CHM 230 Introduction to Analytical Chemistry PDF
Document Details
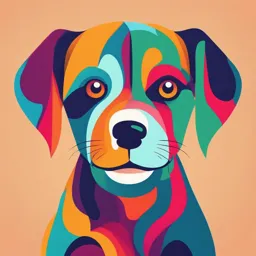
Uploaded by WellEducatedEmpowerment2924
Benue State University
Tags
Summary
This document provides lecture notes on analytical chemistry, covering topics such as separation methods (chromatography, solvent extraction, ion-exchange), analytical methods (gravimetric, electrochemical, optical, instrumental), and specific techniques like filtration and distillation.
Full Transcript
BENUE STATE UNIVERSITY DEPARTMENT OF CHEMISTRY CHM 230-INTRODUCTION TO ANALYTICAL CHEMISTRY (CREDIT UNIT -3) Oklo LECTURE NOTES Separation methods: Chromatography (Tin Layer, Paper and Column), Solvent extraction, ion- ex...
BENUE STATE UNIVERSITY DEPARTMENT OF CHEMISTRY CHM 230-INTRODUCTION TO ANALYTICAL CHEMISTRY (CREDIT UNIT -3) Oklo LECTURE NOTES Separation methods: Chromatography (Tin Layer, Paper and Column), Solvent extraction, ion- exchange: Electrphoresis and dialysis; Colorimetry and radio chemical methods (e.g. Isotopic dilution methods) ANALYTICAL METHODS Water analyses are done by several methods. The most common types of measurements are gravimetric (weighing), electrochemical (using meters with electrodes) and optical (including visual). Instrumental methods are becoming increasingly popular, and instrumentation is getting "smarter" and easier to use with the inclusion of microprocessors. In the simplest case, a sample may just be placed in an instrument and a result read directly on a display. More often some physical separation technique or chemical procedure is needed before a measurement is made, in order to remove interferences and transform the analyte- the target of the analysis- into a form which can be detected by the instrument. Since even raw sewage is generally more than 99.9% water, most environmental analyses are measuring very low concentrations of materials. The results of these measurements are usually expressed in the units "milligrams per liter," abbreviated as mg/L. Since a milligram is one thousandth of a gram, and a liter of water weighs about a thousand grams, a mg/L is approximately equal to one part per million by weight. A part per million ("ppm") is only one ten thousandth of one percent. For toxic metals and organic compounds of industrial origin, measurements are now routinely made in the part per billion (microgram per liter) range or even lower. At such low levels, sensitive equipment and careful technique are clearly necessary for accurate results. Avoiding contamination of the sample and using methods which prevent interferences from other substances in the water are crucial requirements for successful analyses. Separation Techniques Some measurements require separating the analyte from other substances in the water which may interfere with the measurement. Some measurements even require separating the analyte from the water entirely. Separation techniques include: Filtration: The water is passed through a fine-pore filter which can be made of paper, glass fibers, a cellulose acetate membrane, etc. Filtration through a filter of some agreed-upon standard pore size can be used to separate "suspended" from "dissolved" portions of the analyte. The analyte may be the suspended matter which is captured on the filter-- or the filter may be used to clarify the water for analysis of a dissolved material. Often, the filtration is assisted by applying a vacuum below the filter, which is supported on a porous holder in some type of funnel. Distillation: If the analyte can be boiled out of the water, or along with the water, then the vapors can be cooled and re-condensed or trapped in a liquid form in a different container. This way the analyte can be removed from the interfering substances in the original water sample. Often the sample is made acidic or alkaline, or treated chemically in some other way before distillation, to convert the analyte into a volatile (easily evaporated) form, and to immobilize or neutralize interfering substances. Extraction: Some analytes may be much more soluble in an organic solvent than in water. If the solvent does not mix with water, and the sample is shaken with portions of the solvent, almost all of the analyte may be transferred from the water into the solvent, leaving interfering substances behind. This is known as a "liquid-liquid" extraction. The analysis may be completed using the organic portion. There are also continuous versions of this process for use with liquid or with dry samples. Another type of extraction is called "solid-phase extraction." In this kind of procedure, the sample is passed through a column or filter containing a powdered or granulated material which retains (adsorbs) the substances of interest and allows other types of dissolved materials to pass through. Then a solvent, or an acid or alkaline solution, can be passed through to de-sorb and redissolve the analytes, a process known as elution. Either type of extraction can also be used to concentrate the analyte into a smaller total volume, which increases the sensitivity of the analysis. This can be true for distillation or filtration, as well. Chromatography This technique got its name, which means "color picture", because it was first used to separate colored pigments from a single spot on a piece of paper. A solvent, such as alcohol, is allowed to move slowly across the paper, and the different components of the pigment travel at different rates. The result is a series of separated spots of different colors. They move at different rates because of differences in the pigments' relative attraction to the paper (the "stationary phase") and their solubility in the solvent (the "mobile phase"). This principle is used in modern instrumentation to separate mixtures of organic chemicals or inorganic ions. The components can be identified by their retention times (how long it takes them to pass through the instrument) and detectors can be used to measure the amount of each component. In gas chromatography, (or, simply, "GC") the mixture of substances is injected into a narrow, coiled column, several feet long, made of an inert material like glass, silica or stainless steel. The sample has usually been extracted into an organic solvent and concentrated by evaporation as a pretreatment step. The column may be filled with an oil-coated, powdered mineral, which forms the stationary phase. In the narrower capillary columns, the stationary phase is bonded directly to the wall of the tubing. The columns are usually contained in an oven, which may be programmable to raise the temperature at a controlled rate over time. Heating the column allows analysts to use this technique on many substances which are not gases at room temperature, including solvents and toxic chemicals like pesticides and PCB's. A continuous flow of an inert gas, such as argon, helium, or sometimes nitrogen, carries the evaporated mixture through the column. The substances are detected as they exit the column, usually by a technique that converts them into ions (electrically charged atoms or molecules), although one method uses heat conduction. The ions are produced by means such as flames, ultraviolet light, or radioactive materials. They are detected by being attracted to charged plates, where they produce an electrical current proportional to the amount present. The output of the detector usually is shown as a chart of "peaks" vs. time, called a chromatogram, often with the retention time and the intensity of the peak printed out. The retention time is used to identify the substance, while the height or area of the peak is used to quantify its concentration. A more positive identification is possible using a mass spectrometer as the detector. For substances which cannot easily be vaporized because of high boiling point or instability at higher temperatures, there is a liquid version of this technique known as HPLC (High pressure or high performance liquid chromatography). Organic solvents are used as the mobile phase. Ultraviolet (UV) light absorption is often used for detection. Herbicides and pharmaceuticals are common types of substances analyzed by this technique. Another variation of LC is ion chromatography, (IC), where the target analytes are charged inorganic or organic substances. The mobile phase is an aqueous (water-based) solution, and the stationary phase is made up of an ion exchange resin. The detectors usually measure electrical conductivity, although UV absorption can also be used. This technique can be used to measure the concentrations of several important inorganic anions, such as fluoride, sulfate, phosphate, and nitrate all in one analysis ▪ Introduction ▪ Principle ▪ Classifications ▪ Applications Introduction Chroma– “color” and graphein – “to write”. Chromatography is a technique in which substance are separated, purified and identified from a mixture for qualitative and quantitative analysis. On the basis of hydrophobic interactions, Polarity, enzymes and net charges are separated by using chromatography. Chromatography is a physical method of separation of compound. TSWET, referred as the father of chromatography. This technique was first used by Tswet, to separate various plants pigments such as chlorophylls and xanthophylls by passing these pigments through glass column packed with finely divided calcium carbonate. Purpose of Chromatography Analytical: To determine chemical composition of a sample. Preparative: Used in purification of a substance. Principle of chromatography The principle of chromatography is very simple. The sample to be examine or analyzing is termed as solute or analyte is allowed to interact or react with two immiscible phases- I. Mobile phase II. Stationary phase Image source khan academy The stationary phase which may be a solid or a liquid supported on a solid, which is immobile it means it does not move. The mobile phase migrates the sample through stationary phase. The mobile phase may be either liquid or gas. All chromatographic methods involve separation of mobile phase through a stationary phase. Classification of Chromatography Chromatography technique can be explained into three fundamental ways: I. Based on the shape of chromatographic bed. II. Based on the physical nature of the stationary and mobile phases. III. Based on the mechanism of the separation. Based on the shape of chromatographic bed: On the basis of the chromatographic bed, there are following two types of chromatography- I. Planar chromatography. II. Column chromatography. In planar chromatography, the stationary phase is spread on a flat, planar surfaces. The plane can be paper acting as a stationary phase (paper chromatography), or stationary phase spread on glass, metal or plastic plate (thin layer chromatography). Planar chromatography also known as open-bed chromatography. In column chromatography, the stationary phase is within a tube. Image Source Khan Academy Based on the physical nature of the mobile and stationary phase: On the basis of physical nature of mobile phase, there are two types of chromatography: I. Gas chromatography II. Liquid chromatography Further, on the basis of stationary phase, physical nature there are the following types: I. Gas-solid chromatography II. Gas-liquid chromatography III. Liquid-solid chromatography IV. Liquid-liquid chromatography Based on the mechanism of separation: The method of chromatography uses various types of mechanisms to separate analyte. On the basis of mechanism of separation, it is of following types: I. Partition chromatography II. Adsorption chromatography III. Size exclusion chromatography IV. Affinity chromatography V. Ion exchange chromatography Applications In the pharmaceutical sector: In detection of presence of trace elements or chemicals. This is also used for the separation of compound on the basis molecular weight and composition of elements. In the detection of unknown compound and in the purity of mixtures. In the development of drug. In the Chemical industry: HPLC and GC are very much used for detecting various contaminants such as polychlorinated biphenyl (PCBs) in pesticides and oils. In the Food Industry: In the detection of food spoilage and additives. In the quantitative and qualitative analysis of nutrients in food. In Forensic Science: In forensic science, this technique is used in the analysis of crime scenes like hair and blood samples. In Molecular Biology Studies: Various techniques in chromatography such as EC-LC-MS are involved in the study of metabolomics and proteomics along with nucleic acid research. HPLC is used in Protein Separation like Insulin Purification, Plasma Fractionation, and Enzyme Purification and also used in the various industries like Fuel Industry, biochemical processes and biotechnology. Chromatography like HPLC is used in DNA fingerprinting and bioinformatics: Assay & Content Uniformity. HPLC in fingerprinting and Bioinformatics. Petrochemicals and Catalysis. Ebola Immunization. Polymer Synthesis. Clinical diagnosis of diseases and disorder. LC-NMR (liquid chromatography (LC) and nuclear magnetic resonance). DIALYSIS It is one of the most common laboratory techniques that is used to remove molecules from solutions. This principle is also used to artificially purify blood in case of kidney dysfunction. This article delves into what dialysis is, the procedure and its importance. Dialysis - Chemical Separation To define the scientific term, ‘dialysis’ is a process of separating molecules in particular solutions with the help of a membrane that is semipermeable known as a dialysis tube. It is a separation of dissolved ions and molecules from solid solutions. The separation of molecules takes place because there is a difference in the rate of diffusion of certain molecules in the solution. The application of dialysis is used for the elimination of small and unwanted molecules such as salt, and large unwanted molecules such as dyes in particular solutions. It is also used in the studies of drugs and electrophoresis. History of Dialysis The chemical procedure of dialysis was discovered and introduced by a Scottish chemist namely Thomas Graham in the year 1868. Graham used this technique in the separation of small molecules and large molecules in an aqueous solution. The name that he has given to those diffusible substances as crystalloids and those that would get stuck in the membrane or dialysis tube is called colloids. This process of separation is also called Graham’s Law in chemistry. This chemical separation is an extremely rapid and spontaneous process of separating with the help of the semipermeable membrane, and the most types of membranes used in the process of dialysis are cellulose and synthetic polymer. The Procedure of Dialysis Separation To separate molecules, dialysis is one of the most straightforward and fast-paced chemical procedures. There is particular equipment that is required for the process of dialysis such as: 1. A container to keep the molecules. 2. A dialysis tube 3. Control of temperature The Steps Involved in the Procedure of Dialysis are as Follow: Preparation of membrane according to the instructions. Loading the molecules into a tube or any instrument. Placing the sample solution into the dialysis tube Dialyzation of the sample at room temperature for around 2-3 hours. Changing of buffer and again placing the sample for the process of dialyzation for approx 2 hours. Importance of Dialysis It is used for two reasons (i) for introducing new molecules in a sample solution or (ii) to remove small molecules from a sample solution because it flows smoothly in any direction of the membrane. These two reasons make dialysis an extremely important chemical procedure for various applications and scientists prefer this procedure as well. It is also one of the chemical procedures to change the size and matrix of molecules in particular samples with the help of differentiating the sizes of molecules present inside the solution. During the process of dialysis, equilibrium is obtained in between the solution and celluloid as only small molecules can pass through the membrane or dialysis tube. Dialysis chemical separation is also used in the procedure of eliminating salt. Osmosis helps in the smooth functioning of dialysis as the fluid moves from high to low water concentration which makes it easy to pass through the semipermeable membrane or the dialysis tube. Ultrafiltration also helps in dialysis as it easily removes the excess fluid and small molecules in the sample solution which makes the procedure of dialysis extremely easy and fast to perform. Types of Dialysis (Chemical Separation) 1. Diffusion Dialysis The spontaneous and rapid type of dialysis chemical separation process. It is preferred by many scientists. Diffusion Dialysis uses AEM (Anion exchange membranes) and CEM (cation exchange membrane) which is dependent on the compounds and molecules that are to be separated. 2. Electrodialysis It uses the electrical potential for the procedure of dialysis chemical separation and uses the ion- membrane as a driving force. Electrodialysis is extremely important when it comes to removing molecules from aqueous solutions. Three other kinds of electrodialysis are commonly and widely used by scientists in laboratories worldwide. They are as follow: Reverse Dialysis Electro-electrodialysis Donnan dialysis Uses of Dialysis (Chemical Separation) The Application of this Chemical Separation are as Follows: Desalting soy sauces. The dealcoholization of beer Alkali waste Aqueous solutions Acid Caustic soda solution De-acidification of fruits and vegetables Food industry for desalination Amino acids. Neutral and basic element groups. Fact about Dialysis Chemical Separation Here is the List of Facts about the Dialysis Chemical Separation. They are as Follow: Low consumption of heat Operates under room temperature No operating, installing, and other costs. Flexible and stable Good for the environment as it doesn’t pollute it. Dialysis separation is a slow procedure that is slowed by differences in particle size and diffusion rates between colloidal and crystalloid materials. But it is one of the most appreciable methods when it comes to change of solvent, analytes and chemical buffers without disrupting the nature of molecules due to any sudden change of chemical environment and hence in that regard the most beneficial. ELECTROPHORESIS: Electrophoresis is the migration and separation of charged particles (ions) under the influence of an electric field. It is the movement of charged particles through an electrolyte when subjected to an electric field at given pH. Electrophoresis is a simple and sensitive separation technique in clinical and research laboratories. Since its discovery, it has been an essential tool used by biologists and chemists to separate mixtures, especially proteins and nucleic acids. Electrophoresis consists of two words; electro, meaning electricity, and phoresis, meaning movement. Thus, it implies the migration and separation of a charged particle (ions) through a solution under the influence of an electric field. It was first demonstrated in 1807 by Ruess, who noted the migration of particles towards the anode. It has improved from the initial crude paper electrophoresis to the modern automated system. Various improved versions are available, which apply in miniaturization, precision engineering, etc. The benefit of advancement is meeting the requirement for faster and better resolution of results. Electrophoresis can be defined as an electrokinetic process that separates charged particles in a fluid using an electrical field of charge. Electrophoresis of cations or positively charged ions is sometimes referred to as cataphoresis (or cataphoretic electrophoresis). In contrast, sometimes, the electrophoresis of anions or negatively charged ions is referred to as anaphoresis (or anaphoric electrophoresis). Anaphoresis - Electrophoresis of anions or negatively charged particles. Cataphoresis - Electrophoresis of cations or positively charged particles. Iontophoresis – Migration of small ions. Zone Electrophoresis - migration of charged macromolecules in porous Support medium such as paper, cellulose acetate or agarose gel film It’s used in a variety of applications. Though it is most often used in life sciences to separate protein molecules or DNA, it can be achieved through several different techniques and methods depending upon the type and size of the molecules. The methods differ in some ways, but all we need is a source for the electrical charge, a support medium and a buffer solution. Electrophoresis is also used in laboratories for the separation of molecules based on their size, density and purity. The method used to separate macromolecules such as DNA, RNA, or protein molecules is known as gel electrophoresis. It is used in forensics for – Detection and analysis of variations or mutations in the sequence Its clinical applications involve – Serum protein electrophoresis Lipoprotein analysis Diagnosis of haemoglobinopathies and hemoglobin Determination of serum protein Phenotypes and micro heterogenesis Protein genotyping – e.g., ApoE analysis for Alzheimer’s disease (polymorphic protein) Monitoring of Small molecules – e.g., drugs and steroids Cerebrospinal fluid analysis Urine analysis Principle of electrophoresis The fundamental principle of electrophoresis is the existence of charge separation between the surface of a particle and the fluid immediately surrounding it. An applied electric field acts on the resulting charge density, causing the particle to migrate and the fluid around the particle to flow. It is the process of separation or purification of protein molecules, DNA, or RNA that differ in charge, size, and conformation. The charged molecules are placed at one end of the field according to their charge, and an electric field is applied. On passing electric current, depending upon the kind of charge the molecules carry, they move towards the opposite electrodes – either cathode (negative electrode) or anode (positive electrode). The size, shape, and charge of the molecule remains constant during electrophoresis and determines ionic particle mobility. Biological molecules, like amino acids, peptides, proteins, nucleic acids, and nucleotides, possess ionizable groups. These molecules exist in solution as electrically charged species, cations (+), or anions (-) at any given pH. Thus, the electric field allows the migration of the negatively charged molecule towards the anode (a positive terminal). In contrast, the positively charged molecule migrates towards the cathode (a negative terminal). The separation of the molecules, ions, or colloidal particles suspended in the matrix occurs due to the force of an electric field. The molecules move through a sieve-like compound based on the molecular mass and charge ratio. It is an incomplete form of electrolysis as the electric field is removed before the molecules reach the electrode, yet the molecules separate due to electrophoretic mobilities. Nucleic acids have negative phosphate backbones. Hence they move towards the anode in DNA electrophoresis. Ampholytes, like proteins, bear both positive and negative charges. Such compounds have negative charge in normal conditions and migrate towards the anode. At the same time, they are positively charged in acidic conditions and move towards the cathode. Hence, protein bears a negative or positive charge depending on solvent pH and isoelectric point. An ampholyte acts as a positively charged particle or ion migrating towards the cathode in acidic conditions. In contrast, it acts as a negatively charged particle or ion in an alkaline condition and moves towards the anode. Biological molecules, like amino acids, peptides, proteins, nucleic acids, and nucleotides, possess ionizable groups. These molecules exist in solution as electrically charged species, cations (+), or anions (-) at any given pH. Thus, the electric field allows the migration of the negatively charged molecule towards the anode (a positive terminal). In contrast, the positively charged molecule migrates towards the cathode (a negative terminal). The separation of the molecules, ions, or colloidal particles suspended in the matrix occurs due to the force of an electric field. The molecules move through a sieve-like compound based on the molecular mass and charge ratio. It is an incomplete form of electrolysis as the electric field is removed before the molecules reach the electrode, yet the molecules separate due to electrophoretic mobilities. Nucleic acids have negative phosphate backbones. Hence they move towards the anode in DNA electrophoresis. Ampholytes, like proteins, bear both positive and negative charges. Such compounds have negative charge in normal conditions and migrate towards the anode. At the same time, they are positively charged in acidic conditions and move towards the cathode. Hence, protein bears a negative or positive charge depending on solvent pH and isoelectric point. The rate of migration of an ion in a supporting medium under the influence of an electric field depends upon the following factors – The net charge of the molecule The size and shape of the molecule The strength of the electric field The properties of the supporting medium The temperature of the procedure Factors Affecting the Rate of Ion Mobility Inherent factors The velocity of ions depends on both inherent factors and the external environment. The inherent factors that affect the velocity of ions are: -Charge density -Molecular weight -The net charge of the molecule -Size and shape of the molecule i.e., whether it’s tertiary or quaternary structured External factors The external factors affecting the rate of movement of ions are: -Electrical parameters, like current, voltage, and power -Viscosity and pore size of supporting medium -Temperature of solution -The pH of the buffer Electrophoresis Instrument Modern electrophoresis equipment and systems vary based on its types and forms. However, all the electrophoretic system possesses two essential components: 1. Power pack Power supply drives the movement of ionic species in the medium and allows adjustment and control of either the current or the voltage. 2. An electrophoresis unit An electrophoretic system depends on its type but essentially consists of two electrodes of opposite charge (anode and cathode), connected by a conducting medium called an electrolyte. In addition, a supportive medium is present in electrophoretic systems like gel and paper electrophoresis. Buffer (Electrolyte) Buffers carry applied electric current and provide appropriate pH for the process. Conducting (running) buffers like Tris borate EDTA (TBE) and Tris-acetate acid EDTA (TAE) are commonly used. Supportive Medium The supportive medium is the matrix (gel), in which biomolecules are separated. It can be in the slab or capillary form. The supportive mediums used are sugar polymers like agarose gel, polyacrylamide gel, starch gel, and cellulose acetate gel. The medium runs either vertical or horizontal gel systems in gel electrophoresis. Horizontal: agarose gel electrophoresis, and vertical: SDS-PAGE. The higher the pore size, the higher the speed traveled by charged particles. General Procedure of Electrophoresis The electrophoresis process has three main steps; separation, detection, and quantification. Separation The instrument set up is according to its type. In the gel electrophoresis, gels are prepared and cast. Then placed into the electrophoresis chamber. The supportive medium can be agarose gels or polyacrylamide gels. Then appropriate buffer solution is added to the system. After the proper setup of the instrument, the sample is placed into the medium. Then the sample is run at a specific current, voltage, or power. Detection and Quantification Staining with a dye or autoradiography (for radioactive samples) helps in the detection of the separated components. Quantification is done using a densitometer or by direct measurement using an optical detection system. For example, protein is fixed by precipitating in gel with acetic acid. Methanol helps prevent the diffusion of proteins from the gel during the staining process. Forms of Electrophoresis Based on the forms, it is of two types; zone and moving boundary electrophoresis. In zone electrophoresis, molecules fixed to particular zones do not interact with the surroundings. Paper, cellulose acetate strips, and gel electrophoresis are examples of it. In the moving boundary electrophoresis, charged molecules migrate in a free-moving solution without a supporting medium. E.g., Capillary electrophoresis. Types of Electrophoresis There are mainly two types of electrophoresis: Zone electrophoresis and moving boundary electrophoresis. These two are further classified into – Zone electrophoresis : Paper electrophoresis Gel electrophoresis Thin layer electrophoresis Cellulose acetate electrophoresis Moving boundary electrophoresis : Capillary electrophoresis Isotachophoresis Isoelectric Focusing Immuno-electrophoresis Based on the nature of the supporting medium, it is of the following types: Agarose gel electrophoresis Polyacrylamide gel electrophoresis Cellulose Acetate Electrophoresis Capillary Electrophoresis Depending on the mode of technique, it has the following types: Paper electrophoresis Isoelectric focusing electrophoresis Two-dimensional Polyacrylamide gel electrophoresis Pulse field gel electrophoresis Zymography Immunoelectrophoresis Capillary electrophoresis High voltage electrophoresis Isotachophoresis Microchip electrophoresis Capillary Electrophoresis: Principle and Application Electrophoresis is a method of separation where the movement of ions under the influence of electricity helps separate components. There are various types of electrophoresis. Gel electrophoresis, cellular acetate electrophoresis, and capillary electrophoresis are some of the commonly used types of electrophoresis. Capillary electrophoresis is a liquid-based separation technique that uses a capillary as a separation channel under the influence of an electric field. It is an analytical technique where the electroosmotic flow helps separately charged ions. It is applicable in various areas like analysis of chemical substances, DNA analysis, identifying specific proteins, and separating coenzymes. Principle of Capillary Electrophoresis The velocity of the analyte when migrating under the influence of an electric field of intensity “E” is given by the analyte’s electrophoretic mobility and the buffer’s electroosmotic mobility inside the capillary. The electrophoretic mobility of solute depends on the different characteristics of the solute, like electric charge, molecular size, and shapes, and the properties of the buffer, like electrolyte’s ionic strength, pH, viscosity, and additives. The following equation gives the electrophoretic velocity (Vep) of the solute: Vep= μepE = (q/6πηr) (V/L) Where: η is the viscosity of the electrolyte solution, V is the applied voltage, L= the length of the capillary, r is the Stoke’s radius of the solute, μep is the electrophoretic mobility of the solute, and q is the effective charge of the solute. When applied through the capillary filled with the buffer, the electric field generates a solvent flow called the electroosmotic flow inside the capillary. The velocity of which depends on the electrophoretic mobility. Electrophoretic mobility depends on the charge density of the capillary’s internal wall and the buffer’s properties. The following equation gives the electroosmotic velocity (Veo): Veo = μeoE = (ε𝜁/η) (V/L) where ε= buffer’s dielectric constant, 𝜁= zeta potential of the capillary surface, η is the viscosity of the electrolyte solution, V is the applied voltage, μeo is the electrophoretic mobility, and L is the length of the capillary. The solute’s velocity (V) is given by: V= Vep+Veo. The electroosmotic and electrophoretic mobility of the analyte can act in the same or opposite direction based on the solute’s charge. In the case of normal capillary electrophoresis, anions will move in the opposite direction of electroosmotic flow with velocities smaller than the electroosmotic velocity. In contrast, the cations will migrate in the same direction of the electroosmotic flow with velocities higher than the electroosmotic velocity. In conditions with a fast electroosmotic rate compared to the electrophoretic rate of the solutes, the separation of both cations and anions occurs in the same run. The time is taken (t) for the solute to migrate to the detection point from the injection end of the capillary (effective capillary length) is given by: Generally, uncoated fused silica capillaries above pH three that have a negative charge are used where the electroosmotic flow occurs from the anode to the cathode. The electroosmotic flow must be constant for each run for good reproducibility in the migration velocity of the solutes. For some experiments, reduction or suppression of electroosmotic glow by changing the inner wall of the capillary, the concentration, composition, or pH of the buffer solution might be necessary. After adding the sample, an independent zone of each analyte ion of the sample forms. The zone formation is due to the migration within the background electrolyte. The spreading of each solute band occurs as a result of different phenomena. Under ideal conditions, the only phenomenon contributing to the zone’s broadening is molecular diffusion of the solute inside the capillary. Here, the efficiency of the zone is given as the number of theoretical plates (N), which is given by: N= (μep+μeo) (Vl)/ 2DL where D is the molecular diffusion coefficient of the solute buffer. In general practice, other phenomena like the length of the injection plug, detector cell size, unleveled buffer reservoirs, mismatched conductivity between sample and buffer, sample adsorption onto the capillary wall, and heat dissipation, play a significant role in band dispersion. The separation between two bands is gained by modifying the analytes’ electrophoretic mobility, the electroosmotic mobility induced in the capillary, and increasing each analyte’s efficiency for the band as follows; Rs= N(μepb-μepa)/ 4(μaep+μeo) where μepa and μepb= the two analytes’ electrophoretic mobilities, μaep is the average electrophoretic mobility of the two analytes calculated by: μaep= ½ ( μepb+μepa) During a separation, uncharged molecules move at the same velocity as the electroosmotic flow (with very little separation). Positively – charged ions move faster and negatively – charged ions move slower. Fig. mode of capillary zone electrophoresis showing normal migration with electroosmotic flow toward the cathode Capillary Tubes Fig. shows a cross-section of a typical capillary tube. Most capillary tubes are made from fused silica coated with a 15–35 μm layer of polyimide to give it mechanical strength. The inner diameter is typically 25–75 μm, which is smaller than the internal diameter of a capillary GC column, with an outer diameter of 200–375 μm. Fig. Cross section of a capillary column for capillary electrophoresis. The dimensions shown here are typical and are scaled proportionally in this figure. Injecting the Sample There are two common methods for injecting a sample into a capillary electrophoresis column: hydrodynamic injection and electrokinetic injection. In both methods the capillary tube is filled with the buffer solution. One end of the capillary tube is placed in the destination reservoir and the other end is placed in the sample vial. Hydrodynamic injection uses pressure to force a small portion of sample into the capillary tubing. A difference in pressure is applied across the capillary either by pressurizing the sample vial or by applying a vacuum to the destination reservoir. The volume of sample injected, in liters, is given by the following equation Instruments Used in Capillary Electrophoresis 1. Power supply: Capillary electrophoresis requires a high voltage controllable direct current power supply. 2. Buffer reservoirs: Capillary electrophoresis requires two buffer reservoirs held at the same level that contain specified anodic and cathodic solutions. 3. Electrodes: CE needs two electrodes (cathode and anode) immersed in the buffer reservoirs connected to the power supply. 4. Capillary: A capillary made up of fused silica, usually with a diameter of fewer than 100 microns. 5. Viewing window: CE requires an optical viewing window that is aligned to the detector. 6. Injection system: CE requires a suitable injection system for adding sample and buffer into the capillary. The method of injection can be automated for precision. The common mode of injecting samples is gravity, pressure or vacuum, or electrokinetic. 7. Detector: CE requires a detector for monitoring the amount of substance that passes through the capillary at a given time based on conductimetric, fluorimetry, absorption spectrophotometry (UV and visible), amperometric, or mass spectrometric detection. 8. Thermostatic system: CE requires a thermostatic system for maintaining a constant temperature inside the capillary. 9. Recorder: CE requires a recorder that records the data after the completion of electrophoresis. 10. Suitable integrator or computer: CE also needs the right integrator or computer to convert data digitally. Types of Capillary Electrophoresis Method There are six types of capillary electrophoresis methods commonly used; CZE (capillary zone electrophoresis), CGE (capillary gel electrophoresis), Micellar electro kinetic capillary chromatography (MEKC), capillary electrochromatography (CEC), capillary isoelectric focusing (CIEF), and capillary isotachophoresis (CITP). Application of Capillary Electrophoresis Capillary electrophoresis is commonly applied in various fields. Analyzing food contents, genetic differentiation, and clinical diagnosis are typical applications of capillary electrophoresis. The following is a detailed explanation of the area of applications of capillary electrophoresis: 1. Food technology: Capillary electrophoresis is applied to analyze different food, like beverages and fermented food, to detect flavonoids, vitamins, carbohydrates, proteins, pigments, and color. CE is also applicable for detecting DNA and RNA from bacteria and viruses, which indicates contamination. 2. Molecular analysis: The DNA, RNA, and amino acids are detected using capillary electrophoresis, especially in the microbiology laboratory and forensic science. 3. Disease diagnosis: Lipid profile analysis using CITP is critical for detecting cholesterol levels in clinical laboratories. Likewise, the human serum’s analysis of vitamins and minerals is performed using capillary electrophoresis. 4. Environmental monitoring: Capillary electrophoresis has been applied to track inorganic ions in rivers and water. 5. Likewise, nano-capillary electrophoresis is applied to detect environmental pollutants at the nano level. 6. Pharmaceutical laboratories: Capillary electrophoresis is used in the analysis of small molecules in drugs during preparatory stages and standard solutions. Advantages of Capillary Electrophoresis Capillary electrophoresis has many advantages over other methods of electrophoresis and chromatography due to the use of tiny capillaries for separating. The following is a detailed explanation of the benefits of capillary electrophoresis: 1. Highly efficient: Heat dissipation occurs efficiently due to using a small diameter of the capillary. Some articles claim the efficiency of capillary electrophoresis is quite similar to that of liquid chromatography. (7) 2. Less time-consuming: If the person is familiar with computer software, it takes no time to read the result of separation. Likewise, the entire process takes only one hour, from setup to separation. So, capillary electrophoresis carries more advantages as compared to other separation techniques. 3. Wide area of application: Capillary electrophoresis is used in analyzing macromolecules like proteins, DNA, different drugs, and RNA. So, CE is applied widely from pharmaceutical to environmental monitoring. 4. Has the possibility of automation: The injection method, data analysis, buffer, and sample uploading steps can be automated in capillary electrophoresis. Disadvantages of Capillary Electrophoresis Although capillary electrophoresis is highly advantageous, it has some disadvantages, which are as follows: Changing capillaries is challenging: Due to the size of the capillaries, removing and replacing capillaries can be challenging at times. So, expertise is required for changing capillaries to experiment. Less robust: Small changes like the size of capillaries and choice of analytes can be crucial in capillary electrophoresis. So CE is less robust than other methods. Classification of electrophoresis It is classified into the following: Slab gel electrophoresis – A traditional method that uses a rectangular gel regardless of its thickness. Disc electrophoresis – Discontinuities are caused in the electrophoretic matrix because of layers of polyacrylamide or starch gel that differs in composition & pore size. Isoelectric focusing electrophoresis – IEF separates atmospheric compounds (like proteins) with increased resolution in a medium possessing a stable pH gradient. Isotachophoresis – It completely separates smaller ionic substances into adjacent zones that contact one another without overlapping & all of them have the same rate of migration. Pulse-Field electrophoresis – Power is alternatively applied to different pairs of electrodes or electric arrays such that the electrophoretic field is cycled between two directions. 2D electrophoresis is a charge-dependent IEP in the first dimension, whereas it is molecular weight dependent in the second dimension. Uses of Electrophoresis It is applied for routine laboratory experiments, disease diagnosis, research-oriented separations and identification. Similarly, it is used in various other fields, like forensics, agriculture, pharmaceutical, foods, etc. Some of its applications are described below: DNA Analysis and DNA Fragmentation Gel electrophoresis is the core technique for genetic analysis and purification of nucleic acids for further studies or disease diagnosis. Identifying Specific protein The rate of movement of macromolecules in an electric field is a helpful parameter to know any changes in amino acids regarding their charge. Quantitative analysis of specific serum protein classes such as gamma globulins and albumins It helps in the identification and quantitation of hemoglobin and its subclasses. It also helps in the identification of monoclonal protein in either serum or urine. Likewise, it helps in the separation and quantitation of significant lipoprotein classes. Immunoelectrophoresis helps to analyze several kinds of protein’s existence and how they behave chemically in different environments. It is also helpful in purifying proteins for different purposes. Similarly, it is useful in determining the molecular weights of protein. Coenzymes separation It is useful in separating and quantifying coenzymes such as creatine, kinase, lactate dehydrogenase, and alkaline phosphatase coenzyme to their respective subtypes. Analysis of chemical compounds It helps analyze compounds, such as water, soil, air quality or contamination, food quality, processing hygiene, and medical forensic analysis. It also helps in analyzing transition metals. Likewise, it helps to analyze organic compounds. Similarly, it helps in analyzing components of pesticides. Sieving effect and shape and size of molecules Size – bigger the molecule, greater the frictional and electrostatic forces exerted by the medium Larger particle has smaller μ Smaller particle has larger μ Shape – Round exert lesser frictional and electrophoretic retardation Globular protein moves faster than the fibrous protein. DIALYSIS By a choice of a membrane of a particular permeability which is wetted by the solvent it is possible to cause ions to pass through the membrane while large molecules of organic substances or colloidal particles are unable to pass thus the separation of solute can be accomplished and the term dialysis is justified. Dialysis is used extensively to remove electrolytes from colloidal suspensions to render the latter more stable. Chemical and environmental engineers use dialysis to recover sodium hydroxide from certain industrial wastes that have become contaminated with organic substances, as shown in fig.3 below. In the process, the waste material is placed in the cells with permeable membranes, and the cells are surrounded with water. The sodium and hydroxide ions pass through the cell wall into the surrounding water. The water is evaporated to recover the sodium hydroxide, and the organic waste remaining in the cells is disposed of separately. Waste caustic solution must be quite concentrated before recovery by dialysis can be justified economically. Mercerizing waste of cotton textile industry are example. A simple dialysis cell for recovery of sodium hydroxide from an industrial waste. ELECTRODIALYSIS In another application the dialysis principle can be used for demineralization of brackish water. In this case, the brackish water is placed both inside and outside the cell. Electrodes are placed in the water outside the cell and a current is applied, the ions within the cell are caused to flow through the semi permeable membrane and to concentrate in the water outside. The cations flow towards the anode. By this method, the water within the cell is demineralized. This process, termed electrodialysis, uses electrical energy to cause the flow of ions against a concentration. gradient. In practice, a large number of thin, continuous flow cells are used to make the process efficient for large-scaled usage. APPLICATIONS Electrodialysis system can be operated as continuous production or batch production processes. In a continuous process, feed is passed through a sufficient number of stacks placed in the series to produce the final desived product or concentrated quality. Electrodialysis is usually applied to deionization of aqueous solutions. However, desalting of sparingly conductive aqueous organic and organic solutions are also possible. Some application of electrodialysis include: 1. Brackish, seawater desalination and salt production is made possible 2. Small and medium scale drinking water production, nitrate reduction, in hotels, villages Constuction and military camps, hostels and hospitals. The major application of electrodialysis is the sesalination of brackish water as an alternative is reverse osmosis, RO, for potasle water production and salt production e.g japan. Limitation:- Electrodialysis work best at removing low molecular at eight ionic compounds from a feed stream. Non-charged, higher molecular weight and less mobile ionic species will not be readily removed. Electrodialysis becomes less economical when extremely low salt concentration in product are required as large membranes areas are required satisfy capacity requirements for low concentration feeds soluction. Measurement Techniques Gravimetric: Analytical balances routinely used for gravimetric analysis are sensitive to one tenth of a milligram, or one ten-thousandth of a gram. Most laboratories use electronic balances with direct digital readouts. For a measurement of the milligrams per liter of solids in the water, a measured volume of sample can be dried in a tared (pre-weighed) dish; the dish plus solids are weighed after the water has evaporated off; the weight of solids is calculated by subtraction, and the concentration figured by dividing the weight of solids by the volume of the sample. For a filtered sample, the tared filter itself is dried along with the solids it captured, and the suspended solids (those captured on the filter) calculated in the same way. In some chemical analyses, a precipitate is formed by reacting the analyte of interest with another chemical reagent (reacting chemical); then the precipitate can be filtered, dried, and weighed as a suspended solid. This type of analysis is more common with water solutions that are more concentrated than environmental samples, though, such as chemicals purchased for use in water or wastewater treatment. Electrochemical: The outer portions of all atoms and molecules consist of "shells" of electrons, and all chemical reactions involve interactions with these outer electrons-- sharing or transfer, or something in between. It is not surprising, then, that electricity and chemistry are interrelated (just think of batteries), and that electrical measurements can be used to detect and determine some substances of interest. The procedures involve placing electrodes in a water sample and measuring either an electrical potential (voltage), in millivolts, or a current, in milliamperes, which is related to the concentration of analyte. Depending on what they are designed to measure, electrodes can be simple pieces of metals such as gold, silver, platinum, copper, etc.; or they may be elaborate systems with semi-permeable membranes and several internal electrodes and filling solutions. The instrumentation may be capable of reading out directly in concentration units. Usually some sort of calibration procedure is necessary, using one or more standard solutions of known concentration. Colorimetry or spectrophotometry: This method involves measuring the intensity of a color in a solution and relating it to the concentration of the analyte. While some materials of interest are already colored, most of these analyses require the analyst to add some chemical reagents (reacting chemicals) to a sample to produce a characteristic color. The simplest type of measurement is visual comparison of the intensity of the color to a set of color standards which represent various concentrations of the analyte. While this is method does not require any expensive equipment, color perception is rather subjective-- and many people have some degree of color-blindness. A more precise measurement can be made using a colorimeter. A colorimeter is a device consisting of 1) Light source, which can be as simple as tungsten-filament light bulb; 2) Optics for focusing the light 3) Colored filter, which passes light of the color which is absorbed by the treated sample; 4) Sample compartment to hold a transparent tube or cell containing the sample, 5) light-sensitive detector, like the light meter on a camera, which converts the light intensity into an electric current, and 6) Electronics for measuring and displaying the output of the detector. Some colorimeters may be designed to read out directly in concentration units, while others may show the results in units of light absorbance which need to be compared to a calibration curve. (An interesting point is that the filter is not the same color as the solution being tested, but rather the complementary color. We want to use a filter which transmits light of the color which the solution absorbs. A yellow solution looks yellow because it absorbs blue light, so a blue filter would be used.) If we want to get more precise and more interference-free measurements, we can use a spectrophotometer. This is very similar to a colorimeter, except that instead of using a filter to select the color of light to pass through the sample, we instead break the white light up into a rainbow (spectrum) of colors using a prism or a diffraction grating. The light is passed through a narrow opening (slit) before reaching the sample. By rotating the prism or grating, the color {"wavelength") of light can be selected more precisely and we can better match the color with that absorbed by the sample. The principle is shown in the diagram below Fig. Spectrophotometer Needless to say, spectrophotometers cost more than colorimeters, and are likely to be more delicate and less portable, as well. While many tests are done using visible light, some analyses also make use of the invisible ultraviolet or infrared portions of the spectrum. Scanning spectro photometers can also be used to identify some types of analytes by the wavelengths or colors of the light they absorb. There is a variation of this type of testing, usually referred to as atomic spectroscopy, which is used mostly for trace metal analysis. The sample is converted to a gas by one of several methods- - usually involving heating. Then the light from a lamp containing the same metal is passed though the gas and the absorbance measured just as with a liquid sample (atomic absorption spectrophotometry). Alternatively, the intensity of the light emitted from the heated atoms of the metal in the gas can be used as a way of measuring the concentration (atomic emission spectrophotometry). A very popular atomic emission method in use today is called inductively coupled plasma spectrometry. The sample is carried in a stream of argon gas surrounded by coils which emit radio frequency energy that converts some of the gas into a very hot, ionized (electrically charged) form. An advantage of this method is that many elements can be measured simultaneously, or in rapid succession. Titration: Titration depends on using a well-defined chemical reaction to measure the amount of a standard solution needed to react with certain amount of the sample. A known volume, such as 100 mL, of sample is placed into a flask or beaker. The standard reagent is dispensed from a graduated tube called a burette so the volume used can be measured. The "end point" of the reaction is usually determined by observing a color change in an indicator solution, which is added to the flask before the start of the titration. End points are also often determined using electrochemical equipment. Once we know how much of the standard reagent was needed, we can calculate the amount of the analyte that is in the sample, because the reaction will always use the same proportion of the two materials. A common example is measuring the concentration of an acid by titrating with a standard base, such as sodium hydroxide. Chromatography: This technique got its name, which means "color picture", because it was first used to separate colored pigments from a single spot on a piece of paper. A solvent, such as alcohol, is allowed to move slowly across the paper, and the different components of the pigment travel at different rates. The result is a series of separated spots of different colors. They move at different rates because of differences in the pigments' relative attraction to the paper (the "stationary phase") and their solubility in the solvent (the "mobile phase"). This principle is used in modern instrumentation to separate mixtures of organic chemicals or inorganic ions. The components can be identified by their retention times, i.e., how long it takes them to pass through the instrument and detectors can be used to measure the amount of each component.. In gas chromatography, (or, simply, "GC") the mixture of substances is injected into a narrow, coiled column, several feet long, made of an inert material like glass, silica or stainless steel. The sample has usually been extracted into an organic solvent and concentrated by evaporation as a pretreatment step. The column may be filled with an oil-coated, powdered mineral, which forms the stationary phase. In the narrower capillary columns, the stationary phase is bonded directly to the wall of the tubing. The columns are usually contained in an oven, which may be programmable to raise the temperature at a controlled rate over time. Heating the column allows analysts to use this technique on many substances which are not gases at room temperature, including solvents and toxic chemicals like pesticides and PCB's. A continuous flow of an inert gas, such as argon, helium, or sometimes nitrogen, carries the evaporated mixture through the column. The substances are detected as they exit the column, usually by a technique that converts them into ions (electrically charged atoms or molecules), although one method uses heat conduction. The ions are produced by means such as flames, ultraviolet light, or radioactive materials. They are detected by being attracted to charged plates, where they produce an electrical current proportional to the amount present. The output of the detector usually is shown as a chart of "peaks" vs. time, called a chromatogram, often with the retention time and the intensity of the peak printed out. The retention time is used to identify the substance, while the height or area of the peak is used to quantify its concentration. A more positive identification is possible using a mass spectrometer as the detector. For substances which cannot easily be vaporized because of high boiling point or instability at higher temperatures, there is a liquid version of this technique know as HPLC (High pressure or high performance liquid chromatography). Organic solvents are used as the mobile phase. Ultraviolet (UV) light absorption is often used for detection. Herbicides and pharmaceuticals are common types of substances analyzed by this technique. Another variation of LC is ion chromatography, (IC), where the target analytes are charged inorganic or organic substances. The mobile phase is an aqueous (water-based) solution, and the stationary phase is made up of an ion exchange resin. The detectors usually measure electrical conductivity, although UV absorption can also be used. This technique can be used to measure the concentrations of several important inorganic anions, such as fluoride, sulfate, phosphate, and nitrate all in one analysis Mass Spectrometry: In a mass spectrometer, an ionized vapor is passed between magnets or radio frequency coils which separate the ions by mass (actually by charge to mass ratio). The pattern produced is characteristic of the particular substance, which can be identified by comparison with computerized "libraries" of mass spectra. While the instrumentation can be used alone, for environmental analyses it is usually used in tandem with another technique. Used as a "detector" for gas chromatography ("GC- MS"), it can positively identified components which have already been separated from a mixture. There is some use with liquid chromatography, as well (LC-MS). As a detector for metal ions produced in an ICP (see above), it provides very high sensitivity and is being used to determine very low levels of metal in drinking water, and may soon be approved for wastewater effluents and receiving waters. Radio Chemical Methods (e.g. Isotopic dilution methods) References Angerish A (2018). Study of Electrophoresis Techniques and its Types. Journal of Emerging Technologies and Innovative Research. 5(9): 299-304. Fritsch, R., & Krause, I. (2003). ELECTROPHORESIS. Encyclopedia of Food Sciences and Nutrition, 2055-2062. https://doi.org/10.1016/b0-12-227055-x/01409-7