Chapter 2 Minerals and Rocks PDF
Document Details
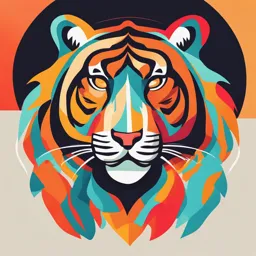
Uploaded by ResourcefulDecagon
ICCT Colleges
Tags
Summary
This document covers the fundamental concepts of minerals and rocks, including their properties (like luster and hardness), classification, and the elements that make up their composition. It also provides a table listing the eight most common elements in the Earth's crust.
Full Transcript
Module Earth and Life Science CHAPTER 2: EARTHY MATERIALS AND PROCESSES LESSON 2.1 MINERALS AND ROCKS 1. To identify common rock-forming minerals using their physical and chemical properties 2. To classify rocks into igneous, sedi...
Module Earth and Life Science CHAPTER 2: EARTHY MATERIALS AND PROCESSES LESSON 2.1 MINERALS AND ROCKS 1. To identify common rock-forming minerals using their physical and chemical properties 2. To classify rocks into igneous, sedimentary, and metamorphic Minerals Minerals are the fundamental components of rocks. They are naturally occurring inorganic substances with a specific chemical composition and an orderly repeating atomic structure that defines a crystal structure. Silicate minerals are the most abundant components of rocks on the Earth's surface, making up over 90% by mass of the Earth's crust. The common non-silicate minerals, which constitute less than 10% of the Earth's crust, include carbonates, oxides, sulfides, phosphates and salts. A few elements may occur in pure form. These include gold, silver, copper, bismuth, arsenic, lead, tellurium and carbon. Although 92 naturally occurring elements exist in nature, only eight of these are common in the rocks of the Earth's crust. Together, these eight elements make up more than 98% of the crust (Table 1). Oxygen (O) 46.6% Silicon (Si) 27.7% Aluminum (Al) 8.1% Iron (Fe) 5.0% Calcium (Ca) 3.6% Sodium (Na) 2.8% Potassium (K) 2.6% Magnesium (Mg) 2.1% Table 1. The eight most common elements in the Earth’s crust( by mass ) 1 Page Rock Forming Minerals: Module Earth and Life Science The physical properties of minerals, such as their hardness, lustre, color, cleavage, fracture, and relative density can be used to identify minerals. These general characteristics are controlled mainly by their atomic structure (crystal structure) 1. Luster – it is the quality and intensity of reflected light exhibited by the mineral a. Metallic – generally opaque and exhibit a resplendent shine similar to a polished metal b. Non-metallic – vitreous (glassy), adamantine (brilliant/diamond-like), resinous, silky, pearly, dull (earthy), greasy, among others. 2. Hardness – it is a measure of the resistance of a mineral (not specifically surface) to abrasion. a. hardness scale designed by German geologist/mineralogist Friedrich Mohs in 1812 (Mohs Scale of Hardness). b. The Mohs Scale of Hardness measures the scratch resistance of various minerals from a scale of 1 to 10, based on the ability of a harder material/mineral to scratch a softer one. c. Pros of the Mohs scale: i. The test is easy. ii. The test can be done anywhere, anytime, as long as there is sufficient light to see scratches. iii. The test is convenient for field geologists with scratch kits who want to make a rough identification of minerals outside the lab. d. Cons of the Mohs scale: i. The Scale is qualitative, not quantitative. ii. The test cannot be used to accurately test the hardness of industrial materials. 3. Crystal Form/Habit The external shape of a crystal or groups of crystals is displayed / observed as these crystals grow in open spaces. The form reflects the supposedly internal structure (of atoms and ions) of the crystal (mineral). It is the natural shape of the mineral before the development of any cleavage or fracture. Examples include prismatic, tabular, bladed, platy, reniform and equant. A mineral that do not have a crystal structure is described as amorphous. 4. Color and streak 2 Page Module Earth and Life Science a. A lot of minerals can exhibit same or similar colors. Individual minerals can also display a variety of colors resulting from impurities and also from some geologic processes like weathering. b. Examples of coloring: quartz can be pink (rose quartz), purple (amethyst), orange (citrine), white (colorless quartz) etc. c. Streak, on the other hand, is the mineral’s color in powdered form. It is inherent in almost every mineral, and is a more diagnostic property compared to color. Note that the color of a mineral can be different from its streak. d. Examples of streak: pyrite (FeS2) exhibits gold color but has a black or dark gray streak. e. The crystal’s form also defines the relative growth of the crystal in three dimensions, which include the crystal’s length, width and height. 5. Cleavage – the property of some minerals to break along specific planes of weakness to form smooth, flat surfaces a. These planes exist because the bonding of atoms making up the mineral happens to be weak in those areas. b. When minerals break evenly in more than one direction, cleavage is described by the number of cleavage directions, the angle(s) at which they meet, and the quality of cleavage (e.g. cleavage in 2 directions at 90°). c. Cleavage is different from habit; the two are distinct, unrelated properties. Although both are dictated by crystal structure, crystal habit forms as the mineral is growing, relying on how the individual atoms in the crystal come together. Cleavage, meanwhile, is the weak plane that developed after the crystal is formed. 6. Specific Gravity – the ratio of the density of the mineral and the density of water a. This parameter indicates how many times more the mineral weighs compared to an equal amount of water (SG 1). b. For example, a bucket of silver (SG 10) 3 would weigh ten times more than a bucket Page of water. Module Earth and Life Science 7. Others – magnetism, odor, taste, tenacity, reaction to acid, etc. For example, magnetite is strongly magnetic; sulfur has distinctive smell; halite is salty; calcite fizzes with acid as with dolomite but in powdered form; etc. Common rock-forming minerals: These are specimens of minerals from the University of Auckland's collection. Along with the common rock-forming minerals, including apatite, corundum, diamond, fluorite, topaz and talc to illustrate minerals used in Moh's Scale of Hardness. apatite augite biotite calcite chlorite corundum diamond fluorite garnet gypsum hornblende ilmenite magnetite muscovite Classification and Identification of Minerals Minerals are classified according to their chemical composition. 1. Definite fixed composition, Quartz is always SiO2, and calcite is always CaCO3. 2. Form both by inorganic and organic processes. For example, calcite (CaCO3) is a common vein mineral in rocks, and also a shell- forming material in many life forms. Calcite of organic origin conforms to the above definition except for the requirement that it be inorganic. 3. "Mineraloids" While not truly falling into the category of minerals, they are still usually classified as minerals. Two well-known examples are Mercury, which lacks a crystal structure due to its liquid state, and Opal, which also lacks a crystal structure as well as a definitive chemical formula. Despite the fact that these mineraloids lack certain essential characteristics of minerals, they are classified as minerals in most reference guides including the acclaimed Dana's System of Mineralogy. 4. Organic minerals is another unique category of minerals. While this term is technically an oxymoron, since the definition of a mineral requires it to be inorganic, there are several naturally occurring rare organic substances with a definitive chemical formula. The best example of this is Whewellite. Most reference guides and scientific sources make an exception to these substances and still classify them as minerals. Rocks Rock or stone is a naturally occurring solid aggregate of one or more minerals. The Earth's outer solid layer, the lithosphere, is made of rock. The types and abundance of minerals in a rock are deter-mined by the manner in which the rock was formed. Many rocks contain silica (SiO2); a compound of silicon and oxygen that forms 74.3% of the Earth's crust. This material forms crystals with other compounds in the rock. 4 Page Geological Classification of rocks according to Characteristics such as Module Earth and Life Science 1. mineral and chemical composition, 2. permeability, 3. the texture of the constituent particles, 4. and particle size. These physical properties are the end result of the processes that formed the rocks. Over the course of time, rocks can transform from one type into another, as des-cribed by the geological model called the rock cycle. These events produce three general classes of rocks : igneous , sedimentary, and metamorphic. 1. Igneous: Igneous rocks form from the cooling of melted rock (either lava or magma) into solid form. If the cooling occurs underground, the rock is an intrusive, or plutonic, igneous rock. If the cooling occurs on the earth's surface, the rock is an extrusive or volcanic rock. Molten material within the Earth is called magma; it is “lava” once it has erupted onto the surface. 2. Metamorphic: Metamorphic rocks form when existing rocks are subjected to intense heat and pressure, usually deep below the earth's surface. These conditions change the original minerals of the rock into new minerals. 3. Sedimentary: Sedimentary rocks are either detrital or chemical. a. Detrital rocks are formed by the compaction of separate particles, or sediments, into a rock. b. Chemical sedimentary rocks form from minerals that have been dissolved in water and precipitate out, forming a solid rock. Geologists describe sedimentary rocks according to the size and shape of the particles in them or their mineral composition (in the case of chemical sedimentary rocks). 5 Page Module Earth and Life Science Rock Cycle The rocks of earth's crust are constantly being recycled and changed into new forms through geologic processes. This continual transformation of rocks from one type to another is called the rock cycle. How rock type can be changed? Rock can be changed through the processes of weathering, heating, melting, cooling, and compaction. Any one rock type can be changed into a different rock type as its chemical composition and physical characteristics are transformed. The minerals and metals found in rocks have been essential to human civilization. Link for Learning: Rocks and Minerals (https://www.youtube.com/watch?v=ZkHp_nnU9DY) Lesson 2.2 : EXOGENIC PROCESSES Objectives: 1. define weathering and distinguish between the two main types of weathering 2. I can identify the factors that affect the rate of weathering 3. identify the different agents of erosion and deposition 4. describe characteristic surface features and landforms created and the processes that contributed to their formation 5. Identify the controls and triggers of mass wasting 6. Distinguish between different mass wasting processes ❖ Exogenic process includes geological phenomena and processes that originate externally to the Earth’s surface. ❖ Generally related to the: 6 atmosphere, Page hydrosphere and Module Earth and Life Science biosphere, and therefore to processes of: o weathering, o erosion, o transportation, o deposition, o denudation etc. ❖ Exogenic factors and processes could also have sources outside Earth, for instance under the influence of the Sun, Moon, etc. The above mentioned processes constitute essential landform-shaping factors. Their rate and activity very often depends on local conditions, and can also be accelerated by human actions. The combined functions of exogenic and endogenic factors influences the present complicated picture of the Earth’s surface. Mountains, valleys and plains seem to change little, if at all, when left to nature, but they do change continuously. The features of the Earth’s surface temporary forms in a long sequence of change that began when the planet originated billions of years ago, and is continuing today. The process that shaped the crust in the past are shaping it now. By understanding them, it is possible to imagine, in a general way, how the land looked in the distant past and how it may look in the distant future. Landforms are limitless in variety. Some have been shaped primarily by: streams of water, glacial ice, waves and currents and movements of the Earth‘s crust or volcanic eruptions. These are landscapes typical of deserts and others characteristic of humid regions. The arctic makes its special mark on rock scenery, as do the tropics. Because geological conditions from locality to locality are never quite the same, every landscape is unique. Rock at or near the surface of the continents breaks up and decomposes because of exposure. The processes involved are called weathering. Weathering Weathering is the decomposition and disintegration of rocks and minerals at the Earth’s surface. Erosion Erosion is the removal of weathered rocks and minerals by moving water, wind, glaciers and gravity. The four processes – weathering, erosion, transportation and deposition work together to modify the earth’s surface. The Work of Weathering Weathering produces some landforms directly, but is more effective in preparing rocks for removal by mass wasting and erosion. Weathering influences relief in every landscape. 7 Page Module Earth and Life Science Types of Weathering 1. Mechanical Weathering Freezing and thawing ❖ Water expands when it freezes. ❖ If water accumulates in a crack and then freezes, its expansion pushes the rock apart and the process is called frost wedging. ❖ In a temperate climate, water may freeze at night and thaw during the day. ❖ Ice cements the rock temporarily, but when it melts, the rock fragments may tumble from a steep cliff. ❖ Large piles of loose angular rocks, called talus slopes, lie beneath many cliffs. These rocks fell from the cliffs mainly as a result of frost wedging. Salt crystal growth ❖ force exerted by salt crystal that formed as water evaporates from pore spaces or cracks in rocks can cause the rock to fall apart Abrasion – ❖ wearing away of rocks by constant collision of loose particles Biological activity – ❖ plants and animals as agents of mechanical weathering 2. Chemical Weathering Dissolution – ❖ dissociation of molecules into ions; common example includes dissolution of calcite and salt Oxidation- ❖ reaction between minerals and oxygen dissolved in water Hydrolysis- ❖ change in the composition of minerals when they react with water Factors That Affect The Type, Extent, And Rate At Which Weathering Takes Place: a. Climate – areas that are cold and dry tend to have slow rates of chemical weathering and weathering is mostly physical; chemical weathering is most active in areas with high temperature and rainfall b. Rock type – the minerals that constitute rocks have different susceptibilities to weathering. Those that are most stable to surface conditions will be the most resistant to weathering. Thus, olivine for example which crystallizes at high temperature conditions will weather first than quartz which crystallizes at lower temperature conditions. c. Rock structure- rate of weathering is affected by the presence of joints, folds, faults, bedding planes through which agents of weathering enter a rock mass. Highly-jointed/fractured rocks disintegrate faster than a solid mass of rock of the same dimension d. Topography- weathering occurs more quickly on a steep slope than on a gentle one e. Time- length of exposure to agents of weather determines the degree of weathering of a rock 8 Page Module Earth and Life Science EROSION ❖ the incorporation and transportation of material by a mobile agent such as water, wind, or ice Agents of Erosion 1. Running water - encompasses both overland flow and stream flow ❖ Overland flow - Runoff that flows down the land slopes in broadly distributed sheets ❖ Stream Flow - water runs along a narrow channel between banks Factors that Affect stream erosion and deposition ✓ Velocity – dictates the ability of stream to erode and transport; controlled by gradient, channel size and shape, channel roughness, and the amount of water flowing in the channel ✓ Discharge – volume of water passing through a cross-section of a stream during a given time; as the discharge increases, the width of the channel, the depth of flow, or flow velocity increase individually or simultaneously 2. Ocean and Sea waves 3. Glaciers - a moving body of ice on land that moves downslope or outward from an area of accumulation (Monroe et. al., 2007) Types of glaciers: a. Valley (alpine) glaciers — bounded by valleys and tend to be long and narrow b. Ice sheets (continental glaciers) — cover large areas of the land surface; unconfined by topography. Modern ice sheets cover Antarctica and Greenland c. Ice shelves — sheets of ice floating on water and attached to the land. They usually occupy coastal embayment. 9 Page Module Earth and Life Science 4. Wind a. Wind erodes by: deflation (removal of loose, fine particles from the surface), and abrasion (grinding action and sandblasting) b. Deflation results in features such as blowout and desert pavement. Abrasion yields ventifacts and yardangs. c. Wind, just like flowing water, can carry sediments such as: (1) bed load (consists of sand hopping and bouncing through the process of saltation), and (2) suspended load (clay and silt-sized particles held aloft). 5. Groundwater a. The main erosional process associated with groundwater is solution. Slow-moving groundwater cannot erode rocks by mechanical processes, as a stream does, but it can dissolve rocks and carry these off in solution. This process is particularly effective in areas underlain by soluble rocks, such as limestone, which readily undergoes solution in the presence of acidic water. b. Rainwater reacts with carbon dioxide from atmosphere and soil to form a solution of dilute carbonic acid. This acidic water then percolates through fractures and bedding planes, and slowly dissolves the limestone by forming soluble calcium bicarbonate which is carried away in solution. 6. Gravity Mass wasting — the downslope movement of soil, rock, and regolith under the direct influence of gravity 10 Page Module Earth and Life Science Factors that control mass wasting processes include: a. As the slope angle increases, the tendency to slide down the slope becomes greater. b. Role of water: adds weight to the slope, has the ability to change angle of repose, reduces friction on a sliding surface , and water pore pressure reduces shear strength of materials Mass Wasting Processes a. Slope failures - sudden failure of the slope resulting in transport of debris downhill by rolling, sliding, and slumping. i. Slump – type of slide wherein downward rotation of rock or regolith occurs along a curved surface ii. Rock fall and debris fall– free falling of dislodged bodies of rocks or a mixture of rock, regolith, and soil in the case of debris fall 11 Page Module Earth and Life Science iii. Rock slide and debris slide- involves the rapid displacement of masses of rock or debris along an inclined surface b. Sediment flow - materials flow downhill mixed with water or air; Slurry and granular flows are further subdivided based on velocity at which flow occurs i. Slurry flow – water-saturated flow which contains 20-40% water; above 40% water content, slurry flows grade into streams 1) Solifluction – common wherever water cannot escape from the saturated surface layer by infiltrating to deeper levels; creates distinctive features lobes and sheets of debris 2) Debris flow – results from heavy rains causing soil and regolith to be saturated with water; commonly have a tongue-like front; Debris flows composed mostly of volcanic materials on the flanks of volcanoes are called lahars. Rodolfo, K.S. (2000) in his paper “The hazard from lahars and jokulhaups” explained the distinction between debris flow, hyperconcentrated flow and mudflow: debris flow contains 10-25 wt% water, hyperconcentrated stream flow has 25-40 wt% water, 12 Page Module Earth and Life Science and mudflow is restricted to flows composed dominantly of mud 3) Mud flow – highly fluid, high velocity mixture of sediment and water; can start as a muddy stream that becomes a moving dam of mud and rubble; differs with debris flow in that fine-grained material is predominant ii. Granular flow – contains low amounts of water, 0-20% water; fluid-like behavior is possible by mixing with air 1) Creep – slowest type of mass wasting requiring several years of gradual movement to have a pronounced effect on the slope ; evidence often seen in bent trees, offset in roads and fences, inclined utility poles. Creep occurs when regolith alternately expands and contracts in response to freezing and thawing, wetting and drying, or warming and cooling 13 Page Module Earth and Life Science 2) Grain flow – forms in dry or nearly dry granular sediment with air filling the pore spaces such as sand flowing down the dune face 3) Debris avalanche – very high velocity flows involving huge masses of falling rocks and debris that break up and pulverize on impact; often occurs in very steep mountain ranges. Some studies suggest that high velocities result from air trapped under the rock mass creating a cushion of air that reduces friction and allowing it to move as a buoyant sheet SUBAQUEOUS MASS WASTING Subaqueous mass movement occurs on slopes in the ocean basins. This may occur as a result of an earthquake or due to an over-accumulation of sediment on slope or submarine canyon. 3 types: a. Submarine slumps - similar to slumps on land 14 Page Module Earth and Life Science b. Submarine debris flow – similar to debris flows on land c. Turbidity current – sediment moves as a turbulent cloud EVENTS THAT TRIGGER MASS WASTING PROCESSES. a. Shocks and vibrations – earthquakes and minor shocks such as those produced by heavy trucks on the road, man-made explosions b. Slope modification – creating artificially steep slope so it is no longer at the angle of repose c. Undercutting – due to streams eroding banks or surf action undercutting a slope d. Changes in hydrologic characteristics – heavy rains lead to water-saturated regolith increasing its weight, reducing grain to grain contact and angle of repose; e. Changes in slope strength – weathering weakens the rock and leads to slope failure; vegetation holds soil in place and slows the influx of water; tree roots strengthen slope by holding the ground together f. Volcanic eruptions - produce shocks; may produce large volumes of water from melting of glaciers during eruption, resulting to mudflows and debris flows Links for Learning: Exogenic and Endogenic processes 15 (https://www.youtube.com/watch?v=8iaXljsMItY) Weathering and Erosion Basics Page (https://www.youtube.com/watch?v=CNUzTmPKxv8) Physical Geology:Mass Wasting, various types (https://www.youtube.com/watch?v=Egq6wS5wAUA) Module Earth and Life Science Lesson 2.3: Endogenic Process Objectives 1. Know the sources and significance of the Earth's internal heat 2. Explain how and why magma rises up 3. Discuss the history behind the Theory of Continental Drift; 4. Describe the Continental Drift Theory; and 5. Enumerate and explain the evidence used to support the idea of drifting continents Endogenic processes include tectonic movements of the crust, magmatism , metamorphism, and seismic activity. Endogenic processes have been responsible for shaping the earth’s relief and the formation of many of the important mineral resources. The principal energy sources for endogenic processes are: 1. heat 2 the redistribution of material in the earth’s interior according to density - The earth’s deep heat originates chiefly from radiation. - The continuous generation of heat in the earth’s interior results in the flow of heat toward the surface. With the proper combination of materials, temperature, and pressure, chambers and layers of partial melting may occur a t certain depths within the earth. The asthenosphere, the primary source of magma formation, is such a layer in the upper mantle. Convection currents may arise in the asthenosphere and they are hypothesized to be lithosphere. In the zones of the volcanic belts of the island arcs and continental margins, the principal magma chambers are associated with super deep dip faults, slanting beneath the continents from the ocean side to depths of about 700 km. Under the influence of the heat flow or under the direct influence of the heat carried by rising abyssal magma , magma chambers form in the crust itself. Reaching the near surface parts, the magma is intruded into them in the form of variously shaped intrusive bodies or is extruded onto the surface , forming volcanoes. Gravitational differentiation has led to the stratification of the earth into geospheres of varying density. Is also manifested in the form of tectonic movements , which, in turn, lead to the tectonic deformation of crustal and upper mantle rocks. The accumulation and subsequent discharge of tectonic stresses along active faults causes earthquakes. THE CONCEPT OF CONTINENTAL DRIFT i. The idea that continents fit together like pieces of a jigsaw puzzle has been around since the 1600s, although little significance was given to it. 16 ii. The continental drift hypothesis was first articulated by Alfred Wegener, a German Page meteorologist, in 1912. He proposed that a single supercontinent, Pangaea, separated into the Module Earth and Life Science current continents and moved across Earth’s surface to their present locations. He published his work through a book entitled “The Origin of Continents and Oceans” in 1915. iii. Until the 1950s-60s, it was still widely held that that continents and ocean basins had fixed geographic positions. As such, scientists were reluctant to believe that continents could drift. What was the driving mechanism? iv. In the 1960s, the post-war boom in oceanography generated a lot of new data about the ocean floor. It turned out that the ocean floor was not as flat and featureless as they had originally thought. The ocean floor was characterized by deep depressions called trenches and a network of ridges that encircled the globe. These topographic data, together with heat flow measurements, led to the emergence of the Seafloor Spreading Hypothesis which revived interest in Alfred Wegener’s idea of drifting continents. Animation of Continental Drift (http://www.tectonics.caltech.edu/outreach/animations/drift.html) It is hypothesized that a combination of these processes leads to the temporal unevenness of the release of heat and light matter toward the surface , which , in turn , can be explained by the occurrence of tectonic magmatic cycles in the history of the earth’s crust. The spatial irregularities of the same abyssal processes may explain division of the crust into more or less geologically active regions, for example, into geosynclines and platforms. Study Question: What is the difference between exogenic and endogenic process ? Links for Learning: Continental Drift (https://www.youtube.com/watch?v=rM8KrmRedSw) The Origins of Magma (https://www.youtube.com/watch?v=WtSE1svxRm4) For more information: Lesson 2.4: DEFORMATION OF THE CRUST Various Methods of Measuring Ocean Depths a. Sounding line – weighted rope lowered overboard until it touched the ocean bottom; this old method is time-consuming and inaccurate b. Echo sounding– type of sonar which measures depth by emitting a burst of high frequency sound and listening for the echo from the seafloor. Sound is emitted from a source on the ship and the returning echo is detected by a receiver on the ship. Deeper water means longer time for 17 the echo to return to the receiver. Page Module Earth and Life Science c. Satellite altimetry – profiles the shape of the sea surface by measuring the travel time of a radar pulse from the satellite to the ocean surface and back to the satellite receiver. The shape of the sea surface approximates the shape of the sea floor. Different Features of The Ocean Floor a. Continental margin – submerged outer edge of the continent where continental crust transitions into oceanic crust i. Passive or Atlantic type – features a wide, gently sloping continental shelf (50- 200m depth), a steeper continental slope (3000-4000m depth), and a flatter continental rise. ii. Active or Pacific type – characterized by a narrow shelf and slope that descends into a trench or trough b. Abyssal plains and abyssal hills – abyssal plain is an extremely flat, sediment-covered stretches of the ocean floor, interrupted by occasional volcanoes, mostly extinct, called seamounts. Abyssal hills are elongate hills, typically 50-300m high and common on the slopes of mid oceanic ridge (Note: figure above is not a very good representation of abyssal hill). These hills have their origins as faulted and tilted blocks of oceanic crust. c. Mid-ocean ridges – a submarine mountain chain that winds for more than 65,000 km around the globe. It has a central rift valley and rugged topography on its flanks. Mid-ocean ridges are cut and offset at many places by transform faults. The trace of a transform fault may extend away from either side of the ridge as a fracture zone which is older and seismically inactive. d. Deep-ocean trenches- narrow, elongated depressions on the seafloor many of which are adjacent to arcs of island with active volcanoes; deepest features of the seafloor. e. Seamounts and volcanic islands – submerged volcanoes are called seamounts while those that rise above the ocean surface are called volcanic islands. These features may be isolated or found in clusters or chains. 18 Page Module Earth and Life Science SEAFLOOR SPREADING HYPOTHESIS In 1960, Harry Hess advanced the theory of seafloor spreading. Hess proposed that seafloor separates at mid-ocean ridges where new crust forms by upwelling magma. Newly formed oceanic crust moves laterally away from the ridge with the motion like that of a conveyor belt. Old oceanic crusts are dragged down at the trenches and re-incorporated back into the mantle. The process is driven by mantle convection currents rising at the ridges and descending at the trenches. This idea is basically the same as that proposed by Arthur Holmes in 1920. Proof for Seafloor Spreading Magnetic stripes on the seafloor: detailed mapping of magnetism recorded in rocks of the seafloor shows that these rocks recorded reversals in direction and strength of the Earth’s magnetic field. Alternating high and low magnetic anomalies run parallel to mid ocean ridges. Pattern of magnetic anomalies also matches the pattern of magnetic reversal already known from studies of continental lava flows. Deep sea drilling results: Age of seafloor forms a symmetric pattern across the mid- oceanic ridges, age increases with distance from the oceanic ridge; no seafloor older than 200 million years could be found, indicating that seafloor is constantly being created and destroyed. Theory of Plate Tectonics The concept of plate tectonics was formulated in the 1960s. According to the theory, Earth has a rigid outer layer, known as the lithosphere, which is typically about 100 km (60 miles) thick and overlies a plastic (moldable, partially molten) layer called the asthenosphere. The lithosphere is broken up into seven very large continental- and ocean-sized plates, six or seven medium-sized regional plates, and several small ones. These plates move relative to each other, typically at rates of 5 to 10 cm (2 to 4 inches) per year, and interact along their boundaries, where they converge, diverge, or slip past one another. Such interactions are thought to be responsible for most of Earth’s seismic and volcanic activity, although earthquakes and volcanoes can occur in plate interiors. 19 Plate motions cause mountains to rise where plates push together, or converge, and continents to fracture and oceans to form where plates pull apart, or diverge. Page Module Earth and Life Science The continents are embedded in the plates and drift passively with them, which over millions of years results in significant changes in Earth’s geography. There are three main types of plate boundaries: 1. Convergent boundaries: where two plates are colliding. Subduction zones occur when one or both of the tectonic plates are composed of oceanic crust. The denser plate is subducted underneath the less dense plate. The plate being forced under is eventually melted and destroyed. i. Where oceanic crust meets ocean crust Island arcs and oceanic trenches occur when both of the plates are made of oceanic crust. Zones of active seafloor spreading can also occur behind the island arc, known as back-arc basins. These are often associated with submarine volcanoes. ii. Where oceanic crust meets continental crust The denser oceanic plate is subducted, often forming a mountain range on the continent. The Andes is an example of this type of collision. iii. Where continental crust meets continental crust Both continental crusts are too light to subduct so a continent-continent collision occurs, creating especially large mountain ranges. The most spectacular example of this is the Himalayas. 2. Divergent boundaries – where two plates are moving apart. The space created can also fill with new crustal material sourced from molten magma that forms below. Divergent boundaries can form within continents but will eventually open up and become ocean basins. i.On land Divergent boundaries within continents initially produce rifts, which produce rift valleys. 20 ii. Under the sea The most active divergent plate boundaries are between oceanic plates and are Page often called mid-oceanic ridges. Module Earth and Life Science 3. Transform boundaries – where plates slide passed each other. The relative motion of the plates is horizontal. They can occur underwater or on land, and crust is neither destroyed nor created. Because of friction, the plates cannot simply glide past each other. Rather, stress builds up in both plates and when it exceeds the threshold of the rocks, the energy is released – causing earthquakes. Links for Learning: Features of the Ocean basin (https://www.youtube.com/watch?v=wP380-Iaoos) Seafloor Spreading Lecture (https://www.youtube.com/watch?v=DZL5GWaLviY&t=27s) Plate Tectonics: Evidence of Pate Movement (https://www.youtube.com/watch?v=6EdsBabSZ4g) Lesson 2.5: HISTORY OF EARTH In the very beginning of earth's history, this planet was a giant, red hot, roiling, boiling sea of molten rock - a magma ocean. The heat had been generated by the repeated high speed collisions of much smaller bodies of space rocks that continually clumped together as they collided to form this planet. As the collisions tapered off the earth began to cool, forming a thin crust on its surface. As the cooling continued, water vapor began to escape and condense in the earth's early atmosphere. Clouds formed and storms raged, raining more and more water down on the primitive earth, cooling the surface further until it was flooded with water, forming the seas. 21 It is theorized that the true age of the earth is about 4.6 billion years old, formed at about the same time as the rest of our solar system. The oldest rocks geologists have been able to Page find are 3.9 billion years old. Using radiometric dating methods to determine the age of rocks Module Earth and Life Science means scientists have to rely on when the rock was initially formed (as in - when its internal minerals first cooled). In the infancy of our home planet the entire earth was molten rock - a magma ocean. Since we can only measure as far back in time as we had solid rock on this planet, we are limited in how we can measure the real age of the earth. Due to the forces of plate tectonics, our planet is also a very dynamic one; new mountains forming, old ones wearing down, volcanoes melting and reshaping new crust. The continual changing and reshaping of the earth's surface that involves the melting down and reconstructing of old rock has pretty much eliminated most of the original rocks that came with earth when it was newly formed. So the age is a theoretical age. When Did Life on Earth Begin? Scientists are still trying to unravel one of the greatest mysteries of earth: When did "life" first appear and how did it happen? It is estimated that the first life forms on earth were primitive, one-celled creatures that appeared about 3 billion years ago. That's pretty much all there was for about the next two billion years. Then suddenly those single celled organisms began to evolve into multicellular organisms. Then an unprecedented profusion of life in incredibly complex forms began to fill the oceans. Some crawled from the seas and took residence on land, perhaps to escape predators in the ocean. A cascading chain of new and increasingly differentiated forms of life appeared all over the planet, only to be virtually annihilated by an unexplained mass extinction. It would be the first of several mass extinctions in Earth's history. Scientists have been looking increasingly to space to explain these mass extinctions that have been happening almost like clockwork since the beginning of "living" time. Perhaps we've been getting periodically belted by more space rocks (ie. asteroids), or the collision of neutron stars happening too close for comfort? Each time a mass extinction occurred, life found a way to come back from the brink. Life has tenaciously clung to this small blue planet for the last three billion years. Scientists are finding new cues as to how life first began on earth in some really interesting places - the deep ocean. Checking the Fossil Record Scientists have studied rocks using radiometric dating methods to determine the age of earth. Another really cool thing they've found in rocks that tells us more about the story of earth's past are the remains of living creatures that have been embedded in the rocks for all time. We call these fossils. It has been the careful study of earth's fossil record that has revealed the exciting picture about the kinds of creatures that once roamed this planet. Fossilized skeletons of enormous creatures with huge claws and teeth, ancient ancestors of modern day species (such as sharks) that have remained virtually unchanged for millions of years, and prehistoric jungles lush with plant life, all point to a profusion of life and a variety of species that continues to populate the earth, even in the face of periodic mass extinctions. By studying the fossil record scientists have determined that the earth has experienced very different climates in the past. In fact, general climactic conditions, as well as existing species, are used to define distinct geologic time periods in earth's history. For example, periodic warming of the earth - during the Jurassic and Cretaceous periods - created a profusion of plant and 22 animal life that left behind generous organic materials from their decay. These layers of organic material built up over millions of years undisturbed. They were eventually covered by younger, Page Module Earth and Life Science overlying sediment and compressed, giving us fossil fuels such as coal, petroleum and natural gas. Alternately, the earth's climate has also experienced periods of extremely cold weather for such prolonged periods that much of the surface was covered in thick sheets of ice. These periods of geologic time are called ice ages. Entire species of warmer-climate species died out during these time periods, giving rise to entirely new species of living things which could tolerate and survive in the extremely cold climate. Believe it or not, humans were around during the last ice age - the Holocene (about 11,500 years ago) - and we managed to survive. Creatures like the Woolly Mammoth - a distant relative of modern-day elephants - did not. Read about a really exciting recent find of a perfectly-preserved, frozen Woolly Mammoth! This was a particularly exciting find because it wasn't a fossil that scientists found, but actual tissue, which still has its DNA record intact. Also, read more about the Ice Man - another frozen tissue sample of a human being who was frozen into the high mountains of France. He was just recently discovered as thousands of years of ice pack have finally melted from around his body. Rocks in the mantle and the core are still hot from the formation of the Earth about 4.6 billion years ago. When the Earth formed, material collided at high speeds. These collisions generated heat (try clapping your hands together - they get hot) that heat became trapped in the Earth. There is also heat within the earth produced by radioactive decay of naturally-occurring radioactive elements. It is the same process that allows a nuclear reactor to generate heat, but in the earth, the radioactive material is much less concentrated. However, because the earth is so much bigger than a nuclear power plant it can produce a lot of heat. Rocks are good insulators so the heat has been slow to dissipate. This heat is enough to partially melt some rocks in the upper mantle, about 50-100 km below the surface. It partially melt because the rocks don't completely melt. Most rocks are made up of more than one mineral, and these different minerals have different melting temperatures. This means that when the rock starts to melt, some of the minerals get melted to a much greater degree than others. The main reason this is important is that the liquid (magma) that is generated is not just the molten equivalent of the starting rock, but something different. The most common type of magma produced is basalt (the stuff that is erupted at mid- ocean ridges to make up the ocean floors, as well as the stuff that is erupted in Hawai'i). Soon after they're formed, little drops of basaltic magma start to work their way upward (their density is slightly less than that of the solid rock), and pretty soon they join with other drops and eventually there is a good flow of basaltic magma towards the surface. If it makes it to the surface it will erupt as basaltic lava. Link for Learning: The Geological History of Earth (https://www.youtube.com/watch?v=Z6k3NRy-YWs) 23 Page