Cells Producing Energy BIOB10H3 Summer 2024 PDF
Document Details
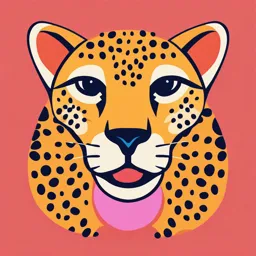
Uploaded by IntelligibleAloe
2024
BIOB10H3
Cecilia Gimenez, PhD
Tags
Summary
This document provides a detailed overview of cell structures and functions for producing energy based on lecture materials.
Full Transcript
Cells producing energy: Structure and function of the mitochondria and chloroplasts Lecture 9 BIOB10H3-Summer 2024 1 Cecilia Gimenez, PhD Mitochondria and chloroplasts Big organelles that can be seen under the light...
Cells producing energy: Structure and function of the mitochondria and chloroplasts Lecture 9 BIOB10H3-Summer 2024 1 Cecilia Gimenez, PhD Mitochondria and chloroplasts Big organelles that can be seen under the light microscope! Mitochondria and chloroplasts have originated from bacteria and have retained the bacterial energy- conversion mechanisms as well as other features of their ancestry. Mitochondria and chloroplasts have an outer and an inner membrane. The inner membrane in mitochondria and the thylakoid membrane in the chloroplast have the machinery for cellular respiration and photosynthesis, respectively. Both organelles have a genetic system. 2 A shared mechanism to harness energy Both share a fundamental mechanism for harnessing energy. This mechanism is known as chemiosmotic coupling [chemical bond–forming reactions that generate ATP (“chemi”) and membrane transport processes (“osmotic”)]. Chemiosmotic coupling occurs in two linked stages and it is performed by the coordinated action of many protein complexes embedded in the inner membrane. Stage 2 Stage 1 3 Electron-conversion mechanisms in mitochondria and chloroplast The mitochondrion converts energy from chemical fuels, whereas the chloroplast converts energy from sunlight. The electrons pass through a series of protein complexes embedded in the inner membrane. 4 Only chloroplasts can be seen using conventional light microscopy. a. True. b. False. 5 CHECK POINT 6 Mitochondria: Structure and function 7 Mitochondria are heterogeneous and dynamic organelles Mitochondria are heterogeneous (in structure and function), highly dynamic organelles that are constantly changing shape, dividing, and fusing. They are often associated with the cell cytoskeleton (more coming in Lecture 10) and can travel long distances in certain cell types (neurons), while remain almost static in others (muscle cells and sperm). 8 Mitochondria specialize into distinct tissue-specific mitochondrial phenotypes that are highly variable in both structure and function https://doi.org/10.1038/s42255-023-00783-1 9 Mitochondria are heterogeneous and dynamic organelles Mitochondria are surrounded by a double-membrane system, consisting of inner and outer mitochondrial membranes separated by an intermembrane space. The inner membrane forms numerous folds (cristae), which extend into the interior (or matrix) of the organelle. http://dx.doi.org/10.5772/58965 10 2 membranes + 2 aqueous compartments The matrix It is highly concentrated in proteins (gel-like). Contains the genetic system of the mitochondria, which is composed of a plethora of enzymes, circular DNA and ribosomes. Encodes for less than half of the proteins that the mitochondria needs to function-Many many proteins need to be imported (tagged)! The matrix, along with the cristae, are the places where food-derived molecules are converted into energy (ATP). 11 The inner and outer membranes of mitochondria are different in structure and function Inner mitochondrial membrane The inner membrane encloses the matrix and contains all the protein complexes involved in the electron transport chain and the ATP synthase. More than 100 different proteins! It has ADP/ATP carrier proteins that carries ADP in and the produced ATP out. The inner membrane has cristae (thin folds). Almost impermeable. Has many channels and pumps. Enriched in cardiolipin (more coming in slide 22). Embedded are the translocon complexes 12 (TIM complex for protein import). Mitochondrial cristae increase the efficiency of ATP synthesis Sites of high membrane curvature in the cristae are concentrated in respiratory enzyme complexes and ATP synthase dimers. This topology of distribution creates a proton “sink” at the tip of the invaginations. The cristae seems to act as “proton traps” that concentrate protons close to the ATP synthase and make the synthesis of ATP more effective (despite the small gradient between the matrix and the cytosol). H+ This arrangement of complexes and ATP synthase are absent in chloroplasts (see more in slide 34). 13 The inner and outer membranes of mitochondria are different in structure and function Outer mitochondrial membrane Sets the boundaries of the mitochondria. The outer membrane is permeable to ions and small molecules and have β- barrel-type membrane proteins that create aqueous channels (porins) (go back to lecture 7). Porins are the most abundant proteins in the mitochondrial outer membrane. Exchange of NADH and ATP. Translocon complexes (TOM complex for protein import). 14 Mitochondrial fission and fusion A balance exists between mitochondria division (fission) and fusion, and these two processes must preserve the structure and function of the two membranes. Fission is driven by dynamin (go back to lecture 8), while fusion involves two different set of proteins to coordinate the sequential fusion of the outer and then tie inner membranes. 15 Dynamin is a mechanoenzyme involved in: a. Endocytosis. b. Phagocytosis. c. Autophagy. d. Mitochondria fission. e. All of the above. f. Only a, b and d are correct. 16 Mitochondria-endoplasmic reticulum contact sites Mitochondria form contacts with other organelles, in particular the endoplasmic reticulum. This physical and functional bridge create specific domains to facilitate the exchange of molecules (lipids and proteins) and ions. Mitochondria buffer Ca+2 concentration by taking it from the ER. Important in Ca+2 homeostasis. Constriction of the ER around the mitochondrion assist the fission process. 17 https://doi.org/10.1016/j.cell.2023.11.040 Mitophagy Dysfunctional mitochondria are cleared by an organelle-specific form of autophagy known as mitophagy (go back to Lecture 8). In most mammals, during the maturation of immune red blood cells (reticulocytes), these cells perform active mitophagy as part of its maturation process. https://www.monash.edu/discovery-institute/lazarou-lab/research 18 Which of the following is NOT true about mitochondria? a. Mitochondrial structural and functional phenotypes greatly vary between cell types. b. The mitochondrial matrix contains the genetic system of the mitochondria; however, many proteins are encoded in the nuclear DNA and need to be imported after synthesis. c. Mitochondria inner membrane is enriched in α-barrel proteins. d.The inner membrane of the mitochondria has an ADP/ATP transporter e. None of the above. 19 CHECK POINT 20 Mitochondria: Function 21 Mitochondria are multiphasic organelles Mitochondria have cell-type specific phenotypes and perform several interconnected functions. https://doi.org/10.1038/s42255-023-00783-1 22 Mitochondria have vital roles in cellular SOME! metabolism 23 1- ATP Production Mitochondria can use a variety of food-derived substrates as fuel. The “fuels "are converted to acetyl CoA (intermediary) by enzymes located in the mitochondrial matrix. acetyl CoA are oxidized in the matrix via the Krebs cycle. This cycle produces the waste product CO2, which diffuses out. The cycle saves the energy released by the oxidation of acetyl CoA in the form of electrons carried by NADH. These electrons are transferred from the matrix to the electron-transport chain (in the inner mitochondrial membrane). Through the chemiosmotic coupling process the energy that was carried by NADH electrons is converted into ATP. 24 The path of electrons through the respiratory chain: generation of the electrochemical gradient During the electron-transfer reactions, protons are pumped across the membrane by each of the 3 respiratory enzyme complexes (Complex I, Complex III, and Complex IV). Electrons from the oxidation of succinate by succinate dehydrogenase (designated as Complex II) are fed into the electron-transport chain in the form of reduced ubiquinone. Complex II does not pump protons and thus does not contribute to the proton-motive force. 25 The electrochemical gradient drives the synthesis of ATP The electrochemical gradient across the inner mitochondrial membrane (created by the 3 proton pumps described in slide 19) drives the synthesis of ATP by the ATP synthase. 26 The ATP synthase is a nanomachine It can be compared with the structure of a turbine, composed by a rotor and a stator. Also called F1Fo ATP synthase or F-type ATPase (go back to Lecture 7). It synthesizes ATP from ADP and phosphate (P) in the F1 head. Arranged as dimers in the tip of the cristae (essential for cristae formation and maintenance). It can work in forward (to produce ATP) or in reverse (to hydrolyze ATP). Proton flow through Fo (the rotor) makes the rotor stalk rotate where the catalytic site for ATP synthesis is located (F1 head). The mechanical force produced by the rotor stalk is converted in chemical energy (ATP). To avoid the non-specific rotation of the head, a stalk at the periphery (yellow) connects the head to the stator subunits, which are inserted in the inner membrane. 27 CHECK POINT 28 Which of the following is false about the mitochondrial ATP-synthase? a. It can work in forward (to produce ATP) or in reverse (to hydrolyze ATP). b. Proton flow through Fo makes the “rotor stalk” rotate where the catalytic site for ATP synthesis is located (F1 head). c. ATP synthase dimers are located at the tip of the cristae invaginations. d. It is a F-type ATPase. e. None of the above. 29 2-Biogenesis of heme groups and iron sulfur clusters Heme (an iron-porphyrin) groups are essential for electron transport. They are present in some of the complexes and covalently attached to cytochrome c (remember iron transport via clathrin- dependent endocytosis? Go back to Lecture 8). Heme group biogenesis is shared between the cytosol and the mitochondria. Iron-sulfur clusters are ensembles of iron and sulfide produced in the mitochondrial. Iron-sulfur clusters are co-factors essential for electron transfer through Complexes I, II and III to cytochrome c, before subsequent transfer to molecular oxygen https://doi.org/10.1016/j.redox.2021.102164 30 Porphyria and the myth of vampirism Porphyria is a rare inherited blood disease due to a group of metabolic disorders of the heme biosynthetic pathway (altered enzymatic activity). Some conditions will trigger porphyria attacks, characterized by the accumulation of porphyrin precursors in the body, which are toxic. It was also known as the vampire disease, because people suffering from it will experience signs and symptoms that shared characteristics with them. It is a systemic disease with multiple potential contributions to mitochondrial dysfunction and oxidative stress. 31 3- Synthesis of phospholipids In the mitochondria phospholipids for the biogenesis of the mitochondrial membrane and others are synthesized (cardiolipin, phosphatidylethanolamine, phosphatidylglycerol and phosphatidic acid. Cardiolipin is a two-headed phospholipid exclusively present in the mitochondrial membranes. It is found in many bacterial membranes. Cardiolipin interacts with proteins involved in oxidative phosphorylation and the transport of ATP. Also, in cristae its disposition might support membrane curvature. 32 4- Regulation of apoptosis Apoptosis (AKA programmed cell death type I) can be activated from the outside (extrinsic pathway) or from the inside (intrinsic pathway) The intrinsic pathway, AKA the mitochondrial pathway, is often in response to developmental signals or to injury such as DNA damage. It depends on the release of mitochondrial proteins (e.g. cytochrome c). In the cytosol, mitochondrial proteins activate caspase proteases that dismantle cells and signal efficient phagocytosis of dead cells. This process is tightly controlled by the Bcl-2 family members, which regulate the release of proteins from the intermembrane space to the cytosol. (BH3)-only proteins inhibit the anti-apoptotic proteins such us BCL-2 that otherwise will keep the pro-apoptotic proteins BAK and BAX inactive. Oligomerization of BAK and BAX results in mitochondrial outer membrane permeabilization. This results in the release of cytochrome c which ultimately leads to the activation of caspases resulting in cell death. 33 https://doi.org/10.1038/nrd.2016.253 Mitochondrial diseases Group of genetic disorders caused by mutations in genes in the nuclear DNA and mitochondrial DNA. Unlike nuclear DNA the mitochondrial DNA is inherited only from mother to child (review conception egg vs sperm). Symptoms of mitochondrial disease (including neurological and non-neurological) are highly variable, even within the same family, and these is because both healthy and damage mitochondria can co-exist (cells have thousands of mitochondria-heteroplasmic mitochondrial DNA). 34 CHECK POINT 35 Chloroplasts: Structure 36 Chloroplasts are big organelles! Present in plants (including algae) and photosynthetic bacteria, they are responsible for capturing light energy to make sugars in a process called photosynthesis (only water and carbon dioxide are required as inputs). Oxygen is released to the environment as a product of this process. Contain chlorophyll to absorb light energy (it is located within the thylakoid membrane). Chloroplasts are generally larger than mitochondria but its internal organization is very similar. It has an outer and inner membrane, but instead of cristae it has thylakoid compartments. The stroma is analogous to the mitochondrial matrix. It contains metabolic enzymes and the ATP synthesis machinery, as well as the chloroplast’s genetic system (ribosomes, RNAs, and the chloroplast DNA). -90% of the chloroplast proteins are imported TOC/TIC complexes. 37 Thylakoid compartments Thylakoid compartments are made of the thylakoid membrane and internal thylakoid lumen. The thylakoid lumen is a third compartment not connected with the intermembrane space, nor with the stroma. The thylakoid membrane corresponds to the mitochondrial cristae, but it is not connected to the inner chloroplast membrane at any point. It is where photosynthesis and the synthesis of ATP take place. Thylakoid membrane is highly folded into numerous local stacks of flattened vesicles called granum or grana, interconnected by no stacked thylakoids (stroma lamellae). https://doi.org/10.1038/nrm3027 38 The thylakoid lumen in chloroplasts is the equivalent of the mitochondrial matrix. a. True. b. False. 39 Chloroplasts in a plant cell 40 Chlorophil Functions in light Conversion of light energy into chemical energy -occurs Absorption entirely within the thylakoid membranes of chloroplasts -requires the chlorophyll (green pigment) inserted in the thylakoid membranes. Structure: Long hydrophobic tail (green) + porphyrin ring with an extensive system of delocalized electrons (bonds in blue). A magnesium atom is held in a porphyrin ring (like iron Anchors in heme-mitochondria). chlorophyll to Chlorophy ll–protein complexes can transfer either thylakoid excitation energy or electrons. membrane 41 CHECK POINT 42 Chloroplasts: Function 43 The process of photosynthesis Process by which plants use CO2 and H20 to make carbohydrates. The set of reactions that happen during photosynthesis in chloroplasts can be divided into 2 categories: 1- Photosynthetic electron-transfer reactions (“light reactions”)- light dependent. 2- The carbon-fixation reactions (“dark reactions”)- light independent. 44 1- Light reactions 1-A photon (a quantum of light) knocks an electron out of the chlorophyll in the first reaction center (photosystem II), creating a positively charged chlorophyll ion (chlorophyll readily gains its electron from water to produce H+ and oxygen). 2- The electron then travels to a second reaction center (photosystem I) (electron STROMA movement is similar to that through the respiratory chain in mitochondria). 3- Electrons and H+ are stored into NAPDH. 4-The energy released by electron transfer (II) drives the pumping of H+ inside the thylakoid space. 5-The resulting electrochemical proton (I) gradient is used by the ATP synthase to drive the synthesis of ATP in the stroma. 45 A bit more detail 1- Electrons taken out from a water molecule (held by the manganese cluster in photosystem II) and protons are released into the thylakoid space by water oxidation. 2- The electrons pass on to plastoquinone, to the cytochrome b6-f complex, at the same time protons are pumped into the thylakoid space (the equivalent of the cytochrome c reductase). 3- Electrons move to photosystem I by the soluble electron carrier plastocyanin (equivalent of cytochrome c), then transferred to ferredoxin-NADP+ reductase (FNR) by the soluble carrier ferredoxin (Fd). 4- The H+ consumed during NADPH formation in the stroma, contribute to the generation of the electrochemical H+ gradient across the thylakoid membrane, which drives ATP synthesis by an ATP synthase. 46 2- Dark reactions NADPH and ATP produced in (1) enter the carbon-fixation cycle to drive the conversion of CO2 into glycerolaldehyde 3-phosphate, a critical intermediary sugar that will serve has the starting material for the synthesis of sugars, amino acids, and fatty acids. 47 CHECK POINT 48 During carbon fixation, which key molecule is produced using the electron carrier and ATP coming from light reactions? a. Atmospheric oxygen. b. Atmospheric carbon dioxide. c. Water. d. Glycerolaldehyde 3-phosphate. e-Phosphate. 49 Which of the following descriptions applies to dark reactions rather than light reactions in plant chloroplasts? a. They produce the sugar glyceraldehyde 3-phosphate. b. They involve the electron-transfer chain embedded in the thylakoid membrane. c. They involve O2 production. d. They generate ATP. 50 Chloroplasts and mitochondria work together In plant cells, chloroplasts convert light energy into chemical energy, and mitochondria consume the chemical energy to produce ATP. The optimal carbon fixation and plant growth require these two energy- transforming organelles to perform strictly coordinated actions. In red mitochondria surrounding chloroplasts. Chloroplast-mitochondrion contact sites have been suggested (physical association). 51 Chloroplasts as niches for viral replication in plants Many plant viral pathogens change and/or rearrange chloroplasts for viral replication (membranous niches). (A) Normal chloroplast. (B-F) Abnormal chloroplasts (viral-induced). Chlorosis- leaves become yellow- defects in chlorophyl production is the most common viral symptom. Viral influence on chloroplast structures and functions usually leads to depleted photosynthetic activity. 52 Why do leaves change color in the fall? When daylight hours are less and temperatures are cooler, photosynthesis slows down and there is less chlorophyll production. This reduction reveals yellow or orange pigments called carotenoids. Anthocyanins are produced mainly in the fall. A reaction between sugars and certain proteins in cell sap forms anthocyanins. This reaction does not occur until the sugar concentration in the sap is quite high. The cool fall temperatures cause the closing of leaf veins and prevent sugars from moving out which prolongs fall color. Unlike chlorophyll and carotene, anthocyanins are not attached to cell membranes, but are dissolved in the cell sap. 53 When daylight hours are less and temperatures are cooler, photosynthesis slows down and the production of chlorophyll…….. a. Slows down. b. Stays at the same level. c. Slows down but quickly rises. d. Rises. 54 Want to delve deeper in these topics? Chapter: 14. 55 Next: LECTURE 10 The cell cytoskeleton and motors: Molecular organization, function and experimental approaches. 56