Module 4: Cell Cycle Regulation & DNA Replication PDF
Document Details
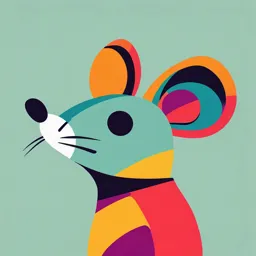
Uploaded by CelebratoryGladiolus282
Rhodes College
2024
Tags
Summary
This document, titled Module 4, discusses the regulation of the cell cycle and DNA replication. It covers topics including cyclins, CDKs, cell cycle checkpoints, and DNA replication models, along with relevant concepts such as oncogenes, tumor suppressor genes, and growth factors. The document was created on November 19, 2024.
Full Transcript
Module 4 Created @November 19, 2024 Tags Bio once a cell reaches the checkpoint in the S phase (G1-S transition) they are committed to division mitosis—making a second nucleus cytokinesis—making the sec...
Module 4 Created @November 19, 2024 Tags Bio once a cell reaches the checkpoint in the S phase (G1-S transition) they are committed to division mitosis—making a second nucleus cytokinesis—making the second cell interphase&m-phase metaphase-anaphase transition (in m-phase) G2 checkpoint (G2-M transition): quality control Regulation of the Cell Cycle Regulating the cell cycle Module 4 1 early insight into cell cycle regulatory mechanisms came from cell fusion experiments. when cells in S phase were fused with cells in G1, the G1 nucleus immediately began replicating its DNA. when cells in M phase were fused with cells in G1, the G1 nucleus began mitosis (even though it only has a single copy of its genome) Cyclins and CDKs cytoplasmic factors from fusion experiments were called cyclins, because their expression peaks at certain phases of the cell cycle. when present, cyclins bind to and activate cyclin-dependent kinases (Cdk). The cyclin-Cdk complex the active cyclin-Cdk complex orchestrates the events of the next phase of the cell cycle. this is accomplished through phosphorylation of a specific set of target proteins. Cyclins G1 / S cyclins - commit the cell to S phase at the end of G1. S cyclins - direct the initiation of DNA replication. M cyclins - orchestrate the events of mitosis. a fourth cyclin called G1 cyclins helps the cell progress through the G1 checkpoint but is not present in all cells. Controlling the cyclins the Cdks that drive the cell cycle are often present throughout the cell cycle, but are inactive without their specific cyclin. the major mechanism for controlling the cyclin concentration is selective proteolytic destruction. this selective destruction is mediated by the ubiquitinoylation and proteosomal degradation Module 4 2 The G2 checkpoint M-cyclins bind to their Cdk to establish the M-Cdk complex (aka maturation-promoting factor, or MPF). M-cyclin begins accumulating at the end of S phase and continues throughout G2 phase. once it’s reached a certain abundance, M-Cdk triggers M phase through a sharp increase in MPF activity, and the cell passes the G2 checkpoint. at a certain point in M-phase, M-cyclin is degraded, MPF activity ceases, and the daughter cells progress into G1 phase. M-Cdk sets the stage for mitosis breakdown of the nuclear lamina - M-CdK phosphorylates the lamin molecules of the nuclear lamina, causing it to disintegrate. chromatin condensation - M-Cdk activates condensin molecules that condense the chromatin into chromosomes. formation of the mitotic spindle - M-Cdk also phosphorylates microtubule- associated proteins which direct the formation of the spindle Cancer “neoplastic transformations” External factors can influence the cell cycle Module 4 3 cells commit themselves to cell division when they receive external signals telling them to divide—these signals are called growth factors one example is platelet-derived growth factor (PDGF), which induces cell division in fibroblasts PDGF induces a signal transduction cascade that allows fibroblasts to pass the G1 checkpoint. when an injury occurs, platelets in the blood stimulate cell division of nearby fibroblasts by releasing PDGF Anchorage-dependence Module 4 4 most mammalian cells need to attach to a substrate before they can grow and divide—this is called anchorage-dependence anchorage-dependence keeps cells from growing independently of the other cells / ECM of their home tissue Density-dependent inhibition cells also receive external signals that inhibit their progression through the cell cycle once a cell culture (or tissue) has grown to a certain density (usually as a monolayer), the cells stop dividing—this is called density-dependent inhibition contact with neighboring cells mediated through cell adhesion molecules and additional signaling molecules that prevent passage past the G1 checkpoint Module 4 5 Cancer cells in culture cancer cells usually lose their anchorage-dependence and density-dependent inhibition this allows them to proliferate within the tissue and establish tumors, which can become very large Oncogenes oncogenes code for proteins that send an inappropriate positive growth signal. Ras is a G-protein that relays growth factor signals from the membrane to the nucleus. if Ras becomes hyperactive, the growth factor signal becomes independent of the growth factor—the cell is one step closer to becoming cancerous. Proto-oncogenes proto-oncogenes are normal genes that are somehow involved with positive growth signals. they can be converted to oncogenes through several mechanisms, including: control by an inappropriate promoter, gene amplification, or point mutations that lead to over-expression or hyperactivity. Tumor suppressor genes tumor suppressor genes code for proteins that send negative growth signals. p53 binds to damaged regions of DNA and inhibits the progression through the cell cycle until the damage has been repaired. when a tumor suppressor gene like p53 becomes inactive, the cell is more likely to divide inappropriately. The multi-step transformation process a single mutation is rarely sufficient to transform a cell into a cancerous cell however, mutations add up, and if the cell begins to express oncogenes and then loses tumor suppressor gene expression, the cell cycle will become dysregulated Module 4 6 dysregulation of the cell cycle is extremely dangerous, as uncontrolled cell division leads to tumors and cancer Beyond transformation tumors often become large enough that they need their own blood supply, and it can subvert the circulatory system in a process called angiogenesis. once a tumor has access to the vasculature, it has a way to escape its home tissue and colonize distant tissues through metastasis DNA Replication The cell cycle Cell’s must duplicate their genomes when cells divide, they require two copies of their genomes; one for the parent cell, and one for the daughter cell. Module 4 7 before the cell can divide, it must undertake the Herculean task of duplicating its entire genome. moreover, it must perform this duplication with a great deal of fidelity What does duplicating a genome entail? first there must be some well-regulated process that initiates the replication process. next, full copies of the entire genome must be made. his new copy must be a perfect (or nearly perfect) copy of the original Semi-conservative Replication Replication replication begins when the double helix is opened (the individual strands are separated). next, a complementary daughter strand is synthesized for each parental strand. these two daughter strands will be identical to the “missing” parental strand Module 4 8 Three models for DNA replication conservative - the two parental strands reunite to form one double helix and the two daughter strands form another. semi-conservative - the two parental strands form “hybrid” double helices with their daughter strands. dispersive - each strand is a mixture of parental and daughter strands. This model is unlikely, because it requires strand breaks. Meselson and Stahl in 1958, Mathew Meselson and Franklin Stahl performed an important experiment to discriminate between the three possible models of DNA replication. it is commonly referred to as the Meselson–Stahl experiment and is often called the most elegant experiment in all of biology. it’s a great example is experimental science in action! The Meselson-Stahl experiment E. coli was grown in media containing either light (14N) or heavy (15N) isotopes of nitrogen. when the DNA from each sample was centrifuged, they could resolve the difference between heavy and light DNA. Module 4 9 bacteria from the 15N media were transferred to 14N media and allowed to replicate their DNA. The first replication following the first replication in the light nitrogen, Meselson and Stahl observed a single band of intermediate weight. this excludes the conservative model, which predicts two separate bands The second replication following the second replication, they saw two bands: one of intermediate density and one that was light. this excludes the dispersive model, which predicts a single band which would be lighter than the intermediate band (as the original heavy DNA is distributed among new strands). these observations support the semi-conservative replication. Module 4 10 DNA Replication in Prokaryotes DNA replication in prokaryotes replication begins at an origin of replication with the formation of a replication bubble or replication fork. the replication fork is simply a region of DNA where the parental strands have been separated so that the replication machinery can access each strand. replication proceeds in both directions until two complete circular chromosomes have been made. bacterial replication is called theta replication because it looks like the Greek letter theta. Residents of the replication fork Module 4 11 Helicase the parental strands must be separated before they can direct the synthesis of daughter strands. enzymes called helicases perform this function. these are large, ring-like protein complexes that encircle a single strand of DNA—think of them like ATP-driven bulldozers that separate strands of DNA. Topoisomerase Module 4 12 when the parental DNA strands are separated, it causes over-winding in front of the replication fork. this over-winding can present a significant obstacle for helicases trying to push the replication fork ahead, and eventually they won’t be able to proceed. enzymes called topoisomerases relieve this tension and allow the replication fork to move along the entire molecule. Topoisomerase topoisomerase relieves tension in DNA molecules by allowing them to freely rotate. topoisomerase binds and nicks the backbone of one strand, creating a free 3’ end that can freely rotate. once the tension in the molecules has been relieved, topoisomerase unbinds, and the backbone is ligated back together. missed day of thanksgiving break Mitosis The G2 checkpoint regulating the cell cycle the cell cycle is regulated by three main checkpoints. Module 4 13 this is how the cell to makes sure it has fully completed all prerequisite steps before going on to the next phase of the cell cycle. now we turn our attention to the G2 checkpoint and M phase. The G2 checkpoint the G2 checkpoint regulates the progression from G2 into M phase. once it commits to M phase, the cell will divide its genetic material between two nuclei and separate into two daughter cells. the entry into M phase is determined by the presence of M-cyclin, which binds to its Cdk to establish the M-Cdk complex (also called MPF). M-Cdk sets the stage for mitosis breakdown of the nuclear lamina - M-CdK phosphorylates the lamin molecules of the nuclear lamina, causing it to disintegrate. chromatin condensation - M-Cdk activates condensin molecules that condense the chromatin into chromosomes. formation of the mitotic spindle - M-Cdk also phosphorylates microtubule- associated proteins which direct the formation of the spindle. the nuclear lamina the nuclear lamina is made of an intermediate filament protein called lamin. M-Cdk directly phosphorylates lamin, resulting in the disintegration of the nuclear membrane—this is a critical early step in mitosis. chromatin condensation we have already discussed the condensation of chromatin several times in this course. the condensation of chromatin into chromosomes leads to the discrete distribution of genetic material between cells. condensin and cohesin Module 4 14 M-Cdk activity leads to the condensation of chromosomes by activating condensin complexes. condensin is a dimer that holds coils of DNA together. sister chromatids are held together by a similar protein called cohesin. cohesin is cleaved by an enzyme at a critical stage of mitosis resulting in the rapid dissociation of sister chromatids. microtubules it’s been a while, but you should remember that microtubules are hollow cytoskeletal filaments that are ~ 25 nm in diameter. microtubules are composed of dimers of α and β-tubulin. microtubules are polarized molecules, which distinct plus and minus ends. the microtubule grows by adding tubulin dimers to the plus end centrosomes Module 4 15 centrosomes, the microtubule organizing centers of cells, contain two centrioles positioned at right angles to each other connected by a protein-rich matrix. the centrosome plays a fundamental role in mitosis, as each centrosome forms a pole of the spindle apparatus. not surprisingly, M-Cdk activity induces the formation of the spindle apparatus the centrosome cycle during the end of G1, the two centrioles separate, and at the beginning of S phase, a daughter centriole forms at the base of the mother centriole. at the beginning of M phase, each centrosome forms an aster of microtubules prior to the disintegration of the nuclear membrane. Mitosis M phase each phase characterized by the activity of the chromosomes, spindle apparatus, and nucleus. mitosis is complete once a new nucleus has formed at the end of telophase, but an additional step called cytokinesis is required to separate the two daughter cells. Module 4 16 M phase therefore consists of two general, but related processes: mitosis and cytokinesis. interphase during interphase, the cell prepares for mitosis by replicating its DNA and centrosomes. the nucleus is intact and the chromatin inside has not condensed into chromosomes. M-Cdk activity hasn’t reached critical mass yet prophase the main event of prophase is the condensation of the interphase chromatin into mitotic chromosomes. Module 4 17 a more subtle change is that the centrosomes begin migrating to either side of the nucleus in anticipation of forming the mitotic spindle. the nuclear membrane, however, remains intact. prometaphase following the chromosome condensation and the migration of the centrosomes, the nuclear membrane disintegrates. this is a critical event because the microtubules emanating from the centrosomes can now attach to the chromosomes. at this stage other organelles of the endomembrane system such as the ER and Golgi apparatus become fragmented. kinetochores the kinetochore is a protein plaque that forms on the centromere of each chromosome. the plus ends of microtubules emanating from the spindle apparatus bind very stably to the kinetochore. these microtubules are therefore called kinetochore microtubules metaphase Module 4 18 once the kinetochore microtubules have attached, they push and pull each chromosome until all chromosomes are lined up in a row. this is called the metaphase plate, and it is an important structure that lies halfway between the poles of the spindle apparatus. the orientation of the metaphase plate will determine the plane of division later on. microtubules of the spindle apparatus kinetochore microtubules - physically bind to the chromosome through the kinetochore. overlap microtubules - overlap with microtubules from the other pole and are connected to each other by motor proteins. astral microtubules radiate out from the poles of the spindle apparatus in an aster (star) pattern the spindle attachment checkpoint there’s one last checkpoint located within M phase called the spindle attachment checkpoint. here, unattached kinetochores delay the transition from metaphase to anaphase. if the cell progressed into anaphase before all of the chromosomes were attached to the spindle, genetic chaos would ensue. the metaphase-to-anaphase transition progression through the spindle attachment checkpoint is driven by a proteolytic complex called the anaphase promoting complex (APC). Module 4 19 APC cleaves an inhibitory protein called securin, which allows a protease called separase to cleave the cohesion complex that holds sister chromatids together. APC also degrades M-cyclin, which eliminates MPF (M-Cdk) activity. anaphase anaphase is the critical step where the cell actively partitions its genetic material. once the sister chromatids have separated, they begin their migration toward the spindle poles, which requires a significant amount of coordination between microtubules and motor proteins. anaphase A Module 4 20 the chromosomal migration toward the poles occurs in by two sequential mechanisms called anaphase A and anaphase B. anaphase A is characterized by the retraction of the kinetochore microtubules, which pull the chromosomes toward each pole. think of anaphase A like reeling in a fish. anaphase B anaphase B is characterized by the separation of the poles themselves, which occurs by two mechanisms. motor proteins push the overlap microtubules apart, which help to separate the poles. motor proteins on the astral microtubules connect with the actin cytoskeleton and help pull the poles apart. telophase Module 4 21 telophase begins once the daughter chromosomes complete their migration and reach the poles. once this occurs, a nuclear envelope forms around each pole (remember that M-cyclin has been degraded by this point). telophase marks the end of mitosis but not the end of M phase. a contractile ring begins to form on the inner surface of the plasma membrane that will finally partition the cell. Cytokinesis cytokinesis the next stage in cell division involves partitioning the cell in two through a process called cytokinesis. Module 4 22 during anaphase B, the poles of the spindle were pushed farther and farther apart by the overlap microtubules. remnants of the overlap microtubules remain in between the nuclei, although they are no longer connected to the poles. the contractile ring cytokinesis requires the formation of a contractile ring that pinches the cell in half. the first obvious sign of cytokinesis is the formation of the cleavage furrow, which quickly deepens as the contractile ring constricts. like the contractile apparatus of muscle, this contractile ring is made of actin and myosin. the midbody near the end of cytokinesis, the two daughter cells are separated by a tiny bridge called the midbody. a mother centriole from a daughter cell separates from the daughter centriole, migrates into the midbody and stays there for some time before returning to the daughter cell—the reason for this strange behavior is not known. once division is complete, the cells truly separate and the structures of the midbody leave a mark on the inside of the plasma membrane. Module 4 23 continuity of the cell cycle once the cell has divided each new cell is in G1. the activity of M-Cdk is reduced by the degradation of M-cyclin by the APC complex during mitosis, and is kept at a low level by the continued degradation of M-cyclin during G1. mitosis without cytokinesis when mitosis is not followed by cytokinesis, a multi-nucleated cell called a syncytium is formed. immediately following fertilization in drosophila, 13 nuclear divisions take place without cytokinesis—this generates ~ 6000 nuclei in a single cell. these nuclei migrate to the periphery of the cell, where one massive round of cytokinesis occurs through a process called cellularization. Module 4 24 cytokinesis in plants the cell wall of plant cells presents an obvious challenge for cytokinesis. the two daughter cells simply wall themselves off by producing a structure called the cell plate. the cell plate is enveloped by a plasma membrane, and grows until it completely separates the two daughter cells. -cellulose microfibrils are then added to the matrix of the cell plate. Mutation natural selection Module 4 25 natural selection is the process by which individuals with inherited traits become evolutionarily more fit because of those traits. but natural selection only works, if there’s a genetically diverse population to select from—so how do we produce a genetically diverse population. mutation changes to the genetic code are called mutations, which comes from the Latin word for “change.” mutations can be beneficial, detrimental, inconsequential, or even lethal. the important point is that no matter what the phenotypic outcome of the mutation, it is inherited by the mutated cell’s lineage. Replication mistakes, DNA damage, and repair mutation rates Module 4 26 mutations occur at relatively fixed rates in most organisms (e.g., the mutation rate in E. coli is ~ 1 in 109, or 1 in a billion nucleotides). E. coli’s genome is ~ 4.6 Mb (4.6 million bps), which means that it is likely to faithfully replicate its genome without any mistakes in each division. how does E. coli maintain such a low mutation rate? DNA polymerases faithfully replicate the genetic code—except when they don’t! just how good are DNA polymerases at picking the correct deoxyribonucleotide? what happens if they make a mistake? where do these mistakes come from? very rarely, an incorrect base looks and feels just like the correct base to the polymerase. tautomers ketones exist in equilibrium with enols (upper right), and together, the pair are called tautomers this can also apply to double-bonded amino groups, but we won’t discuss this. tautomeric shifts occur very rarely in the ketone groups of the nitrogenous bases tautomeric shifts about one in105 bases that DNA polymerase grabs will be in its enol form. the enol form is a bit like the keto form’s evil twin, as it can base pair differently than the keto form. not surprisingly, this is a how incorrect nucleotides can wind up in DNA. the proofreading polymerase Module 4 27 DNA polymerase III can proofread and edit its own work using an editing site within a subunit of the enzyme. if the enol form of a tautomer is added, it quickly shifts back to its keto form and the refuses to bind its partner. DNA polymerase III cannot add another nucleotide to an unpaired 3’ end, so it excises the base and tries again. 3’ to 5’ exonucleolytic proofreading Module 4 28 here, DNA polymerase has added a cytosine residue where a thymine residue was needed. this was because of a tautomeric shift, which has since reversed, resulting in an unpaired 3’ end. DNA polymerase cannot elongate this 3’ end. it then uses its 3’ to 5’ exonucleolytic activity to “take a step back,” and remove this mis-paired base. the correct base (thymine) is then added and the strand continues to be elongated. the benefit of proofreading adding in the proofreading ability of DNA polymerase III brings our mutation rate down to ~ 1 error in 107 bases. but the measured mutation rate is 1 in 109 bases, where does this last 100- fold drop in the mutation rate come from? Module 4 29 spontaneous damage long after the polymerase is gone, good bases can spontaneously go bad. when you have a genome comprised of billions of base pairs, something is bound to happen to some of them. spontaneous damage to the nitrogenous bases can add up, leaving the genome riddled with mutations. spontaneous damage: depurination the most common form of spontaneous damage is depurination. sometimes, the glycosidic bond between a purine (adenine and guanine) break and the nucleotide is left without an “identity.” a human cell loses ~ 5000 purines / day simply due to spontaneous depurination. spontaneous damage: deamination Module 4 30 another form of spontaneous damage is deamination cytosine residues commonly lose their amino group and become uracil the genome of a human cell undergoes ~ 100 deamination / day consequences of spontaneous damage if left uncorrected, deamination leads to a guanine residue being replaced with an adenine residue in one of the daughter DNA strands—this mutation is called a transition. depurination leads to a different kind of mutation, where a base pair is deleted in one of the daughter strands—this is called a frameshift mutation. base excision repair Module 4 31 double-stranded DNA has a built- in “back-up” copy of the genetic code in the complementary strand. repair mechanisms that use the complementary strand to repair an inappropriate or damaged base are called strand-directed repair mechanisms. the repair mechanism for single- nucleotide damage to the genome is called base excision repair. here, the damaged base is removed, the sugar-phosphate backbone is broken, and the site is filled with the correct base. the benefit of strand-directed DNA repair strand-directed repair mechanisms further reduce the mutation rate 100-fold, by repairing any spontaneous or otherwise unavoidable damage to the genome. the final mutation rate is brought down to acceptable levels at ~ 1 in 109 (or 1 in a billion) nucleotides. Mutations nucleic acid substitutions Module 4 32 a purine-for-purine or pyrimidine- for-pyrimidine mutation is called a transition (e.g., adenine–guanine, or cytosine–thymine). a purine-for-pyrimidine or pyrimidine-for purine mutation is called a transversion (e.g., adenine–thymine, or adenine– cytosine). transitions are less disruptive to the structure of DNA, and occur more frequently than transversions. substitutions: silent mutations because of the redundancy of the genetic code, many nucleic acid substitutions do not change the corresponding protein’s primary structure— these are called silent mutations. in the example, there is a transition from G to A that leads to a GGU codon instead of GGC, but because both GGU and GGC code for glycine, the protein’s primary structure is unchanged. substitutions: missense mutation Module 4 33 a missense mutation occurs when a nucleic acid substitution changes the protein’s primary structure. in the example, a transition from cytosine to thymine leads to a change in the codon from GGC (glycine) to AGC (serine). the consequences of missense mutations can vary from being benign to disastrous depending on the chemical nature of the amino acid and its location in the protein. substitutions: nonsense mutation sometimes, a mutation changes a codon that codes for an amino acid into a stop codon—this is bad. this is called a nonsense mutation, and usually has serious consequences for the protein. nonsense mutations lead to truncated proteins, which usually cannot perform their intended function. frameshift-to-nonsense mutation (+ or - 1 or 2 bps) insertions and deletions can change the reading frame, resulting in frameshift mutations. in this case, a frameshift results in a stop codon immediately after the start codon. Module 4 34 frameshift mutations often produce long strings of “gibberish,” and truncations that can render a protein useless, or possibly lead to protein aggregation and harm the cell full-codon insertion or deletion (+ or - 3 bp) although insertion and deletion mutations are quite serious, a rare, but relatively benign form occurs when a full codon is either inserted or deleted. a 3-base insertion or deletion doesn’t change the reading frame, but adds or deletes a full amino acid from the protein’s primary sequence. mutations at the phenotype level lethal mutation - the mutation interferes with a critical gene, resulting in the death of the organism. conditional mutation - the phenotype associated with the mutation is only observed under certain conditions (e.g. at high temperatures). loss-of-function mutation (usually recessive) - a mutation results in a decrease in the gene product’s ability to perform its normal function. null mutation - a mutation that results in the complete loss of function of the gene product. gain-of-function mutation (usually dominant) - sometimes, a mutation leads to a gene product with novel properties. Mutagens mutagens mutagens are agents that increase the mutation rate above natural levels. Module 4 35 mutagens come in three general forms: physical, chemical, and viral (although we will not discuss tumor viruses). physical mutagens: pyrimidine dimers ultraviolet light induces a photochemical reaction between neighboring pyrimidines that forms a pyrimidine dimer (these are sometimes called thymine dimers as they often involve two thymine residues). DNA polymerases tend to get stuck on pyrimidine dimers and replication is arrested. even if a polymerases that can handle the pyrimidine dimer, it usually inserts an incorrect nucleotide, resulting in mutation. pyrimidine dimers are the genetic lesion most commonly associated with melanoma. nucleotide excision repair bulky lesions such as pyrimidine dimers are corrected by a mechanism called nucleotide excision repair. enzymes scan the DNA looking for distortions in the double helix. Module 4 36 the sugar-phosphate backbone is broken on each side of the lesion and a patch of surrounding nucleotides is removed by a helicase. the resulting gap is filled in by DNA polymerase with complementary nucleotides xeroderma pigmentosum (XP) some people have genetic defects in their nucleotide excision repair mechanism. this results in a condition called xeroderma pigmentosum, in which pyrimidine dimers from the UV component of sunlight results in skin cancer. these people develop disfiguring lesions on their faces and hands chemical mutagens: base-altering agents some chemical mutagens bind directly to the nucleotide bases, such as aflatoxin—one of the most mutagenic substances known. Aflatoxin B1 is produced by a fungus (Aspergillus flavus) that grows on grains and peanuts stored in humid tropical environments. by itself, aflatoxin isn’t a problem, but it is metabolized in the liver into an extremely mutagenic epoxide that forms adducts with the bases of DNA. chemical mutagens: intercalating agents are usually rigid planar aromatic molecules that slip in between adjacent base pairs. intercalating agents they cause slight bulges in the double helix that lead to frameshift mutations. one common intercalating agent is ethidium bromide, which is commonly used to visualize DNA in agarose gels—be careful quantifying mutagenicity: the Ames test Module 4 37 in the Ames test, a potential mutagen is mixed with a homogenized liver extract and a culture of histidine-dependent bacteria. the gene responsible for making histidine is non-functional (usually it’s out of frame), so these bacteria require supplemental histidine. a substance is considered mutagenic if it converts the non-functional histidine gene into a functional gene ubiquita/proteosome Gametogenesis and Fertilization Meiosis Gametogenesis casual inspection of the male and female gametes in this micrograph suggests there must be more to the formation of gametes than simply producing haploid cells the production of gametes is referred to as gametogenesis in both males and females Meet the gametes: sperm sperm are tiny streamlined motile cells designed to carry paternal genes. Module 4 38 the head contains the acrosomal vesicle and haploid nucleus. the acrosomal vesicle is loaded with hydrolytic enzymes necessary for penetrating the egg’s coat. the midpiece is loaded with mitochondria to provide the energy required to drive the flagellum. the flagellum is the structure that confers motility to the sperm The testes the male reproductive system is designed to produce and deliver sperm. in the testes, sperm production occurs within the seminiferous tubules in an outward-to-inward orientation. unlike the female reproductive system, males produce a tremendous quantity of sperm continuously throughout their lives after they reach puberty. Spermatogenesis spermatogonia continually divide by mitosis, and some of the resulting daughter cells become primary spermatocytes. primary spermatocytes pass through meiosis I, resulting in two haploid secondary spermatocytes. secondary spermatocytes complete meiosis II to make four spermatids. these spermatids differentiate into mature sperm and are released into the seminiferous tubule. note that most of the differentiation of a sperm occurs after the cells become haploid. Cytoplasmic bridges cytokinesis following meiosis I and meiosis II remains incomplete, leaving cytoplasmic bridges connecting the cytoplasms of spermatids derived from the same spermatogonium. this is important because survival and further differentiation require genes made from the X chromosome, and some spermatids only have a Y Module 4 39 chromosome. by sharing a cytoplasm, each spermatid is exposed to the gene products of the X chromosome for as long as possible. Meet the gametes: eggs eggs (or ova) are gigantic gametes, that can be surprisingly large (imagine an ostrich egg). mammalian babies have access to their mother’s nutrients while they develop, whereas birds and reptiles develop outside of their mothers and require a large source of “pre-packaged” nutrients. the egg contains a haploid pronucleus along with many organelles and nutrients in the cytoplasm The female reproductive system the female reproductive system consists of the ovaries, Fallopian tubes, uterus, cervix and vagina. mature oocytes (eggs) are produced in the ovaries one at a time, and released into the Fallopian tubes to await fertilization. a fertilized egg will implant into the wall of the uterus and develop into a fetus. Oogenesis: before puberty oogenesis begins with the mitotic division of oogonia inside the ovary to establish primary oocytes. primary oocytes begin meiosis I but are arrested in this stage until sexual maturity. this all happens prior to birth, and during the intervening time, the primary oocyte develops a coat and cortical granules. Oogenesis: after puberty after puberty, primary oocytes are individually selected for further maturation. primary oocytes complete meiosis I by dividing asymmetrically to produce a polar body (that later degenerates) and a secondary oocyte. Module 4 40 the secondary oocyte is arrested in metaphase II and is released upon ovulation, waiting to be fertilized. meiosis II is completed after fertilization, producing a second polar body. Fertilization - although we commonly refer to fertilization as the fusion of sperm and egg, the real definition is the formation of a diploid nucleus from the pronuclei from each gamete. The acrosomal reaction mammalian eggs are covered by a glycoprotein coat called the zona pellucida. upon binding to the proteins of the zona pellucida, the sperm releases the hydrolytic enzymes of its acrosomal vesicle to digest the the zona pellucida. this is called the acrosomal reaction, and it allows the sperm to penetrate the egg’s coat and finally reach the plasma membrane. The cortical reaction if two or more sperm fuse with the egg, the resulting cell will have too many chromosomes and won’t be viable— this is called polyspermy. vesicles loaded with hydrolytic enzymes are positioned around the egg’s periphery; these vesicles are called cortical granules. once the first sperm enters the egg, a wave of calcium crosses the entire cell leading to the fusion of the cortical granules with the plasma membrane. Module 4 41 hydrolytic enzymes destroy the zona pellucida and prevent additional sperm from binding—this is called the cortical reaction. Unification of the pronuclei after the entry of the sperm’s haploid nucleus, the two pronuclei remain separate until the first mitotic division. the sperm provides a centriole that joins with the egg’s centriole to form a centrosome. this new centrosome replicates, the DNA of each pronucleus is replicated, the nuclear envelope breaks down, and the first (of many) mitotic divisions begins —and fertilization is complete. and it’s off to the races… once fertilization is complete, the zygote undergoes a series of divisions and amazing changes that turn a simple cell (or ball of cells) into a complete animal. if you can, take an embryology course! Recombination Natural selection requires genetic diversity we saw earlier that natural selection requires a genetically diverse population from which to select being able to shuffle their genetic material allows organisms to adapt to their environments more rapidly (from a population standpoint of course) Shuffling the genetic deck however, nature has another trick up its sleeve for mixing things up genetically; it actively recombines genes in a process called general recombination. recombination produces novel combinations of alleles that might be useful for an organism in a particular environment. Module 4 42 this dramatically enhances the genetic diversity of gametes and accelerates adaptation through natural selection Recombination Revisiting Morgan in order to understand recombination, we need to travel back to Thomas Hunt Morgan’s fly room at Columbia University. the basic principles of recombination were worked out in a series of classical experiments from Morgan’s lab Genetic linkage and recombination Morgan worked with flies that differed at two characters: body color and wing size. wild type flies have gray bodies and normal sized wings, whereas mutant flies had black bodies and vestigial wings (both mutant alleles were recessive). importantly, these genes were located on the same chromosome and were therefore inherited together as a unit—this is genetic linkage. Module 4 43 Anomalous non-parental phenotypes Morgan crossed his flies to produce a dihybrid F1 generation. when these F1 flies were test crossed with mutant flies, the majority of offspring showed the parental phenotype (remember that these two genes are inherited as a unit because they’re on the same chromosome). however, some flies showed a non-parental phenotype, which was not consistent with the genetic linkage, and suggests that the genes had been recombined in some way. Follow the chromosomes I Module 4 44 we can understand this better by following the chromosomes. the F1 dihybrid can make either b+vg+ or b vg gametes, whereas the mutant fly can only make b vg gametes. the only way to explain non-parental phenotypes is to assume that parts of the chromosome have been exchanged Follow the chromosomes II if we consider this genetic exchange, now the F1 dihybrid fly can make four possible gametes. Module 4 45 the majority of the offspring have parental phenotypes, but the recombinant offspring have non-parental phenotypes. A closer look a recombination recombination occurs through a process called crossing over, which occurs during prophase I of meiosis. during prophase I, the homologous chromosomes pair up to form a bivalent. complementary regions of each chromosome are exchanged between the maternal and paternal homologs through synapsis. this generates offspring with new combinations of the parental genes. The synaptonemal complex Module 4 46 crossing over occurs while DNA is held in a special structure called the synaptonemal complex. the two DNA strands are bound to a ladder-like protein structure called the central element and two lateral elements. in the center of the structure sits a protein complex called the recombination module. Stages of prophase I prophase I is itself divided into five stages: leptotene, zygotene, pachytene, diplotene, and diakinesis. the events of each stage are organized according to the assembly and disassembly of the synaptonemal complex. synapsis occurs during pachytene when the synaptonemal complex is functional. Chiasmata once the crossing over is complete, and the synaptonemal complex breaks down, homologs are held together by chiasmata. chiasmata are simply the remnants of the cross over event, but are important for physically holding homologous chromosomes together until anaphase I of meiosis. each bivalent has at least one chiasma by the end of prophase I, although many have two or three. Chromosomal Disorders Module 4 47 meiosis gone wrong occasionally, mistakes in mitosis or meiosis lead to cells with an inappropriate number of chromosomes; a condition called aneuploidy. if only a single copy of a particular chromosome is present, this is called monosomy, and if three copies of a particular chromosome are present, this is called trisomy. when an aneuploid cell arises, this genetic status is passed on to all subsequent cells through mitosis nondisjunction aneuploidy most commonly occurs due to a nondisjunction event during meiosis. here, the chromosomes fail to separate during anaphase and the resulting gametes receive either an extra chromosome (n+1) or lack a chromosome (n-1). nondisjunction can occur during either anaphase I or anaphase II. Nondisjunction of the sex chromosomes nondisjunction of the sex chromosomes is quite common and the aneuploid offspring are viable. Module 4 48 there are several viable outcomes for aneuploidy of the sex chromosomes: an XXX female, an XO female, and XXY male, and an XYY male. XXX females and XYY males do not have any physiological abnormalities and are fertile, but OY males are not viable. Klinefelter syndrome an XXY male has a condition known as Klinefelter syndrome, which occurs in 1 / 500 to 1000 live male births. boys with Klinefelter syndrome appear normal until puberty, but have undersized male genitalia and are infertile. they also tend to be taller than average, have narrow shoulders, develop small breasts, and have mild cognitive deficits. Turner syndrome Module 4 49 an XO female has a condition known as Turner syndrome, which occurs in 1 / 2500 live female births. Turners syndrome is the only viable monosomy in humans. women with Turner syndrome are short in stature, have a low hairline, webbed neck, low-set ears, and underdeveloped reproductive organs— their intelligence is usually normal. Down Syndrome: trisomy 21 humans with three copies of chromosome 21 have a condition known as Down syndrome, which affects nearly 1 in 700 children. this syndrome is characterized by short stature, facial abnormalities, heart defects, cognitive impairment, and have an increased risk of leukemia and Alzheimer's disease. large-scale chromosomal changes Module 4 50 pieces of chromosomes can get moved around quite a bit due to strand breaks and reattachments, leading to deletions, duplications, and inversions. one particularly important type of chromosomal abnormality is a translocation, where segments of non-homologous chromosomes are reciprocally exchanged. nonreciprocal translocations are less common but do occur. Robertsonian Translocation Module 4 51 Down Syndrome can also be caused by translocations, such as the Robertsonian translocation. here, the short arm of an acrocentric chromosome is exchanged for another, creating a large metacentric chromosome. this type of translocation occurs between chromosomes 15 and 21, causing affected individuals to have extra copies of a large part of chromosome 21. the Philadelphia chromosome a reciprocal translocation during mitosis between chromosomes 9 and 22 in cells that produce white blood cells can lead to chronic myelogenous leukemia (CML). this translocation fuses two genes that usually control the cell cycle (BCR and Abl1) into a single gene and the resulting hybrid protein leads to unregulated growth. this shortened form of chromosome 22 is called the Philadelphia chromosome Ploidy changes in cancer: HeLa cells Module 4 52 HeLa cells were originally cultured in 1951 from a young woman who was dying of cervical cancer named Henrietta Lacks. when cultured, these cells were found to be immortal, that is, they could divide over and over again due to the ectopic expression of telomerase. the number of chromosomes in HeLa cells varies, but there are usually > 80! Module 4 53