy level and the higher energy level. Electrons in the excited state are unstable and they fall back down to lower energy levels after a short period of time. As the electrons fall back down, the excess energy is released in the form of a photon of light which has a definite amount of energy. Since an electron can only fall back to a certain definite energy level, only fixed amounts of light energy are given off, i.e. light of a definite frequency is given off. The frequency of light emitted depends on the difference in energy between two energy levels and is given by: E2 – E1 = hf Energy of higher energy level Frequency of Energy of Planck’s light emitted lower energy Constant level The equation E2 – E1 = hf tells us that the energy difference is proportional to the frequency of the light emitted. The definite amount of energy emitted appears as a line of particular colour in the emission spectrum. Since only definite amounts of energy are emitted, this implies that electrons can occupy only definite energy levels. Each element has a unique emission line spectrum because each element has a different number of electrons and electron transitions. Excited Staten=4 Spectrum Excited Staten=3 Excited State unstable and drops back down UV Excited State But only as far as n=2 n = 2 this time V i Energy released as a photon s Frequency proportional i to energy drop b l e IR n=1 Ground State © SLSS Science Limerick Education Using Hydrogen as an example: - Electron in hydrogen is always in lowest energy level (n=1), a.k.a. the ground state. - When the ‘H’ atom is given energy, the electrons jumps to a higher energy level and is now in an ‘excited state’. - After a short time the electron drops back down, emitting energy in the form of light. Energy H H H The energy given out is the energy difference between the 2 energy levels: E = E2 – E1 The light energy given out appears as a line on the spectrum. An emission spectrum of ‘H’ always shows the same lines of definite frequency, proving that atoms can only emit definite amounts of energy which is proof for the existence of energy levels. Each element gives a unique line spectrum due to the fact that each element has a unique number and arrangement of electrons and so will have unique electron transitions. Hydrogen's emission spectrum has four colored lines. Since H has only one electron, this would seem impossible. How is it possible for hydrogen's emission spectrum to have four lines, when hydrogen has only one electron? Answer: Electrons can occupy different energy levels. When an electron goes from a high energy to a low energy level, it gives off the extra energy as a photon. This photon has a certain wavelength depending on the difference in energy of the levels. There may only be one electron, but it can go to any of those energy levels and then drop down and emit a photon. Spectral Series Elements not only emit visible light, but they also emit light in the UV and IR regions of the electromagnetic spectrum as well. The spectral series consists of the - Balmer series (all lines in visible region) - Lyman series (UV region) - Paschen series (IR region). When excited electrons drop from high energy levels to the ground state: Lyman (UV) When electrons drop from n=3 or greater to n=2: Balmer (Visible) When electrons drop from higher levels to n=3: Paschen (IR) Summary Electron normally in Ground State Energy supplied [ as heat or electricity] Electron jumps to higher energy level Now in Excited State Unstable Drops back to a lower level © SLSS Science Limerick Education Energy that was absorbed to make the jump up is now released as a photon Frequency depends on difference in energy levels [ E2 - E1 = hf ] h = Plank’s Constant f = frequency of light When electron falls to n = 1 level gives UV Range n = 2 level gives Visible Range n = 3,4 or 5 levels gives IR Range © SLSS Science Limerick Education Atomic Absorption Spectrometry (A.A.S.) Scientists studying spectra have also found that atoms can absorb light. If white light is passed through a gaseous sample of an element, it is found that light of certain wavelengths are missing i.e. Darks lines appear in the spectrum. This is called an atomic absorption spectrum. - The light that comes out of the sample is passed through a prism - Certain wavelengths are found to be missing. - These wavelengths are absorbed by the sample and they are found to correspond to the lines of colour in the emission spectrum of the sample. - Explanation: Atoms in their ground state absorb the same radiations as they emit in the excited state. Absorption Spectra Absorption spectra is the spectra observed after white light has been passed through the element. An absorption spectra is a continuous band of colour with lines of colour missing. Reason for the missing band of colour: - When atoms are excited, electrons absorb energy and jump from a lower energy level to a higher energy level. - The energy that is absorbed corresponds to the missing wavelength in an absorption spectra. Uses Emission Absorption Spectra Spectra Identification of elements Identification of elements Sodium street lamps Concentration of elements Fireworks Energy Sublevels As spectra developed, more sophisticated spectrometers began to develop. Scientists were able to look more closely at lines formed in emission spectra. They noticed: - What originally appeared to be a single line actually consisted of a number of lines close together. - These lines were so close together that they could not be from electrons dropping from different energy levels. - Therefore, each main energy level (except the first) must be split into a number of sublevels, all of which were close in energy. Scientists labelled the sublevels using the letters s, p, d and f. S sublevel – lowest energy (closest to nucleus) P sublevel – 2nd lowest D sublevel – 2nd highest F sublevel – highest energy Wave Nature of the Electron 1923: Louis de Broglie said electrons have ‘wave’ like properties (electrons do not travel in precise paths). Louis De Broglie continued… Based on his calculations, he suggested that fairly large objects (such as tennis balls), the wave motion would be difficult to detect. But for small particles, like electrons, it would be easy. This idea that electrons can move in a wave like manner is known as ‘wave particle duality’. The wave like movement of electrons was treated mathematically by a German physicist called Werner Heisenberg. 1927: Heisenberg’s Uncertainty Principal It is not possible to determine at the same time the exact position and velocity of an electron - We can only describe the probability of finding an electron in a particular position. - Heisenberg stated that Bohrs model of the atom was incorrect as it showed electrons moving with certain speeds in orbits of fixed distances from the nucleus. Heisenberg continued… It is clear therefore that the idea of an electron moving in a fixed orbit around the nucleus had to be modified. If an electron is not travelling in a fixed path, then we can only talk about the probability of finding an electron at a particular position inside the atom. Schrödinger's Probability Equation 1926 Due to the uncertainty of the position of an electron we should refer to the ‘probability’ of finding an electron in a particular location within an atom. Probability is calculated using mathematical equations developed by Schrodinger. It was decided that: an ‘atomic orbital’ is a region in space AROUND THE NUCLEUS of an atom in which there is a high probability of finding an electron. Limitations of Bohrs Theory This theory worked well for the emission spectrum of Hydrogen, but not for bigger atoms with more electrons as it failed to account for the many lines in their emission spectra's. Bohrs theory did not take into account that the electron had a wave like motion. Therefore the picture of an electron moving in a precise path at a precise distance from the nucleus is incorrect. Bohrs theory did not take into account the presence of sublevels. Atomic Orbitals There are a number of different types of orbitals. S-orbitals: - Each S sublevel contains 1s orbital - This holds 2 electrons. - Spherical shape. - 1s, 2s,3s,4s... P-orbitals - Each P sublevel contain 3p orbitals (Px, Py, Pz) - Each contain 2 electrons - Dumb-bell shaped - Higher in energy than s orbitals. D-orbitals: - D sublevels contain 5 orbitals which hold 2 electrons each. Complex shape. Higher in energy than p orbitals. F-orbitals - Complex shape. Higher in energy than d orbitals. n=1 energy level: - 1 s orbital (holds 2 electrons) n=2 energy level: - 4 orbitals (2s,2px,2py,2pz) (8 electrons in total) n=3 energy level: - 9 orbitals (3s, 3px,3py,3pz and five 3d orbitals) (18 electrons in total). n=4 energy level: - 18 orbitals (4s, 4px,4py,4pz, five 4d orbitals and seven 4f orbitals). Capacity If a number (n) is assigned to each energy level then the electron capacity is 2n2. i.e. Energy Level 1 = 2(1)2 = 2e- 2 = 2(2)2 = 8e- On closer examination of the spectra it was found that energy levels were made up of sublevels. n=1 1 sublevel n=2 2 sublevels n=3 3 sublevels n=4 4 sublevels Sub-levels were given the initials s,p,d,f Note: The 2nd energy level has an ‘s’ and a ‘p’ sublevel. The ‘s’ sublevel has 1 ‘s’ orbital The ‘p’ sublevel has 3 ‘p’ orbitals; px, py and pz Electronic Configurations of Atoms and Ions A group of orbitals that all have the same energy is called an energy sub-level. For example, the 2px, 2py and 2pz orbitals make up the 2p sublevel. An energy sublevel is a group of atomic orbitals within an atom, all of which have the same energy. Note: 3d sublevel is higher in energy than the 4s sublevel. Not more than two electrons can occupy an orbital at one time and the Aufbau Principal states that electrons will occupy the lowest energy sublevel available. Therefore, electrons will occupy the 4s sublevel before the 3d sublevel. When writing electronic configurations, follow the following pattern: 1s2, 2s2, 2p6, 3s2, 3p6, 4s2, 3d10, 4p6, 4d10 Examples: Electronic configuration of hydrogen (atomic number = 1): 1s1 Electronic configuration of Lithium (atomic number = 3): 1s2 ,2s1 Electronic configuration of Sodium (atomic number = 11): 1s2 ,2s2 ,2p6 ,3s1 Exceptions This arrangement works for all but 2 of the first 36 elements: - Copper: Expected1s22s22p63s23p64s23d9 Actual 1s22s22p63s23p64s13d10 - Chromium: Expected1s22s22p63s23p64s23d4 Actual 1s22s22p63s23p64s13d5 Reason: These unexpected configurations are due to the extra stability, in the case of chromium, of a structure with a half-filled 3s sublevel, and in the case of copper with a full 3d sublevel. Electronic Configuration of Ions Electronic configuration of Mg2+? Electronic configuration of Cl-? Arrangement of electrons in orbitals of equal energy How are three electrons 3 electrons in the p sublevel distributed among the three p orbitals? Fredrik Hund put forward a rule to answer this question: Hund’s rule of maximum multiplicity states that when two or more orbitals of equal energy are available, the electrons occupy them singly before filling them in pairs. Arrangement of Electrons in individual orbitals The ‘electrons in boxed’ diagram show how electrons are distributed in orbitals. - The box = the orbital - The arrow = the electron Arrows are used because it was discovered that as electrons revolve around the nucleus, they spin on their own axes. This spin can be either clockwise or counter-clockwise. Pauli Exclusion Principal: no more than two electrons may occupy an orbital and they must have opposite spin A group of orbitals that all have the same energy is called an energy sub-level. For example, the 2px, 2py and 2pz orbitals make up the 2p sublevel. An energy sublevel is a group of atomic orbitals within an atom, all of which have the same energy.