DR. JONES - BMS EXAM 3 (13 LECTURE HRS) PDF
Document Details
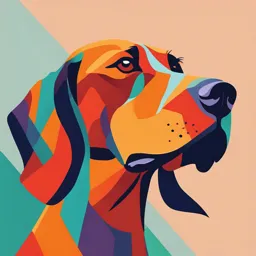
Uploaded by AttentiveEarth
LECOM School of Pharmacy
Dr. Jones
Tags
Summary
This document contains lecture notes for a BMS exam covering topics such as neural tissue and membrane potential. The summary includes examples of questions, such as What is the main function of neural tissue?
Full Transcript
NEURAL TISSUE – DR. JONES (PPT NO. 1) 1. What is the main function of neural tissue in the body? It facilitates communication within the body. 2. What is the functional unit of the nervous system? The neuron. 3. What are the four main parts of a neuron? Dendrites, soma, axon, and terminal butto...
NEURAL TISSUE – DR. JONES (PPT NO. 1) 1. What is the main function of neural tissue in the body? It facilitates communication within the body. 2. What is the functional unit of the nervous system? The neuron. 3. What are the four main parts of a neuron? Dendrites, soma, axon, and terminal buttons. 4. What is the function of dendrites in a neuron? They receive signals from other neurons. 5. What is the soma of a neuron? It is the cell body of the neuron, which contains the nucleus. 6. What is the role of the axon in a neuron? The axon transmits electrical impulses away from the soma to other neurons or muscles. 7. What are terminal buttons in a neuron? They are the structures at the end of the axon that release neurotransmitters to communicate with other neurons. 8. How are neurons classified based on projections from the soma? Neurons are classified as unipolar, bipolar, pseudounipolar, and multipolar. 9. What is a unipolar neuron? A neuron with a single projection from the soma that divides into two branches. 10. What is a bipolar neuron? A neuron with two projections, one axon and one dendrite, extending from the soma. 11. What is a pseudounipolar neuron? A neuron that has one projection from the soma, which splits into two branches, functioning similarly to a unipolar neuron. 12. What is a multipolar neuron? A neuron with multiple projections, including one axon and several dendrites. 13. What are glial cells? Glial cells are the support cells of the nervous system. 14. What is the main function of glial cells? They support neurons and facilitate proper signaling. 15. How do glial cells contribute to neuron function? They provide structural support, protection, and nutrients to neurons. 16. What is the central role of the nervous system? To coordinate and control communication throughout the body. 17. How is the nervous system organized? It is divided into the central nervous system (CNS) and the peripheral nervous system (PNS). 18. What does the central nervous system (CNS) consist of? The brain and spinal cord. 19. What is the role of the peripheral nervous system (PNS)? It connects the central nervous system to the rest of the body. 20. What is the primary function of the CNS? To process and integrate information, and to control most functions of the body and mind. 21. What is the primary function of the PNS? To relay information between the CNS and the limbs and organs. 22. How do neurons communicate with each other? Through electrical and chemical signals. 23. What is the role of neurotransmitters in neural communication? They are chemical messengers that transmit signals across synapses between neurons. 24. What is a synapse? A junction between two neurons where information is transmitted. 25. What happens at the synaptic cleft? Neurotransmitters are released from the terminal buttons to communicate with the next neuron. 26. What part of the neuron is responsible for sending outgoing signals? The axon. 27. What part of the neuron is responsible for receiving incoming signals? The dendrites. 28. What is the significance of neuron classification? It helps to identify neurons based on structure and function. 29. What role do glial cells play in maintaining homeostasis in the nervous system? They regulate the environment around neurons, ensuring proper function. 30. What happens if glial cells fail to support neurons properly? Neuronal signaling can be disrupted, leading to dysfunction in the nervous system. MEMBRANE POTENTIAL – DR. JONES (PPT NO. 2) 1. What is membrane potential? It is the potential difference across the membrane of a cell at rest, with the inside of the cell being negative compared to the outside. 2. Why do charges line up along the plasma membrane? Because opposite charges are attracted to each other. 3. What are the two main determinants of membrane potential? The concentration of electrolytes in the extracellular fluid (ECF) and intracellular fluid (ICF) and the membrane permeability to different ions. 4. What two forces act on ions across a membrane? Chemical and electrical forces. 5. How does membrane permeability to potassium (K+) affect the resting potential? If the membrane is only permeable to K+, the resting potential is determined primarily by K+ movement. 6. What happens when the concentration gradient balances the electrical gradient? Electrochemical equilibrium is reached, and there is no net movement of ions across the membrane. 7. What is the Nernst equation used for? It is used to calculate the electrochemical equilibrium potential for an ion. 8. What is the Nernst equation? Eion = 61 log (Cout / Cin), where Cout is the extracellular concentration, Cin is the intracellular concentration, and z is the ion valence. 9. What is the equilibrium potential for K+ using the Nernst equation? The equilibrium potential for K+ is approximately -90 mV. 10. What is the equilibrium potential for Na+ using the Nernst equation? The equilibrium potential for Na+ is approximately +60 mV. 11. What is the measured resting membrane potential of a typical cell? The resting membrane potential is -70 mV. 12. If the cell is only permeable to K+, what is the equilibrium potential? The equilibrium potential is -90 mV. 13. If the cell is only permeable to Na+, what is the equilibrium potential? The equilibrium potential is +60 mV. 14. What equation is used to calculate membrane potential (Vm) when the membrane is permeable to multiple ions? The Goldman-Hodgkin-Katz equation. 15. What is the general form of the Goldman-Hodgkin-Katz equation? Vm = 61 log ( PK [Kout + PNa [Naout] + PCl [Clin] / PK [Kin + PNa [Nain] + PCl [Clout] ) 16. What is the calculated membrane potential using typical ion concentrations? Approximately -83 mV. 17. Which ion has the most influence on the membrane potential? The ion that the membrane is most permeable to, usually K+. 18. What are leak K+ channels? Non-gated channels that allow K+ to leave the cell, contributing to high K+ permeability. 19. How do Na+ leak channels compare to K+ leak channels? Na+ leak channels are less abundant than K+ leak channels, so the membrane is less permeable to Na+. 20. What is the role of the Na+/K+ ATPase pump? It maintains ion gradients by actively transporting Na+ out of the cell and K+ into the cell. 21. Why is it important to study membrane potential? Because nerve and muscle cells are excitable and can change their membrane potential to communicate. 22. How do nerve and muscle cells communicate? By changing their membrane permeability to ions, often through the opening and closing of ion channels. 23. What happens if the membrane becomes highly permeable to Na+? The membrane potential would become more positive, approaching the equilibrium potential for Na+. 24. What would happen if extracellular K+ concentration increased? The resting membrane potential would become less negative, depolarizing the cell. 25. How does the Na+/K+ pump contribute to maintaining the resting membrane potential? It pumps Na+ out and K+ in, creating the necessary gradients that establish the resting potential. 26. What is the importance of the K+ leak channels in membrane potential? They allow K+ to continuously exit the cell, making the inside of the cell more negative and stabilizing the resting potential. 27. What happens if the membrane is impermeable to a particular ion? That ion will not contribute to the membrane potential. 28. How do changes in ion permeability affect excitable cells? They cause changes in membrane potential, which is essential for initiating action potentials in nerve and muscle cells. 29. What is the main factor that determines the resting membrane potential? The relative permeability of the membrane to K+ and Na+. 30. How does the Goldman-Hodgkin-Katz equation differ from the Nernst equation? The Goldman-Hodgkin-Katz equation accounts for multiple ions and their permeabilities, while the Nernst equation only calculates the equilibrium potential for a single ion. ACTION POTENTIAL – DR. JONES (PPT NO. 3) 1. What does it mean to depolarize the membrane? It means moving the membrane potential in a positive direction from its resting state. 2. What does hyperpolarization refer to? It refers to moving the membrane potential in a negative direction from rest. 3. What is repolarization? It is the process of returning the membrane potential to its resting state. 4. In what type of tissue do large changes in membrane potential occur? Large changes in membrane potential occur in excitable tissue. 5. What is the key factor in the generation of an action potential? Changes in ion permeability. 6. How fast are the changes in ion permeability during an action potential? They are rapid changes followed by quick recovery. 7. What are graded potentials? They are local membrane potential changes that vary with stimulus strength. 8. How do graded potentials behave as they move away from the stimulus? They are decremented, meaning they decrease in strength with distance. 9. Can graded potentials be excitatory or inhibitory? Yes, graded potentials can either depolarize (excitatory) or hyperpolarize (inhibitory) the membrane. 10. What triggers an action potential? A local depolarization of the membrane activates voltage-gated Na+ channels. 11. What happens when the threshold for an action potential is reached? The membrane becomes 600 times more permeable to Na+ than to K+. 12. What occurs when Na+ enters the cell during an action potential? The membrane becomes more positive, resulting in depolarization. 13. How many gates do voltage-gated Na+ channels have? Two gates: the m-gate (activation gate) and the h-gate (inactivation gate). 14. What is the role of the m-gate on a sodium channel? It is the activation gate that opens when the membrane depolarizes. 15. What is the function of the h-gate on a sodium channel? It is the inactivation gate that blocks the channel when the membrane becomes more positive. 16. What ensures rapid depolarization during an action potential? Positive feedback, where depolarization causes more Na+ channels to open. 17. What happens to Na+ channels at the peak of the action potential? Voltage-gated Na+ channels inactivate as the h-gate blocks the pore. 18. What channels open as Na+ channels inactivate during repolarization? Voltage-gated K+ channels open, allowing K+ to leave the cell. 19. How many gates do voltage-gated K+ channels have? They have one gate, the n-gate, which opens slowly during depolarization. 20. What occurs when K+ channels open during repolarization? K+ leaves the cell, causing the membrane to return to a more negative potential. 21. What is afterhyperpolarization? It is a phase where the K+ permeability is greater than at rest due to both voltage-gated and leak K+ channels being open. 22. Why does afterhyperpolarization occur? Because K+ permeability exceeds resting levels as voltage-gated K+ channels remain open. 23. When is the membrane potential restored to its resting state? When all voltage-gated K+ channels close. 24. What term is used to describe the state after an action potential when the membrane becomes more negative than at rest? Afterhyperpolarization. 25. How do voltage-gated K+ channels differ from Na+ channels in activation speed? K+ channels open more slowly than Na+ channels during depolarization. 26. What is the primary ion responsible for repolarization of the membrane? Potassium (K+), which leaves the cell to repolarize the membrane. 27. What happens to the h-gate of Na+ channels after depolarization? The h-gate blocks the Na+ channel, inactivating it. 28. What effect does Na+ have on the membrane potential during an action potential? Na+ influx causes the membrane to become more positive, leading to depolarization. 29. What causes the membrane potential to return to a negative value during repolarization? The exit of K+ from the cell through voltage-gated K+ channels. 30. Why is it important for voltage-gated K+ channels to close after an action potential? Their closure restores the membrane potential to its resting level, ending the afterhyperpolarization phase. REFRACTORY AND PROPAGATION – DR. JONES (PPT NO. 4) 1. What is the absolute refractory period? It is the period during which no stimulus, no matter how strong, can elicit a new action potential. 2. What is the relative refractory period? It is the period during which a stronger-than-normal stimulus can elicit an action potential. 3. Can a stimulus elicit an action potential during the absolute refractory period? No, no stimulus can elicit an action potential during the absolute refractory period. 4. What happens during the relative refractory period with a stronger stimulus? A stronger stimulus may elicit an action potential during the relative refractory period. 5. What is meant by "all or none" in relation to action potentials? Action potentials either occur maximally or not at all; they are not graded based on stimulus strength. 6. How does an increase in stimulus strength affect action potentials? It increases the number of action potentials, not their strength. 7. Where are action potentials initiated in a neuron? They are initiated in the axon hillock. 8. What opens voltage-gated channels in the initial segment of an axon? Local potentials. 9. What are the two methods of voltage propagation along an axon? Contiguous conduction and saltatory conduction. 10. What is continuous conduction? It is the slow conduction of action potentials in unmyelinated axons. 11. How does charge flow during continuous conduction? Charge flows from the initial depolarization site to inactive areas, though some leaks out along the axon. 12. How do local potentials spread in continuous conduction? Local potentials spread to the axon terminal button in an unmyelinated axon. 13. What is saltatory conduction? It is the fast conduction of action potentials in myelinated axons. 14. How does myelination affect conduction velocity? Myelination increases conduction velocity by insulating the axon. 15. What are the cells responsible for myelination in the PNS? Schwann cells. 16. What are the cells responsible for myelination in the CNS? Oligodendrocytes. 17. Where are action potentials located in saltatory conduction? Action potentials occur at the nodes of Ranvier. 18. What role do the nodes of Ranvier play in saltatory conduction? They allow action potentials to "jump" from node to node, speeding up conduction. 19. How does myelin function as an insulator? Myelin acts like insulation on a wire, helping to hold charge inside the axon. 20. How does axon diameter affect conduction velocity? Larger axon diameters allow charge to move more quickly to neighboring regions. 21. How does a smaller axon diameter affect the flow of charge? A smaller diameter provides more resistance to charge flow, slowing conduction. 22. What is multiple sclerosis (MS)? MS is an autoimmune disease where the immune system attacks the myelin in the CNS. 23. How does multiple sclerosis affect conduction velocity? It decreases conduction velocity by damaging the myelin sheath of neurons. 24. What are the two types of refractory periods in neurons? Absolute refractory period and relative refractory period. 25. During which refractory period can a new action potential be generated? During the relative refractory period, with a stronger stimulus. 26. Why can't a new action potential occur during the absolute refractory period? Because the sodium channels are either already open or inactivated, preventing any further depolarization. 27. What is the main cause of afterhyperpolarization? The slow closing of voltage-gated potassium channels after repolarization. 28. What ensures that action potentials only travel in one direction along the axon? The refractory periods prevent the action potential from reversing direction. 29. What would happen if myelin were damaged along an axon? Conduction would slow significantly or stop altogether, as seen in diseases like MS. 30. What type of conduction is faster: continuous or saltatory? Saltatory conduction is faster due to the myelination of axons. SYNAPSE – DR. JONES (PPT NO. 5) 1. What is a synapse? It is the junction between two neurons or between a neuron and a muscle. 2. What are the components of a synapse? Presynaptic neuron, synaptic cleft, and postsynaptic neuron. 3. What are the two types of synapses? Electrical synapses and chemical synapses. 4. How do electrical synapses function? Pre- and postsynaptic cells are connected via gap junctions. 5. How do chemical synapses function? Neurotransmitters are released from presynaptic cells and bind to receptors on the postsynaptic membrane. 6. What role does Ca²⁺ play in presynaptic events at a chemical synapse? Ca²⁺ is required for the regulated exocytosis of neurotransmitters. 7. What are V-snares involved in neurotransmitter release? Synaptotagmin and synatobrevin. 8. What are T-snares involved in neurotransmitter release? Syntaxin and SNAP-25. 9. What happens when neurotransmitters bind to postsynaptic receptors? Ion channels open or close, changing membrane permeability and membrane potential. 10. What are the two types of postsynaptic receptors? Inotropic and metabotropic receptors. 11. What is an inotropic receptor? It is a receptor protein that is also an ion channel. 12. What is a metabotropic receptor? It is a receptor that activates a signaling cascade to open or close ion channels. 13. What are the three mechanisms for removing neurotransmitters from the synapse? Inactivation by enzymes, reuptake, and diffusion away from the synapse. 14. What is an excitatory synapse (EPSP)? A synapse where the postsynaptic membrane is depolarized, bringing it closer to the threshold for an action potential. 15. What is an inhibitory synapse (IPSP)? A synapse where the postsynaptic membrane is hyperpolarized, moving it away from the threshold for an action potential. 16. What is temporal summation? The additive effect of multiple synaptic inputs occurring in close time from the same presynaptic neuron. 17. What is spatial summation? The additive effect of synaptic inputs occurring simultaneously from different presynaptic neurons. 18. How are EPSPs and IPSPs produced? They are produced by neurotransmitters released from axon terminals. 19. What are the main classes of neurotransmitters? Acetylcholine, catecholamines/biogenic amines, and amino acids. 20. What is the major neurotransmitter of the autonomic and somatic nervous system? Acetylcholine. 21. How is acetylcholine synthesized? It is synthesized from choline and acetyl coenzyme A in axon terminals. 22. How is acetylcholine metabolized? It is metabolized by the enzyme acetylcholinesterase, and choline is recycled. 23. What are biogenic amines? Small molecules synthesized from amino acids, including catecholamines like dopamine, epinephrine, and norepinephrine. 24. What enzymes metabolize catecholamines? Monoamine oxidase (MAO) and catechol-O-methyltransferase (COMT). 25. What is serotonin also known as? 5-hydroxytryptamine (5-HT). 26. How is serotonin synthesized? It is synthesized from the amino acid tryptophan. 27. What is the role of serotonin in the brain? It affects mood and emotions. 28. How is serotonin removed from the synaptic cleft? Through reuptake and metabolism by MAO. 29. What are the two types of excitatory amino acid neurotransmitters? Glutamate and its receptors, AMPA and NMDA. 30. What are the two types of inhibitory amino acids? GABA (which increases Cl⁻ or K⁺ permeability) and glycine (which increases Cl⁻ permeability). NEUROMUSCULAT JUNCTION, NMJ – DR. JONES (PPT NO. 6) 1. What is involved in somatic neural signaling at the neuromuscular junction? Muscle fiber, α-motor neuron, and motor endplate. 2. What are the key structures of the neuromuscular junction? The neuromuscular junction consists of the presynaptic nerve terminal, synaptic cleft, and postsynaptic motor endplate. 3. What type of receptor is found at the neuromuscular junction? Nicotinic acetylcholine receptors. 4. What is the sequence of events after acetylcholine binds to nicotinic receptors? Local depolarization, threshold potential is reached, and an action potential is triggered. 5. What is an end plate potential (EPP)? A local (graded) depolarization at the neuromuscular junction. 6. How does an EPP lead to an action potential in the muscle? EPPs sum to reach the threshold potential, triggering an action potential. 7. How does the muscle action potential compare to the neuronal action potential? The muscle action potential is similar but has a slightly longer duration than a neuronal action potential. 8. Where does the muscle action potential propagate after it is generated? It propagates into the t-tubules to signal Ca²⁺ release. 9. What is excitation-contraction coupling? The process where the action potential propagates into the t-tubule, activating DHP (voltage sensor) and ryanodine receptor to release Ca²⁺ from the sarcoplasmic reticulum. 10. What are the two key proteins involved in Ca²⁺ release during excitation-contraction coupling? DHP receptor (voltage sensor) and ryanodine receptor (Ca²⁺ release channel). 11. What is Myasthenia Gravis? An autoimmune disorder where antibodies destroy some nicotinic acetylcholine receptors on the motor endplate. 12. What are the forms of Myasthenia Gravis? Extraocular (affecting eye muscles) and generalized skeletal muscle weakness. 13. How is Myasthenia Gravis treated? With acetylcholinesterase inhibitors (e.g., neostigmine), immunosuppressive drugs, and thymectomy. 14. What is Lambert-Eaton Syndrome? An autoimmune disorder where antibodies destroy voltage-gated Ca²⁺ channels on nerve endings at the neuromuscular junction. 15. What muscles are primarily affected by Lambert-Eaton Syndrome? Proximal muscles in the lower extremities. 16. What are the clinical symptoms of Lambert-Eaton Syndrome? Gait issues, difficulty raising arms, and weakness, which improve with repetitive activity. 17. What is the association between Lambert-Eaton Syndrome and cancer? It is often associated with small cell lung cancer. 18. How is Lambert-Eaton Syndrome treated? Repetitive stimulation, immunosuppressive drugs, and aminopyridines, which improve acetylcholine release by blocking K⁺ channels. 19. What is the role of aminopyridines in treating Lambert-Eaton Syndrome? They block K⁺ channels, prolong Ca²⁺ channel activation, and enhance acetylcholine release. 20. How does smooth muscle neuromuscular signaling differ from skeletal muscle? Smooth muscle has no clearly defined neuromuscular junction; instead, it has synapses en passant with varicosities. 21. What kind of nerve endings typically synapse with smooth muscle? Mainly autonomic nerve endings. 22. How is excitation-contraction coupling mediated in smooth muscle? Through extracellular Ca²⁺ entry, which triggers release from the sarcoplasmic reticulum and contraction. 23. What are the types of action potentials seen in smooth muscle? Spike potentials, plateau potentials, and slow waves. 24. What mediates the upstroke of smooth muscle spike potentials? Ca²⁺-mediated upstroke. 25. What mediates the repolarization of smooth muscle action potentials? K⁺-mediated repolarization. 26. What causes plateau potentials in smooth muscle? Sustained Ca²⁺ entry into the cell. 27. What are slow waves in smooth muscle action potentials? Rhythmic fluctuations that can lead to spike potentials. 28. What role does the t-tubule play in skeletal muscle contraction? It transmits the action potential deep into the muscle fiber to initiate Ca²⁺ release. 29. How does acetylcholine affect the motor endplate at the neuromuscular junction? It binds to nicotinic receptors, causing Na⁺ influx and local depolarization. 30. What is the primary ion responsible for depolarization at the neuromuscular junction? Sodium (Na⁺). MUSCLE ANATOMY – DR. JONES (PPT NO. 7) 1. What is muscle contraction at the cellular level? Muscle contraction is a cellular event that leads to whole muscle force generation, which is the summation of the force generated by individual muscle cells. 2. What are the three types of muscle control? Voluntary (skeletal), involuntary (cardiac), and visceral (smooth). 3. Which muscles are classified as striated muscles? Skeletal and cardiac muscles. 4. Which type of muscle is responsible for large forceful movements? Skeletal muscle. 5. What type of muscle is responsible for pumping blood through the circulation? Cardiac muscle. 6. Which muscle type controls the movement of fluids through visceral organs and vasculature? Smooth (visceral) muscle. 7. How does skeletal muscle fatigue? Skeletal muscle experiences both rapid and slow fatigue. 8. What is the primary source of heat in the body from muscle tissue? Skeletal muscle. 9. What is the primary function of smooth muscle in the body? To control the movement of fluid through visceral organs and blood vessels, with long and short contraction times. 10. What are skeletal muscles composed of? Skeletal muscles are composed of fibers (muscle cells) that extend the entire length of the muscle. 11. What is the name of the muscle cell membrane? Sarcolemma. 12. What is the name of the cytoplasm inside a muscle cell? Sarcoplasm. 13. What unique characteristic do skeletal muscle cells have regarding nuclei? Skeletal muscle cells are multinucleated. 14. What is the contractile unit of a skeletal muscle cell? The sarcomere. 15. What proteins are involved in the structure of a sarcomere? Actin (thin filament) and myosin (thick filament). 16. What is the role of titan in the sarcomere? Titan anchors myosin to the α-actin Z disc (line). 17. What happens to the I-band during muscle contraction? The I-band decreases in size during contraction. 18. What is the structure of the actin filament in skeletal muscle? Actin is a double helical filament made of g-actin monomers. 19. Where are the myosin-binding sites located on the actin filament? Myosin-binding sites are located on the g-actin monomers of the actin filament. 20. What protein lies between the actin grooves and covers the myosin-binding sites? Tropomyosin. 21. What are the three subunits of the troponin complex? Tn-I, Tn-C, and Tn-T. 22. What is the role of troponin in muscle contraction? Troponin is attached to tropomyosin and regulates the exposure of the myosin-binding sites on actin during muscle contraction. 23. What is the structure of the myosin filament in skeletal muscle? Myosin has a tail composed of light and heavy meromyosin (S2) and a head (S1) region with actin-binding sites. 24. What is the structure of the tail of the myosin filament? The tail of myosin is an α-helical dimer composed of light and heavy meromyosin. 25. What region of the myosin filament contains ATP enzymatic activity? The head (S1) region of myosin contains ATP enzymatic activity. 26. What is the primary function of the head (S1) of the myosin filament? The head (S1) of myosin binds to actin and facilitates muscle contraction through ATP hydrolysis. 27. How does the sarcomere contribute to the force generation in muscles? The sarcomere shortens during muscle contraction, generating force through the sliding filament mechanism. 28. What organelles surround the sarcomere to supply energy and calcium? Mitochondria and the sarcoplasmic reticulum surround the sarcomere. 29. What is the function of the sarcoplasmic reticulum in muscle contraction? The sarcoplasmic reticulum stores and releases calcium, which is essential for muscle contraction. 30. What happens to the length of the sarcomere during muscle contraction? The sarcomere shortens as actin and myosin filaments slide past each other. SKELETAL MUSCLE – DR. JONES (PPT NO. 8) 1. What triggers muscle contraction in skeletal muscle? Action potential leads to an increase in Ca2+ levels in the sarcoplasm. 2. What ion regulates muscle contraction in skeletal muscle? Calcium (Ca2+). 3. What is the process called that leads to the shortening of the sarcomere during muscle contraction? Cross-bridge cycling. 4. What energy source is used during cross-bridge cycling? ATP (adenosine triphosphate). 5. What happens during the first step of cross-bridge cycling? ATP is hydrolyzed by the myosin head, providing energy for the cycle. 6. What occurs after the hydrolysis of ATP in cross-bridge cycling? The myosin head binds to actin, with ADP and Pi (inorganic phosphate) still attached. 7. What causes the myosin head to perform the "power stroke" or "ratchet" motion? The release of ADP and Pi from the myosin head. 8. What causes the myosin head to detach from actin during cross-bridge cycling? A new ATP molecule binds to the myosin head. 9. What happens once the myosin head detaches from actin? The cross-bridge cycle can start over again. 10. What happens to Ca2+ levels during muscle relaxation? Ca2+ levels drop in the sarcoplasm. 11. Why do Ca2+ levels drop during muscle relaxation? The action potential stops, acetylcholine (Ach) is removed, and Ca2+ is pumped back into the sarcoplasmic reticulum by Ca2+ ATPase. 12. What is the role of Ca2+ in muscle contraction? Ca2+ binds to troponin, causing a conformational change that moves tropomyosin away from the myosin-binding sites on actin. 13. What is required to initiate the detachment of the myosin head from actin? A new ATP molecule must bind to the myosin head. 14. What enzyme removes acetylcholine (Ach) during muscle relaxation? Acetylcholinesterase. 15. What causes the myosin head to return to its resting position after detachment from actin? Hydrolysis of the newly bound ATP molecule resets the myosin head. 16. What is the role of Ca2+ ATPase during muscle relaxation? It pumps Ca2+ back into the sarcoplasmic reticulum, lowering cytosolic Ca2+ levels. 17. What happens to the actin-myosin binding site when Ca2+ is removed during relaxation? Tropomyosin moves back to cover the myosin-binding sites on actin, preventing further cross-bridge formation. 18. How is the cross-bridge cycle repeated during muscle contraction? As long as Ca2+ is present and ATP is available, the cross-bridge cycle continues to repeat. 19. What happens to the sarcomere during cross-bridge cycling? The sarcomere shortens, causing muscle contraction. 20. What provides the energy for the myosin head to perform the power stroke? The energy comes from ATP hydrolysis, which occurs before the myosin head binds to actin. 21. What are the two by-products released after ATP is hydrolyzed by myosin? ADP (adenosine diphosphate) and Pi (inorganic phosphate). 22. What marks the end of a muscle contraction cycle? The removal of Ca2+ and the detachment of the myosin head from actin. 23. How does a muscle return to its resting state after contraction? Ca2+ is removed, acetylcholine is degraded, and the sarcomere returns to its resting length. 24. What molecular event directly leads to the ratchet movement of the myosin head? The release of ADP and Pi from the myosin head. 25. During relaxation, where is Ca2+ actively transported to? Back into the sarcoplasmic reticulum. 26. What happens to the muscle fiber when ATP is depleted? The muscle fiber cannot relax because myosin cannot detach from actin, potentially leading to a condition called rigor mortis. 27. What is the significance of ATP in both muscle contraction and relaxation? ATP is required for myosin to bind to actin (contraction) and for the myosin head to detach from actin (relaxation). 28. What structural proteins regulate the interaction between actin and myosin? Tropomyosin and troponin regulate the binding of myosin to actin by controlling access to the myosin-binding sites on actin. 29. How does the absence of an action potential affect muscle contraction? Without an action potential, Ca2+ is not released from the sarcoplasmic reticulum, preventing muscle contraction. 30. What causes the drop in Ca2+ levels in the sarcoplasm during muscle relaxation? The cessation of the action potential and the active pumping of Ca2+ back into the sarcoplasmic reticulum by Ca2+ ATPase. SMOOTH MUSCLE – DR. JONES (PPT NO. 9) 1. What protein complex is absent in smooth muscle that is present in skeletal muscle? The troponin complex. 2. How is the contraction mechanism in smooth muscle similar to skeletal muscle? Both involve cross-bridge cycling. 3. To what are actin filaments attached in smooth muscle cells? Actin filaments are attached to dense bodies. 4. What regulates contraction in smooth muscle? Contraction is regulated by Ca2+ via myosin light chain (MLC) activation. 5. What happens when MLC is phosphorylated in smooth muscle? Phosphorylation of MLC allows it to interact with actin, leading to contraction. 6. What role do phosphatases play in smooth muscle contraction? Phosphatases decrease contraction by dephosphorylating MLC. 7. What is the term for the cross-bridge cycling process in smooth muscle that is similar to skeletal muscle? Latch-state. 8. What are the three types of coupling that regulate smooth muscle? Electrochemical, pharmochemical, and mechanochemical coupling. 9. What ion's release is crucial for smooth muscle contraction? Ca2+ (calcium). 10. How does smooth muscle relaxation occur? By decreasing intracellular Ca2+ levels. 11. What enzyme is activated during smooth muscle relaxation to reduce contraction? Myosin phosphatases. 12. What intracellular messenger increases to promote smooth muscle relaxation? cGMP (cyclic guanosine monophosphate). 13. How does cAMP affect smooth muscle contraction? It inhibits myosin light chain kinase (MLCK), leading to relaxation. 14. What ion channel is involved in smooth muscle hyperpolarization during relaxation? Ca2+-activated K+ channels. 15. What is the function of myosin light chain kinase (MLCK) in smooth muscle contraction? MLCK phosphorylates the myosin light chain, enabling interaction with actin. 16. What does the "latch-state" in smooth muscle refer to? It refers to a state where myosin remains attached to actin for a prolonged period, maintaining contraction with low energy use. 17. What increases cGMP levels in smooth muscle cells? The activation of myosin phosphatases. 18. How does cAMP contribute to smooth muscle relaxation? By inhibiting MLCK, it prevents phosphorylation of MLC, reducing contraction. 19. What triggers smooth muscle hyperpolarization? The activation of Ca2+-activated K+ channels. 20. What role does Ca2+ play in smooth muscle contraction? Ca2+ binds to calmodulin, activating MLCK, which phosphorylates MLC, leading to contraction. 21. What is the function of phosphatases in smooth muscle relaxation? They dephosphorylate MLC, decreasing contraction. 22. How do electrochemical signals affect smooth muscle contraction? They regulate Ca2+ influx, affecting the contraction process. 23. What is the primary difference between smooth and skeletal muscle contraction regulation? Smooth muscle contraction is regulated by myosin phosphorylation, whereas skeletal muscle is regulated by troponin-tropomyosin complex. 24. What happens when Ca2+ is removed from the cytoplasm in smooth muscle? The muscle relaxes as myosin is dephosphorylated. 25. What are the primary molecules that inhibit smooth muscle contraction? cAMP and cGMP, which decrease phosphorylation of MLC. 26. What is the role of MLCK in smooth muscle contraction? MLCK phosphorylates myosin light chains, enabling actin-myosin interaction. 27. What happens when intracellular Ca2+ levels drop in smooth muscle? Contraction ceases, and relaxation begins. 28. What is the effect of cAMP on MLCK? cAMP inhibits MLCK, leading to smooth muscle relaxation. 29. What ion helps activate myosin phosphatase during smooth muscle relaxation? Ca2+, indirectly through signaling pathways that increase cGMP. 30. What type of channel promotes smooth muscle relaxation through hyperpolarization? Ca2+-activated K+ channels. CARDIAC MUSCLE – DR. JONES (PPT NO. 10) 1. What are the tissue layers of the heart? The heart's tissue layers include the epicardium, myocardium, and endocardium. 2. What are the three general anatomical layers of the heart? The heart consists of the epicardium (outer layer), myocardium (middle muscle layer), and endocardium (inner layer). 3. What type of muscle is cardiac muscle classified as? Cardiac muscle is classified as striated muscle. 4. What structures in cardiac muscle are responsible for cell-to-cell cohesion? Intercalated discs are responsible for cell-to-cell cohesion in cardiac muscle. 5. What role do gap junctions play in cardiac muscle? Gap junctions allow electrical signals to pass between cardiac muscle cells, enabling coordinated contraction. 6. Why are there many mitochondria in cardiac muscle? Cardiac muscle has many mitochondria to meet the high energy demands of continuous contraction. 7. What is the molecular mechanism of contraction in cardiac muscle? The molecular mechanism of contraction in cardiac muscle involves actin-myosin cross-bridge cycling, similar to skeletal muscle. 8. How do action potentials in cardiac muscle lead to contraction? Action potentials in cardiac muscle cause contraction by triggering Ca2+ influx during the plateau phase. 9. What is the significance of the plateau phase in cardiac muscle action potentials? The plateau phase prolongs depolarization, allowing more Ca2+ to enter the cell, which is crucial for sustained contraction. 10. How does the plateau phase of cardiac muscle action potentials differ from skeletal muscle action potentials? The plateau phase in cardiac muscle is longer, allowing increased Ca2+ entry, whereas skeletal muscle action potentials are shorter. 11. What structure in cardiac muscle allows the spread of electrical signals between cells? Gap junctions. 12. What are intercalated discs made up of? Intercalated discs are composed of desmosomes and gap junctions. 13. What is the primary energy source for cardiac muscle cells? ATP produced by the numerous mitochondria in cardiac muscle cells. 14. What ion is crucial for cardiac muscle contraction? Ca2+ (calcium). 15. What happens during the contraction phase of cardiac muscle? The cardiac muscle contracts through actin-myosin cross-bridge cycling initiated by Ca2+ influx. 16. How is cardiac muscle contraction similar to skeletal muscle contraction? Both involve cross-bridge cycling between actin and myosin. 17. How do intercalated discs contribute to cardiac muscle function? They enhance mechanical and electrical connectivity between cardiac muscle cells. 18. What is the main difference between cardiac muscle and skeletal muscle in terms of action potential? Cardiac muscle action potentials have a plateau phase, while skeletal muscle action potentials do not. 19. Why is the plateau phase essential for the functioning of the heart? It ensures that the heart has a sustained contraction, which is necessary for effective pumping of blood. 20. What is the role of mitochondria in cardiac muscle? They provide the energy (ATP) required for continuous and sustained contraction. 21. What are the primary components of the intercalated discs? Desmosomes and gap junctions. 22. What role does Ca2+ play in cardiac muscle contraction? Ca2+ binds to troponin, allowing myosin to bind to actin and initiate contraction. 23. How does cardiac muscle differ from skeletal muscle in terms of fatigue? Cardiac muscle is highly resistant to fatigue due to its abundant mitochondria and continuous blood supply. 24. What causes the initiation of contraction in cardiac muscle? The influx of Ca2+ during the plateau phase of the action potential. 25. What is the function of desmosomes in intercalated discs? Desmosomes provide mechanical strength to prevent cardiac muscle cells from separating during contraction. 26. What feature of cardiac muscle cells allows them to contract in a coordinated manner? Gap junctions allow for synchronized contraction by facilitating the flow of electrical signals between cells. 27. What is the significance of the long refractory period in cardiac muscle? It prevents tetanus (continuous contraction), ensuring the heart relaxes between beats. 28. How does the cardiac action potential affect intracellular calcium levels? The plateau phase of the action potential allows more Ca2+ to enter the cell, which is necessary for contraction. 29. What structural feature ensures that cardiac muscle cells do not tear apart during contraction? Desmosomes in the intercalated discs. 30. What ensures the continuous energy supply required for cardiac muscle contraction? The large number of mitochondria in cardiac muscle cells ensures a continuous supply of ATP. AUTONOMICS ANATOMY – DR. JONES (PPT NO. 11) 1. What are the two main divisions of the autonomic nervous system? The sympathetic division and the parasympathetic division. 2. What is the primary role of the autonomic nervous system? To maintain homeostasis in the body. 3. How many neural connections are there between the spinal cord and target tissues in the autonomic nervous system? There are two neural connections. 4. What are the types of neurons in the autonomic nervous system? Preganglionic neurons and postganglionic neurons. 5. Are preganglionic neurons myelinated or unmyelinated? Preganglionic neurons are myelinated. 6. What neurotransmitter is released by preganglionic neurons? Acetylcholine (Ach). 7. Are postganglionic neurons myelinated or unmyelinated? Postganglionic neurons are unmyelinated. 8. What neurotransmitters can postganglionic neurons release? Acetylcholine (Ach) and norepinephrine (NE). 9. Where do sympathetic preganglionic neurons originate? They originate in spinal cord segments T1 to L2. 10. Where do short preganglionic neurons synapse in the sympathetic division? In the sympathetic chain ganglion (paravertebral ganglion). 11. What is the role of the adrenal gland in the sympathetic division? Preganglionic neurons synapse onto chromaffin cells, which secrete epinephrine (80%) and norepinephrine (20%). 12. Where do parasympathetic preganglionic neurons originate? They originate in the brainstem (cranial nerves) and S2-S4. 13. Where do long preganglionic neurons synapse in the parasympathetic division? In ganglia that are in or near the target tissue. 14. What is an autonomic reflex composed of? A receptor, afferent neuron, integration center, efferent neuron(s), and an effector. 15. What type of fibers do parasympathetic preganglionic neurons have? Cholinergic fibers that release acetylcholine (Ach). 16. What receptors are found on the cell bodies of postganglionic neurons in the parasympathetic division? Nicotinic acetylcholine receptors (nAchR). 17. What type of fibers do postganglionic neurons in the parasympathetic division have? Cholinergic fibers that release acetylcholine (Ach). 18. What receptors are found on the target tissues in the parasympathetic division? Muscarinic acetylcholine receptors. 19. What type of fibers do sympathetic preganglionic neurons have? Cholinergic fibers that release acetylcholine (Ach). 20. What receptors are found on the cell bodies of postganglionic neurons in the sympathetic division? Nicotinic acetylcholine receptors (nAchR). 21. What types of fibers can sympathetic postganglionic neurons have? Adrenergic fibers that release norepinephrine (NE) or epinephrine (E) and some cholinergic fibers that release acetylcholine (Ach). 22. What receptors are found on the target tissues in the sympathetic division? Alpha and beta adrenergic receptors, and muscarinic receptors in sweat glands. 23. What type of receptor signaling do adrenergic receptors use? G-protein coupled receptor signaling. 24. What is the function of Gαq in adrenergic receptor signaling? It typically activates phospholipase C, leading to increased intracellular calcium. 25. What is the function of Gαs in adrenergic receptor signaling? It typically stimulates adenylate cyclase, increasing cAMP levels. 26. What is the primary neurotransmitter for sympathetic postganglionic fibers? Norepinephrine (NE). 27. What is the primary neurotransmitter for parasympathetic postganglionic fibers? Acetylcholine (Ach). 28. What is the effect of acetylcholine binding to muscarinic receptors? It mediates various physiological responses such as decreased heart rate and increased glandular secretion. 29. What is the effect of norepinephrine binding to alpha adrenergic receptors? It generally causes vasoconstriction and increases blood pressure. 30. What is the effect of norepinephrine binding to beta adrenergic receptors? It generally causes vasodilation in some vascular beds and increases heart rate and force of contraction. AUTONOMICS FUNCTION – DR. JONES (PPT NO. 12) 1. What are the overall functions of the autonomic nervous system? To regulate involuntary bodily functions and maintain homeostasis. 2. What are single innervation organs? Organs that receive input from only one division of the autonomic nervous system. 3. Give an example of a single innervation organ. Vasculature. 4. How does the sympathetic division affect vasculature? It maintains "vasomotor tone" by adjusting the firing frequency of sympathetic fibers. 5. What is dual innervation? When an organ receives input from both sympathetic and parasympathetic divisions, leading to antagonistic effects. 6. How does the sympathetic division affect cardiac output? It increases cardiac output. 7. How does the parasympathetic division affect cardiac output? It decreases cardiac output. 8. What is the effect of the sympathetic division on pupillary response? It dilates the pupils. 9. What is the effect of the parasympathetic division on pupillary response? It constricts the pupils. 10. What is Horner’s Syndrome? A condition caused by a lesion in the sympathetic supply to the head, eye, and neck. 11. What is a symptom of Horner’s Syndrome? Ptosis (drooping eyelid) and miosis (constricted pupil). 12. How is Horner’s Syndrome diagnosed? Cocaine testing; cocaine normally causes pupils to dilate. 13. What is the difference in pupil response between a normal eye and an eye affected by Horner's Syndrome? In Horner’s Syndrome, the affected pupil does not dilate in response to cocaine. 14. How does the parasympathetic division affect bladder function? It promotes voiding. 15. How does the sympathetic division affect bladder function? It promotes urine retention. 16. What is an example of a cooperative response in dual innervation? Salivation. 17. How does the parasympathetic division affect salivation? It dilates the salivary glands. 18. How does the sympathetic division affect salivation? It constricts the salivary glands. 19. What types of drugs interact with the autonomic nervous system? Drugs that can stimulate or inhibit the actions of the sympathetic or parasympathetic divisions. 20. Which drugs would you use to treat high blood pressure? Antihypertensives that affect the autonomic nervous system, such as beta-blockers. 21. What role does the cerebral cortex play in the autonomic nervous system? Specific thoughts can activate the hypothalamus. 22. What is the major control center of the visceral motor system? The hypothalamus. 23. How does the hypothalamus respond to stimuli? It directs the autonomic nervous system based on internal and external stimuli. 24. What is the function of the midbrain in relation to the autonomic nervous system? It contains reticular formation control centers that influence autonomic functions. 25. What autonomic functions are controlled by the spinal cord? Urination, defecation, and sexual response. 26. How does the autonomic nervous system maintain homeostasis? By regulating involuntary functions such as heart rate, blood pressure, and digestion. 27. What are the main neurotransmitters used in the autonomic nervous system? Acetylcholine (Ach), norepinephrine (NE), and epinephrine (E). 28. How do autonomic reflexes operate? Through a pathway involving receptors, afferent neurons, integration centers, efferent neurons, and effectors. 29. What is the effect of sympathetic stimulation on blood vessels? It typically causes vasoconstriction, increasing blood pressure. 30. What is the effect of parasympathetic stimulation on blood vessels? It generally causes vasodilation, leading to decreased blood pressure. VASCULAR ANATOMY – DR. JONES (PPT NO. 13) 1. What is the general function of arteries? To carry oxygenated blood away from the heart to the tissues of the body. 2. What are the two main anatomical types of arteries? Elastic arteries and muscular arteries. 3. What is the primary function of elastic arteries? To accommodate the surge of blood from the heart during systole and maintain blood pressure during diastole. 4. What is the primary function of muscular arteries? To distribute blood to various parts of the body and regulate blood flow through vasoconstriction and vasodilation. 5. What are arterioles? Small arteries that lead to capillaries and play a key role in regulating blood flow and pressure. 6. What are metarterioles? Specialized arterioles that connect arterioles to capillary beds and control blood flow into those beds. 7. What is the role of precapillary sphincters? To regulate blood flow into capillary beds by constricting or dilating based on the body’s needs. 8. What is a thoroughfare channel? A direct pathway through which blood can flow from arterioles to venules, bypassing capillaries. 9. What is the structure of capillaries? They are single cell thick, allowing for efficient exchange of substances between blood and tissues. 10. What are the three types of capillaries? Continuous, fenestrated, and sinusoidal. 11. Which type of capillary is found in bone marrow? Sinusoidal capillaries. 12. What is a key characteristic of venules? They are more porous than capillaries and can receive fluid from the surrounding tissues. 13. How does the wall structure of venules compare to that of capillaries? Venules have little or no tunica media and are thin-walled. 14. What is a major characteristic of medium veins? They have thin walls and contain valves to prevent backflow of blood. 15. What role do valves in medium veins play? They help maintain blood flow toward the heart, especially in low-pressure conditions. 16. What is the function of the skeletal muscle pump? It helps increase venous return to the heart by compressing veins during muscle contraction. 17. What are large veins known for? They contain the largest proportion of blood in the body. 18. What is a characteristic of the tunica media in large veins? It has very little smooth muscle compared to arteries. 19. What are examples of large veins? Pulmonary veins, vena cava, jugular veins, and renal veins. 20. What are pressure patterns in the vascular system? They refer to the variations in blood pressure throughout the circulatory system. 21. What does total surface area refer to in the vascular context? The combined area of all blood vessels available for exchange of substances. 22. How does blood velocity change in different vessels? Blood velocity is highest in arteries, slows in capillaries for exchange, and increases again in veins. 23. What is relative resistance in blood vessels? It refers to the opposition to blood flow within the circulatory system, influenced by vessel diameter and length. 24. What are circulatory shunts? Direct connections that allow blood to bypass certain capillary beds, often found in fetal circulation. 25. How does the structure of capillaries facilitate exchange? Their thin walls and large surface area maximize diffusion of gases, nutrients, and wastes. 26. What distinguishes continuous capillaries? They have uninterrupted endothelial cells, allowing for selective exchange of small molecules. 27. What distinguishes fenestrated capillaries? They have pores (fenestrations) that increase permeability to larger molecules. 28. What distinguishes sinusoidal capillaries? They have larger openings that allow for the passage of blood cells and larger proteins. 29. Why is the tunica media important in arteries? It provides the necessary strength and elasticity to handle high pressure during heart contractions. 30. What role does the autonomic nervous system play in vascular anatomy? It regulates vascular tone and blood flow through sympathetic and parasympathetic stimulation. ARTERIAL CIRCULATION – DR. JONES (PPT NO. 14) 1. What is the formula for Mean Arterial Pressure (MAP)? MAP = Cardiac Output x Total Peripheral Resistance (TPR). 2. What does TPR represent in the context of arterial circulation? TPR is the sum of resistances to blood flow through all organs, primarily due to arterioles. 3. How is blood flow to an organ determined? Blood flow to an organ is determined by its arteriolar resistance, as MAP is constant throughout the body. 4. What happens to arteriolar resistance in a working muscle? A working muscle is expected to decrease arteriolar resistance to increase blood flow. 5. What is the goal of local vasomotor control? The goal of local vasomotor control is to match metabolism to blood flow. 6. What is meant by "vasomotor control"? Vasomotor control refers to the regulation of the diameter of blood vessels to distribute and control blood flow to organs. 7. What are the two types of control for arterial circulation regulation? Local control and central control. 8. What is involved in central control of arterial circulation? Central control involves neural and hormonal/chemical mechanisms. 9. How does local regulation affect blood flow? Local regulation affects blood flow by adjusting arteriolar resistance in response to the metabolic needs of tissues. 10. What role do arterioles play in arterial circulation? Arterioles regulate blood flow and resistance to maintain MAP and distribute blood to organs. 11. What is the significance of maintaining a constant MAP throughout the body? Maintaining a constant MAP ensures that all organs receive adequate blood flow regardless of changes in activity or position. 12. How do sympathetic nerves influence arteriolar resistance? Sympathetic nerves can cause vasoconstriction, increasing arteriolar resistance and decreasing blood flow to specific areas. 13. What effect does increased metabolism have on arteriolar resistance? Increased metabolism typically leads to decreased arteriolar resistance to increase blood flow to active tissues. 14. What are vasodilators? Vasodilators are substances that cause blood vessels to widen, decreasing resistance and increasing blood flow. 15. What are vasoconstrictors? Vasoconstrictors are substances that cause blood vessels to narrow, increasing resistance and decreasing blood flow. 16. What is the function of endothelial cells in arterioles? Endothelial cells regulate vascular tone and respond to local chemical signals to adjust arteriolar resistance. 17. How does nitric oxide affect arteriolar resistance? Nitric oxide is a vasodilator that decreases arteriolar resistance, enhancing blood flow. 18. What is the role of hormones in regulating arterial circulation? Hormones can influence blood vessel tone and arteriolar resistance, thereby affecting blood flow and pressure. 19. Give an example of a hormone that causes vasoconstriction. Norepinephrine is an example of a hormone that causes vasoconstriction. 20. How does exercise affect arterial circulation in muscles? During exercise, blood flow to muscles increases due to vasodilation and decreased arteriolar resistance. 21. What is autoregulation in the context of blood flow? Autoregulation refers to the ability of organs to maintain constant blood flow despite changes in perfusion pressure. 22. What factors can influence local vasomotor control? Local factors such as metabolic activity, oxygen levels, carbon dioxide levels, and pH can influence vasomotor control. 23. How do local metabolic byproducts affect arterioles? Local metabolic byproducts (e.g., carbon dioxide, lactic acid) typically promote vasodilation to increase blood flow. 24. What is the relationship between cardiac output and blood pressure? Cardiac output directly affects blood pressure; an increase in cardiac output can raise blood pressure if resistance remains constant. 25. Why is the control of blood flow important during physical activity? It ensures that active tissues receive sufficient oxygen and nutrients while waste products are effectively removed. 26. What is the effect of temperature on arteriolar resistance? Increased temperature generally leads to vasodilation, while decreased temperature can cause vasoconstriction. 27. How does the body respond to a decrease in blood pressure? The body may increase sympathetic stimulation and release vasoconstrictors to raise blood pressure and maintain MAP. 28. What is the importance of feedback mechanisms in regulating blood flow? Feedback mechanisms help adjust blood flow in real-time, ensuring tissues receive adequate perfusion according to their needs. 29. What role does the baroreceptor reflex play in arterial circulation? The baroreceptor reflex helps maintain blood pressure by adjusting heart rate and vascular resistance in response to changes in blood pressure. 30. How does arteriolar resistance contribute to blood pressure regulation? Changes in arteriolar resistance can significantly affect blood pressure; increased resistance raises blood pressure, while decreased resistance lowers it. CAPILLARY EXCHANGE – DR. JONES (PPT NO. 15) 1. What is the primary function of capillaries? The primary function of capillaries is the exchange of materials between tissues and blood. 2. What are the two main processes of material exchange in capillaries? The two main processes are diffusion and transcytosis. 3. What is filtration in the context of capillary exchange? Filtration refers to the bulk movement of fluid out of the capillaries into the interstitial space. 4. What factors influence filtration and reabsorption in capillaries? Filtration and reabsorption are influenced by Starling forces. 5. What is the net filtration pressure at the arteriole end of a capillary? The net filtration pressure at the arteriole end is generally positive, favoring filtration. 6. What is the net filtration pressure at the venous end of a capillary? The net filtration pressure at the venous end is generally negative, favoring reabsorption. 7. What effect does vasoconstriction have on capillary pressure (Pc)? Vasoconstriction decreases capillary pressure (Pc) at the organ level. 8. What effect does vasodilation have on capillary pressure (Pc)? Vasodilation increases capillary pressure (Pc) downstream of the arteriole. 9. Name two metabolites that can regulate capillary filtration. Metabolites such as carbon dioxide and lactic acid can regulate capillary filtration. 10. How do immune responses affect capillary filtration? Immune responses can lead to increased capillary permeability, altering filtration. 11. What is edema? Edema is the excess fluid accumulation in the interstitial space. 12. What are some consequences of edema? Consequences of edema include swelling, congestion of fluid, decreased nutrient diffusion, and low blood volume. 13. What can cause increased capillary filtration leading to edema? Causes include hypertension due to increased blood volume, right heart failure, and left heart failure. 14. What effect does liver disease have on capillary reabsorption? Liver disease can decrease reabsorption due to reduced plasma protein levels (πc). 15. How does protein deficiency affect capillary reabsorption? Protein deficiency decreases the oncotic pressure (πc), leading to reduced reabsorption and potential edema. 16. What is a potential cause of obstruction in the lymphatic system? Conditions like elephantiasis can cause obstruction in the lymphatic system. 17. How does right heart failure contribute to edema? Right heart failure leads to increased systemic venous pressure, resulting in increased capillary filtration. 18. How does left heart failure contribute to pulmonary edema? Left heart failure increases pulmonary venous pressure, leading to increased capillary filtration in the lungs. 19. What role do Starling forces play in capillary exchange? Starling forces determine the direction and magnitude of fluid movement across capillary walls. 20. What happens to nutrient diffusion in cases of severe edema? Severe edema can decrease nutrient diffusion due to the increased distance from capillaries to tissues. 21. Why is it important to regulate capillary filtration? Regulating capillary filtration is crucial to maintain fluid balance and prevent edema. 22. What are the two main types of capillary exchange processes? The two main types are passive processes (like diffusion) and active processes (like transcytosis). 23. What happens during transcytosis in capillaries? During transcytosis, substances are transported across endothelial cells in vesicles. 24. How does increased capillary pressure (Pc) lead to edema? Increased capillary pressure promotes more fluid to leak into the interstitial space, resulting in edema. 25. What is the relationship between capillary filtration and blood volume? An increase in blood volume can raise capillary filtration, contributing to edema. 26. What is the physiological significance of maintaining adequate capillary exchange? Adequate capillary exchange is essential for delivering nutrients, removing wastes, and maintaining tissue homeostasis. 27. What can happen to fluid exchange if the lymphatic system is obstructed? Obstruction of the lymphatic system can lead to fluid accumulation and swelling in the tissues (edema). 28. How do inflammatory responses affect capillary permeability? Inflammatory responses can increase capillary permeability, allowing more fluid and proteins to enter the interstitial space. 29. What is the effect of high blood pressure on capillary filtration? High blood pressure increases capillary filtration, potentially leading to edema. 30. What physiological conditions can contribute to decreased reabsorption in capillaries? Conditions such as liver disease, protein deficiency, and increased vascular permeability can lead to decreased reabsorption. VENOUS RETURN – DR. JONES (PPT NO. 16) 1. What is the primary function of veins in the circulatory system? Veins return blood to the heart. 2. What type of resistance do veins offer? Veins are low resistance vessels. 3. What is the pressure level in veins? Veins operate under low pressure. 4. What does high compliancy in veins mean? It means veins can stretch easily to accommodate varying volumes of blood. 5. What role do veins play in blood volume? Veins serve as a blood reservoir. 6. How does venous return affect the heart? Venous return determines how much blood enters the heart, influencing preload and end-diastolic volume (EDV). 7. What is the relationship between cardiac output and venous return? Cardiac Output equals Venous Return. 8. What is the pressure gradient's role in maintaining venous return? The pressure gradient allows blood to flow back to the heart, with very low pressure on the venous side. 9. Why is the right atrium significant in venous return? The right atrium has the lowest pressure, facilitating blood return from the veins. 10. How does the skeletal muscle pump assist venous return? The skeletal muscle pump massages veins during muscle contraction, increasing forward pressure. 11. What happens to blood in the veins during muscle relaxation? Blood will pool in the veins when muscles are relaxed. 12. Describe the respiratory pump's effect on venous return. During inspiration, pressure in the right atrium decreases, enhancing venous return; during exhalation, pressure increases. 13. What impact does gravity have on venous return? Gravity can cause blood to pool in the veins of the lower extremities when standing. 14. How does blood distribution differ when lying down versus standing? Lying down leads to even blood distribution, while standing causes pooling in lower extremities. 15. What is a potential consequence of blood pooling in the veins? Increased capillary pressure (Pc) and the risk of edema. 16. What reflex helps manage blood pressure and venous return? The baroreceptor reflex. 17. What is preload in the context of cardiac function? Preload refers to the initial stretching of the cardiac muscle fibers prior to contraction, determined by venous return. 18. How does high compliance in veins contribute to their function? High compliance allows veins to accommodate larger volumes of blood without significantly increasing pressure. 19. What happens to venous return during heavy exercise? Venous return increases due to enhanced skeletal muscle pump activity. 20. What occurs in the right atrium during inhalation? The pressure in the right atrium decreases, promoting venous return. 21. What happens to venous return during exhalation? The pressure in the right atrium increases, which can decrease venous return temporarily. 22. What is the effect of constriction of veins on venous return? Constriction increases venous return by reducing the volume of the compliant veins. 23. What is a mechanism that can counteract the effects of gravity on venous return? The skeletal muscle pump. 24. What role does the diaphragm play in venous return during breathing? The diaphragm's movement affects thoracic pressure, influencing the respiratory pump's effectiveness. 25. How can prolonged standing lead to edema? Prolonged standing can lead to pooling of blood in the legs, increasing capillary pressure and resulting in edema. 26. What is the primary determinant of how much blood enters the heart? Venous return. 27. What can happen if venous return is impaired? Impaired venous return can lead to decreased cardiac output and inadequate blood flow to tissues. 28. How do veins maintain their function despite low pressure? Veins rely on mechanisms such as muscle contraction and respiratory movements to aid venous return. 29. What is the significance of the pressure gradient in venous return? The pressure gradient is essential for blood to flow from the veins back to the heart. 30. Why is the skeletal muscle pump particularly important for individuals who are inactive? It helps facilitate venous return in the absence of significant muscle activity, preventing blood pooling and associated complications. BLOOD PRESSURE – DR. JONES (PPT NO. 17) 1. What is blood flow directly proportional to? Blood flow is directly proportional to the pressure gradient. 2. What is blood flow indirectly proportional to? Blood flow is indirectly proportional to the resistance of the vessel. 3. What is the formula for blood flow (F)? F = Delta P/R where Delta P is the pressure gradient and R is resistance. 4. How is the pressure gradient generated in the vasculature? The pressure gradient is generated by the heart's pumping action, particularly in the aorta. 5. What is laminar flow? Laminar flow is the flow of a fluid through narrow tubes where the velocity is lowest near the edges. 6. When does turbulent flow occur? Turbulent flow occurs when the velocity is too high or the tube is too large, though it usually does not occur in healthy vessels. 7. What is the Poiseuille-Hagen equation used for? The Poiseuille-Hagen equation is used to determine the factors altering resistance in blood flow. 8. What factors influence resistance according to the Poiseuille-Hagen equation? Resistance is influenced by viscosity and vessel radius. 9. What are arterioles referred to as in terms of their function? Arterioles are referred to as resistance vessels because they change peripheral resistance. 10. What is the role of veins in circulation? Veins are capacitance vessels that can stretch to accommodate large changes in volume without causing a large increase in pressure. 11. What is the formula for Mean Arterial Pressure (MAP)? MAP can be calculated using MAP = DP + 1/3 (SP – DP) or MAP = 0.6 (DP) + 0.4 (SP). 12. What does DP stand for in the context of blood pressure? DP stands for diastolic pressure. 13. What does SP stand for in blood pressure measurements? SP stands for systolic pressure. 14. How does gravity affect Mean Arterial Pressure (MAP)? Gravity can influence MAP by affecting the distribution of blood in the body, particularly when standing. 15. What is a sphygmomanometer used for? A sphygmomanometer is used to measure blood pressure. 16. What is pulse pressure (PP)? Pulse pressure is the difference between systolic pressure and diastolic pressure. 17. Why is it important to understand the relationship between flow, pressure, and resistance? Understanding this relationship is crucial for diagnosing and treating cardiovascular conditions. 18. What effect does vessel radius have on resistance? A larger vessel radius decreases resistance, allowing for increased blood flow. 19. How does viscosity affect resistance in blood vessels? Higher viscosity increases resistance, making it harder for blood to flow. 20. What happens to blood flow when resistance increases? When resistance increases, blood flow decreases if the pressure gradient remains constant. 21. What are the two main types of blood flow in vessels? Laminar flow and turbulent flow. 22. How do arterioles regulate blood pressure? Arterioles can constrict or dilate, thus changing peripheral resistance and regulating blood pressure. 23. What is the clinical significance of measuring MAP? MAP is a critical indicator of perfusion pressure and is important in assessing organ function. 24. What condition can result from changes in blood viscosity? Conditions such as dehydration or polycythemia can lead to increased blood viscosity and resistance. 25. How does an increase in heart rate affect blood pressure? An increase in heart rate can raise blood pressure by increasing cardiac output. 26. What role do the kidneys play in regulating blood pressure? The kidneys help regulate blood pressure by controlling blood volume and releasing hormones such as renin. 27. What happens to pulse pressure during exercise? Pulse pressure typically increases during exercise due to higher systolic pressure. 28. Why is it essential to monitor blood pressure in clinical settings? Monitoring blood pressure is essential for diagnosing hypertension and other cardiovascular issues. 29. What physiological changes occur in response to low blood pressure? The body may increase heart rate, constrict blood vessels, and release hormones to raise blood pressure. 30. What is the significance of understanding blood flow dynamics in physiology? Understanding blood flow dynamics is crucial for diagnosing and managing cardiovascular diseases and conditions. BLOOD PRESSURE REGULATION BY CARDIAC OUTPUT DR JONES (PPT NO. 18) 1. What does Mean Arterial Blood Pressure (MAP) represent? MAP represents the average pressure in a person's arteries during one cardiac cycle. 2. How is cardiac output defined? Cardiac output is the total volume of blood pumped by the ventricles in one minute. 3. What is the formula for calculating cardiac output (CO)? CO = Heart Rate (HR) x Stroke Volume (SV). 4. What factors control cardiac output? Cardiac output is controlled by neurological and chemical factors. 5. What is the intrinsic firing rate of the SA node? The intrinsic firing rate of the SA node is approximately 100 beats per minute (bpm). 6. What is tachycardia? Tachycardia is an increase in heart rate above 100 bpm. 7. What is bradycardia? Bradycardia is a decrease in heart rate below 60 bpm. 8. What are chronotropic agents? Chronotropic agents are substances that affect heart rate; positive agents increase it, while negative agents decrease it. 9. What is the role of the sympathetic nervous system in heart rate regulation? The sympathetic nervous system increases heart rate through norepinephrine and β1-adrenergic receptors. 10. How does norepinephrine affect the SA node? Norepinephrine increases the current of F-type Na+ channels and Ca2+ channels, leading to increased heart rate. 11. What is the role of the parasympathetic nervous system in heart rate regulation? The parasympathetic nervous system decreases heart rate through acetylcholine and muscarinic receptors. 12. How does acetylcholine affect heart rate? Acetylcholine activates K+ channels and inhibits adenylate cyclase, reducing heart rate. 13. What is the resting heart rate primarily influenced by? The resting heart rate is primarily influenced by parasympathetic activity. 14. What is the baroreceptor reflex? The baroreceptor reflex is a mechanism that senses changes in arterial blood pressure and helps regulate blood pressure. 15. What type of receptors are baroreceptors? Baroreceptors are stretch receptors that detect changes in arterial blood pressure. 16. What happens when baroreceptors detect an increase in arterial blood pressure? An increase in arterial blood pressure leads to increased action potentials to cardiac centers, resulting in increased parasympathetic output. 17. What factors control stroke volume (SV)? Stroke volume is controlled by preload, contractility, and afterload. 18. What is preload? Preload is the volume of blood returned to the heart before contraction. 19. How does increased preload affect stroke volume? Increased preload stretches cardiac myocytes, leading to an increase in stroke volume according to the Frank- Starling law. 20. What is contractility? Contractility refers to the force of contraction of the heart muscle (myocytes) for a given preload. 21. What are positive inotropic agents? Positive inotropic agents increase contractility by increasing cAMP and cytoplasmic Ca2+ levels. 22. What can be classified as negative inotropic agents? Negative inotropic agents include conditions such as increased PCO2 (decreased pH), decreased PO2, and hyperkalemia. 23. How does adrenergic stimulation affect contractility? Adrenergic stimulation increases contractility by promoting calcium release from the sarcoplasmic reticulum. 24. What is afterload? Afterload is the pressure that the ventricles must overcome to eject blood into the aorta. 25. How does increased afterload affect stroke volume? Increased afterload decreases stroke volume, as the heart must work harder against higher pressures. 26. What is the significance of understanding cardiac output? Understanding cardiac output is essential for assessing heart function and overall cardiovascular health. 27. What is the relationship between stroke volume and cardiac output? Stroke volume and heart rate together determine cardiac output, influencing blood pressure and tissue perfusion. 28. What role does the cardiovascular system play in blood pressure regulation? The cardiovascular system regulates blood pressure through adjustments in cardiac output and vascular resistance. 29. What physiological changes can lead to increased cardiac output? Increased heart rate and/or increased stroke volume lead to increased cardiac output. 30. How does the body respond to low blood pressure through cardiac output regulation? In response to low blood pressure, the body may increase heart rate and stroke volume to restore normal blood pressure levels. CARDIOVASCULAR: VASCULAR CONTROL AND REFLEXES DR. JONES (PPT NO. 19) 1. What is the primary purpose of neural control in the cardiovascular system? The primary purpose is the regulation of blood pressure. 2. How does the body respond to hemorrhage? The body initiates compensatory mechanisms to maintain blood pressure and blood flow to vital organs. 3. What physiological changes occur during hemorrhage? Increased heart rate, vasoconstriction, and increased release of hormones like norepinephrine occur. 4. What is cardiac output (CO) during intense exercise? Cardiac output can increase to 35 L/min during intense exercise. 5. How does the cardiovascular system adapt during exercise? Increases in heart rate and stroke volume occur to supply more oxygen to muscles. 6. What role do baroreceptors play in blood pressure regulation? Baroreceptors detect changes in blood pressure and send signals to the central nervous system to initiate responses. 7. What happens to peripheral resistance during exercise? Peripheral resistance generally decreases to allow for increased blood flow to active muscles. 8. How does blood flow distribution change during exercise? Blood flow is redirected from non-essential organs to active muscles. 9. What compensatory mechanisms occur in response to blood loss during hemorrhage? Increased heart rate, increased systemic vascular resistance, and fluid retention occur. 10. What is the role of the sympathetic nervous system during hemorrhage? The sympathetic nervous system increases heart rate and causes vasoconstriction to maintain blood pressure. 11. How does the body maintain blood pressure during severe hemorrhage? The body activates the renin-angiotensin-aldosterone system to retain fluid and increase blood volume. 12. What changes occur in heart rate during exercise? Heart rate increases significantly to meet the heightened oxygen demand of working muscles. 13. What is the relationship between exercise intensity and cardiac output? Cardiac output increases with exercise intensity to supply oxygen and nutrients to muscles. 14. What happens to blood viscosity during hemorrhage? Blood viscosity may increase due to lower blood volume, affecting flow dynamics. 15. How does the cardiovascular system respond to standing up quickly? Baroreceptors detect the drop in blood pressure and stimulate the sympathetic nervous system to maintain blood flow. 16. What changes occur in blood vessel diameter during exercise? Blood vessels in active muscles dilate to increase blood flow, while others may constrict. 17. What is the effect of hemorrhage on organ perfusion? Organ perfusion may decrease, especially to non-essential organs, as the body prioritizes vital functions. 18. How does the body utilize the skeletal muscle pump during exercise? The skeletal muscle pump helps return venous blood to the heart, aiding in maintaining cardiac output. 19. What are the main hormones involved in blood pressure regulation during hemorrhage? Norepinephrine, epinephrine, and angiotensin II are key hormones involved. 20. What impact does exercise have on overall vascular resistance? Overall vascular resistance decreases, allowing for greater blood flow to active muscles. 21. How does the respiratory system assist the cardiovascular system during exercise? Increased respiration improves oxygen intake and carbon dioxide removal, supporting increased cardiac output. 22. What is the significance of stroke volume during exercise? Stroke volume increases to enhance the amount of blood ejected from the heart with each beat. 23. What role do chemoreceptors play in vascular control? Chemoreceptors detect changes in blood chemistry (like CO2 and O2 levels) and help regulate cardiovascular responses. 24. How does chronic exercise training affect resting heart rate? Chronic exercise training typically leads to a lower resting heart rate due to improved cardiovascular efficiency. 25. What is the immediate cardiovascular response to hemorrhage? The immediate response includes increased heart rate and vasoconstriction to maintain blood pressure. 26. How does the body compensate for decreased blood volume during hemorrhage? The body retains sodium and water through hormonal mechanisms to restore blood volume. 27. What cardiovascular adaptations occur with regular exercise? Enhanced cardiac efficiency, increased capillary density, and improved oxygen delivery to tissues. 28. What cardiovascular reflexes are triggered by sudden drops in blood pressure? Baroreceptor reflexes that increase heart rate and systemic vascular resistance. 29. How does prolonged exercise affect the body's thermoregulation? Increased blood flow to the skin assists in heat dissipation through sweating. 30. Why is monitoring blood pressure during exercise important? It helps assess cardiovascular health and ensures that blood pressure remains within a safe range during physical activity.