Cellular Physiology - Intro PDF
Document Details
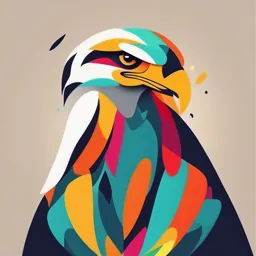
Uploaded by ReputableBouzouki6951
Northeastern University
Tags
Summary
This document provides an introduction to cellular physiology, covering topics such as cell types, evolution of the cellular concept, basic cell composition, and homeostasis. It includes details about surface area to volume ratio and different types of cells.
Full Transcript
Modern Physiology - Physiology is an academic discipline - Modern physiology is a synthetic academic discipline - Includes classical physiology, cellular physiology, and molecular biology - Physiology, cellular physiology, and pharmacology are close disciplines Cell is the Basic...
Modern Physiology - Physiology is an academic discipline - Modern physiology is a synthetic academic discipline - Includes classical physiology, cellular physiology, and molecular biology - Physiology, cellular physiology, and pharmacology are close disciplines Cell is the Basic Unit of Life - Evolution of the cellular concept - 1665 Robert Hooke -- Introduced concept of the cell - 1670s Antoine van Leeuwenhoek -- Biological observation using microscope - 1833 Robert Brown -- nucleus - 1838 Schleiden and Schwann -- Cellular Theory: all organisms composed of cells; foundation of modern biology - Cellular Theory -- Schleiden and Schwann (1838) beginning of modern biology - Cellular Pathology -- Virchow (1848) beginning of modern medicine - Cellular Pharmacology -- Ehrlich (1899) beginning of pharmacology Basic Cell composition - Cells are mostly composed of six atoms: carbon, oxygen, hydrogen, nitrogen, calcium, and phosphorus - (99% of net weight) - Four atoms: Carbon, hydrogen, oxygen, and nitrogen, amount to 96% of net weight. Thus, basic empirical formula may be approximated as C4H7O2N1 - The other five atoms: sodium, potassium, chlorine, magnesium, and sulfur amount to 0.85% of net weight. These 11 elements are necessary for life. The rest are 60 trace elements Cell types - Cells were originally divided into two main classes by whether they contain a nucleus - Traditional biological classification -- two groups: - Prokaryotes: single cell, non-nucleus (bacteria 1-5 um) - Eukaryotes: nucleolus (plants, animals, fungi, algae, and protozoa (10-100 um) - New biological classification -- three domains - Bacteria (prokaryotes divided into bacteria and archae) - Archae - Eukaryotes Surface area/volume ratio - Surface area/volume ratio is the major constrain of the cell - The surface area determine the size of cell membrane - Size of the cell membrane determine the transport - The internal volume determines the metabolism - The surface area increases with the square of the cell radius - The volume increases with the cube of the cell radius Increase in S \--\> Increase in T Decrease in S \--\> Decrease in T Increase in V \--\> Increase in M Decrease in V \--\> Decrease in M Homeostasis -- Major Concept in Physiology - Claude Bernard 1857: - The fixity of the internal environment is the condition of free and independent life. All the vital mechanisms, however, varied\.... have only one object that is of preserving constant conditions\...of the internal environment - The function of all organ systems of the organism is to maintain the internal environment relatively constant - GOAL IS \--\> SUSTAIN NORMAL INTERNAL ENVIRONMENT, ANY IMBALANCE IS A DISEASED STATE Homeostasis multicellular organism -- parameters maintained 1. Energetic substrate 2. O2 and CO2 3. Products of metabolism 4. PH 5. Electrolyte concentration 6. Water 7. Temperature 8. Volume and Pressure Cell Physiology Nanoscale - Hydrogen atom diameter: 01 nM - Carbon atom diameter: 0.16 nM - Sulfur atom diameter: 0.2 nM - Water molecule: 0.1 nM - Ionic channel: 0.2 nM - Amino acid: 0.8 nM - Glucose: 1 nM - DNA diameter: 2 nM - Globular protein: 4 nM (typical proteins are from 2-10nm -- more typically 4-5nm) - Insulin: 5 nM - Microfilaments/Hb: 6 nM - Biological membrane size: 7 nM - Antibody: 10 nM - Ribosome/Rhinovirus: 20 nM - Microtubule: 25 nM - Hepatitis virus: 30 nM - Nuclear pore: 50 nM - HIV: 100 nM - Very large viruses (Rhabdoviruses, Paramyxoviruses): 150-250 nM - Small bacteria: 150-250 nM - Lysosomes/Peroxisomes: 200 nM - Wavelength of visible light: 350-750 nM - Giant viruses: 800 nM - Mitochondria/Bacteria: 1000 nM (1 um) - Eukaryotic cell (average cell size): 10000 nM (10 um) Matter at Nanoscale \*\* - Gravity demonstrates little effect - Intermolecular forces dominate - Surface tension dominates - Behavior of electrons is different at nanoscale - Quantum effects become prominent - Physical phenomena negligible at a macroscopic level, become the major dominant effect at the micro-and nanoscale Frequencies at Nanoscale - Biological objects are characterized by resonance frequencies. Frequency (V) is inversely related to wavelength (λ) - V= λ-1 and V\~L-1 - Thus, resonance frequences are larger in small biological objects and smaller in large objects - Decrease size (L) \-\--\> Increase V - Increase size (L) \-\--\> Decrease V Four types of cells/tissue 1. Muscle (striated and smooth) 2. Nervous (neurons and supporting cells) 3. Epithelial (epithelial membranes and glands) 4. Connective (large extracellular spaces with extracellular material) - Organs: structures typically composed of four primary types of tissue Elements of Cellular Evolution: Primitive atmosphere - 1920 -- suggested that simple organic molecules formed in early earth's primitive atmosphere - Simple molecules -- spontaneously polymerize in primitive earth's atmosphere (operating at extremely low PO2) - Primitive atmosphere of earth is thought to have contained little free oxygen - Such an atmosphere provides reducing conditions - Energy such as sunlight or electrical discharge, can form organic molecules spontaneously Elements of Cellular Evolution: Formation of Organic Molecules - The spontaneous formation of organic molecules was demonstrated in 1950s - In Miller's experiments electric sparks were introduced into a mixture of H2, CH4, NH3, and H2O - Electrical sparks formed organic molecules, including amino acids - Miller's experiments did not precisely reproduce the conditions of primitive earth - However, demonstrated the possibility of the spontaneous synthesis of organic molecules Elements of Cellular Evolution: Formation of Macromolecules and the RNA world - The next step in evolution was the formation of macromolecules - The monomers of macromolecules polymerized spontaneously under prebiotic conditions - Mixtures of amino acids, for example, result in polymerization to form polypeptides - Macromolecules related to life must have the ability to replicate itself - Macromolecule making copies of itself may be capable of reproduction - Only the nucleic acids can direct their own self-replication RNA world - In the 1980s it was discovered that RNA is capable of catalyzing chemical reactions - RNA can cause the polymerization of nucleotides - RNA can serve as a template and to catalyze its own replication - It was suggested that self-replicating RNA was the initial genetic system - A period of early chemical evolution known as the RNA world - The self-replicating RNA and associated molecules were enclosed in a phospholipid membrane - Phospholipid structure was maintained as a unit, capable of self reproduction and further evolution - RNA-directed synthesis probably evolved by this time, in which case - Thus the first cell could consist of self-replicating RNA and encoded proteins Evolution of prokaryotes and eukaryotes - The prokaryotic evolution lasted 1 to 1.5 billion years - The eukaryotes developed at least 2.7 billion years ago, following prokaryotic evolution - DNA sequences indicate that the archaebacteria and eubacteria are different from each other - Present-day eukaryotes are different from archaebacteria and eubacteria - The divergence of three lines of descent from a common ancestor occurred early in evolution - Common ancestor gave rise to present-day archaebacteria, eubacteria, and eukaryotes Elements of evolution: Prokaryotic cells 1 - Present-day prokaryotes, are divided into two groups -- the archaebacteria and eubacteria - Archaebacteria and eubacteria diverged early in evolution - Some archaebacteria reside in extreme environments -- like primitive earth - For example, thermoacidophiles live in hot sulfur springs at 80C and pH about 2 Elements of evolution: Prokaryotic cells 2 - Prokaryotic cells (bacteria) lack a nucleus with a nuclear envelope - Prokaryotic cells are generally smaller and simpler than eukaryotic cells - Prokaryotic cells do not contain cytoplasmic organelles or a cytoskeleton - Prokaryotic cells genomes are less complex - The same basic molecular mechanisms govern both prokaryotes and eukaryotes - The same basic mechanisms indicate that all present-day cells are descended from a single primordial ancestor Prokaryotes (bacteria) - Bacteria is a large group of organisms - Live in a wide range of environments: soil, water, and other organisms (e.g., human pathogens) - Most bacterial cells are spherical, rod-shaped, or spiral, with diameters of 1 to 10 um) - The DNA is from 0.6 million to 5 million base pairs - The DNA is capable to encode about 5000 different proteins - A typical prokaryotic cell is illustrated by E. coli - A common inhabitant of the human intestinal tract - The cell is rod-shaped, about 1 um in diameter and about 2 um long - Like most prokaryotes, E. coli is surrounded by a rigid cell wall Modern prokaryotic cell (E. Coli) - Cell wall is composed of polysaccharides and peptides - The cell wall is easily penetrated by a wide range of molecules - The plasma membrane separates between the inside of the cell and the external environment - The plasma membrane is a typical selective phospholipid bilayer with proteins - The DNA of E. coli is a single circular molecule and is not separated from the cytoplasm - The cytoplasm contains approximately 30,000 ribosomes Cyanobacteria are the largest and most complex prokaryotes that developed photosynthesis -- photosynthetic bacteria Elements of Evolution - Eukaryotic cells - The origin of eukaryotes is a huge enigma and a major challenge for evolutionary biology - There is a sharp divide in the organizational complexity between eukaryotes and prokaryotes - Eukaryotes have a complex intracellular compartmentalization - The most sophisticated prokaryotes (archaea and bacteria) do not have a compartmentalization - The characteristic eukaryotic complexity arose almost ready made - There are no intermediate stages between the prokaryotes and eukaryotes - This leap in complexity of eukaryotes is one of the principal challenges in evolutionary biology Eukaryotic cells contain membrane-enclosed organelles - Eukaryotic cells are generally much larger than the prokaryotic cells - In eukaryotes cell volume is at least a thousandfold greater than in prokaryotes - Eukaryotic cells are surrounded by plasma membrane - Eukaryotic cells contain a variety of cytoplasmic organelles and a cytoskeleton - The compartmentalization (organelles) allow eukaryotic cells to be efficient Yeast as an example of eukaryotes - Eukaryotes may be unicellular organisms capable of self-replication - The simplest eukaryotes are the yeasts - Yeasts are more complex than bacteria - Yeasts are smaller and simpler than the cells of animals or plants Amoeba as an example of eukaryotes - Some unicellular eukaryotes are complex cells, containing as much DNA as human cells - May be specialized to perform a variety of tasks, including photosynthesis and movement - Some unicellular capture and digest other organisms - Amoeba proteus, for example, is a large, complex cell - Its volume is more than 100,000 times that of E. coli, and its length can exceed 1 mm - Amoebas are highly mobile organisms that use cytoplasmic extensions (pseudopodia) - Amoebas move and engulf other organisms, including bacteria, yeasts, and protozoa The last eukaryote common ancestor (LECA) - All extant eukaryotic forms evolved from a last eukaryote common ancestor (LECA) - A large set of genes, in the area light of phylogenomics, are conserved across eukaryotes - All eukaryotes share the main features of cellular architecture and regulation - Generally, cellular architecture and regulation of eukaryotes are different than prokaryotes - The ancestral features of some eukaryotic systems are increasingly detected in prokaryotes Eukaryotic cells and the kinase-phosphatase regulatory system - The complexity of the eukaryotic cells is provided by an extremely sophisticated signaling system - The main signaling systems in eukaryotes is the kinase-phosphatase system - The kinase-phosphatase system regulates protein function - The kinase-phosphatase through phosphorylation and dephosphorylation Nucleus - Nucleus is an essence of eukaryotic cells - Stores genetic information in the form of DNA - Largest organelle in the eukaryotic cell - Surrounded by a nuclear envelope (two membranes - outer and inner) - Envelope contains nuclear pores - Nucleus diameter is about 5 um - In eukaryotes DNA is organized as linear structure - The nucleus is the site of DNA replication and of RNA synthesis - The translation of RNA occurs on ribosomes in the cytoplasm Organelles - Endoplasmic reticulum - Golgi complex - Ribosomes - Lysosomes - Peroxisomes - Mitochondria - Chloroplast - Cytoskeletal elements Nuclear envelope - Double membrane separated by perinuclear space about 20-40 nm - Outer membrane is continuous with the lumen of the ER - Outer membrane is often studded with ribosomes Nuclear pores - Specialized channel - Provide direct contact between nucleoplasm and cytoplasm - Mammalian nucleus about 3000-4000 pores (10-20 pores per 1um) - The rate of transport is inversely related to the size of the molecule - Increase in size \-\--\> Decrease in transport - Decrease in size \-\--\> Increase in transport \*Smaller molecules enter by free diffusion \*Bigger molecules enter by active diffusion Endoplasmic reticulum (1 per cell) 1. Mechanical support 2. Involved in protein synthesis (granular ER) 3. Involved in lipid synthesis (agranular ER) 4. Formation of new cell membranes 5. System for transport molecules 6. Involved in metabolism of xenobiotics - Endoplasmic reticulum (ER) is an extensive network of intracellular membranes - Eukaryotic cells are large size cells and protein transport is a formidable task - The ER extends from the nuclear membrane throughout the cytoplasm - ER participates in the transport and processing of proteins and lipid synthesis - From the ER, proteins within membrane vesicles are transported to the Golgi apparatus - ER and the Golgi apparatus sort and transport proteins to the plasma membrane and lysosomes Golgi complex (1 to hundreds per cell) - In the Golgi apparatus, proteins are processed and sorted for transport to their destinations - Golgi apparatus is a site of lipid synthesis - Golgi complex in plant cells synthesize of some of the cell wall polysaccharides - Synthesized proteins and lipids collect in the smooth ER - Packed into transport vesicles - Transport vesicles fuse with Golgi complex and modify product into final form - Golgi complex sorts and directs product to the appropriate destination - Secretory vesicles fuse with the membrane and release product outside the cell - Transport vesicles - Secretory vesicles (200 times larger than transport vesicles) Mitochondria and Chloroplast -- endosymbiotic theory (eukaryotes) - 1883 -- chloroplast - is a result of symbiosis between bacteria and the cell - 1920 -- a symbiotic origin of mitochondria was suggested - 1960 -- mitochondria and chloroplast contain their own DNA - 1970 -- mitochondria and chloroplast are semiautonomous structures - Contain DNA/RNA/ribosomes Eukaryotic cells and mitochondria - The hallmark of the eukaryotic cell is the presence of mitochondria - It is suggested that a last common ancestor (LECA) already possessed mitochondria - There are no direct counterparts to mitochondria in archaea or bacteria - Mitochondria have a central role in energy transformation, signaling, and cell death Mitochondria 1. Outer membrane a. Metabolic enzymes b. Transport proteins c. Contains porins d. Freely permeable to ions and small molecules up to 5000 dalton 2. Inner membrane (folded into cristae) e. Enzymes of oxidative phosphorylation 3. Matrix f. Enzymes of citric acid cycle \*Increase Ac \--\> Increase M \* Decrease Ac \--\> Decrease M Mitochondria and Chloroplast - Mitochondria and chloroplast play critical roles in energy metabolism - Mitochondria are found in almost all eukaryotic cells - Mitochondria are the sites of oxidative metabolism and ATP generation - Chloroplast is found in plant cells - Chloroplasts are the sites of photosynthesis - Chloroplasts are found only in the cells of plants and unicellular eukaryotes (green algae) - Mitochondria and chloroplasts are similar to bacteria in size, and reproduce by dividing in two - Mitochondria and chloroplasts contain their own genetic systems - Mitochondria and chloroplasts contain their own DNA - DNA encodes some of the components of mitochondria and chloroplasts - DNA of the mitochondria and chloroplasts replicate when the organelle divides - Genes are transcribed within the organelle and translated on organelle's ribosomes - DNA of the mitochondria and chloroplasts are distinct from the nuclear genome of the cell - Ribosomes and ribosomal RNAs of mitochondria and chloroplasts are more closely related to bacteria than to the eukaryotes Endosymbiosis and evolution - An endosymbiotic origin mitochondria and chloroplast is now generally accepted - Endosymbiotic associations during evolution are highly advantageous for the participants - Mitochondria suggested to have evolved from the aerobic bacteria - The acquisition of aerobic bacteria provided an anaerobic cell with the oxidative metabolism - Chloroplasts are suggested to have evolved from photosynthetic bacteria (cyanobacteria) - The acquisition of photosynthetic bacteria provided photosynthesis and nutritional independence - During evolution, original bacterial genes were incorporated into the nuclear genome of the cell - Only a few components of mitochondria/chloroplasts are still encoded by the organelle genomes Nucleus -- Viral origin hypothesis (General outline) - There is an old dispute in biology -- whether viruses are alive biological objects - Viral eukaryogenesis (Philip Bell 2001) explains the origin of the nucleus in eukaryotes - First step: - According to the hypothesis, cell nucleus evolved from the DNA virus - During evolution change from ancient RNA to DNA first occurred viruses - Endosymbiosis of a large DNA virus with a bacteria formed the eukaryotic virus - Second step: - During evolution, the virus gradually acquired genes from the host genome - During evolution, the virus eventually evolved into the eukaryotic nucleus - Theory is, somewhat, supported by the discovery of large DNA viruses (Mimi virus) - Mimi virus synthesizes proteins (500-700nm) Viral origin hypothesis -- summary - Virus was modified in a nucleus by obtaining genes from archaeal and bacterial cells - The virus became the information center for the cell operation - The cell provided mechanisms of gene translation - General functioning of the cell remained, mostly, unchanged - Thus, the bacterial cells preserved the glycolytic ATP production - Storage of genetic information was a function of a new virus-formed nucleus The evolution of metabolism - The controlled utilization of metabolic energy is central to all cell activities - Adenosine 5'-triphosphate ATP is suggested to be the major direct source of metabolic energy - It is suggested that during evolution ATP synthesis evolved in three evolutionary stages - Glycolysis, photosynthesis, and oxidative phosphorylation - These metabolic pathways changed earth's atmosphere, and changed the course of evolution