BMS 200 - Physiology of the Cardiac Cycle PDF
Document Details
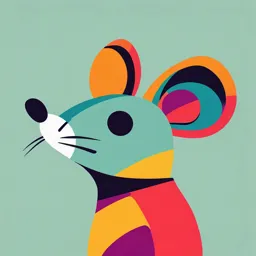
Uploaded by HandierMesa
CCNM - Boucher Campus
null
null
Tags
Summary
This document details the BMS 200 Physiology of the Cardiac Cycle. It contains learning outcomes related to cardiac anatomy and the cardiac cycle, alongside questions and diagrams. The document's focus is on the concepts of ventricular pressure, volume, and their curves.
Full Transcript
BMS 200 – Physiology of the Cardiac Cycle Learning Outcomes Explain the following features of the Wigger diagram in the context of cardiac anatomy and the physiology of the cardiac cycle: Pressure changes in the right and left ventricles, right and left atria, pulmonary artery and aorta...
BMS 200 – Physiology of the Cardiac Cycle Learning Outcomes Explain the following features of the Wigger diagram in the context of cardiac anatomy and the physiology of the cardiac cycle: Pressure changes in the right and left ventricles, right and left atria, pulmonary artery and aorta Isovolumetric contraction and relaxation in the right and left ventricle Ventricular volume during systole and diastole The phonocardiogram and electrocardiogram Describe the contribution of passive filling, atrial contraction, and length of diastole to ventricular filling Define the following parameters and apply them to the determination of cardiac output: End diastolic volume, end-systolic volume, stroke volume, ejection fraction, heart rate Define the following parameters and describe the physiologic basis for their impact on stroke volume: contractility, preload, afterload Interpret the PV loop to determine: Stroke volume, end-systolic volume, end-diastolic volume, systolic and diastolic blood pressure Contractility, afterload, preload, and the diastolic pressure curve Overall workload of the ventricle Explain why preload, afterload, and contractility maintain a consistent cardiac output across the left and right ventricles Question: Which ventricle pumps more blood/minute? The right or the left ventricle? Factors to consider: o The pressures that both ventricles develop o The thickness of the walls o Their vascular connections Force of Contraction The force that a cardiomyocyte generates with each systole depends on two things: ▪ Amount of calcium available to bind to troponin – this is known as inotropy Factors that increase inotropy: ▪ Increased sympathetic nervous system stimulation ▪ Increased heart rate (“loads” more calcium in the SR during relaxation) ▪ Things that increase SNS effectiveness – thyroid hormone, cortisol, etc. ▪ Optimal overlap between actin and myosin during diastole This is mostly determined by the state of ventricular filling – also known as preload Factors that affect strength of contraction in skeletal muscle Positive inotropic agents, like certain hormones (e.g., epinephrine) and drugs (e.g., digitalis), can increase the force of contraction, leading to increased sarcomere shortening. Negative inotropic agents, on the other hand, reduce the force of contraction, which can be associated with reduced sarcomere shortening. Factors that affect strength of contraction in skeletal muscle Better overlap of actin and myosin → increased force development ▪ In the top diagram, the bottom black line represents the preload (prior to contraction) ▪ the red line directly above it represents the force that is generated during systole at that preload Review: ECG - timing How do the ECG waves and intervals correspond to the excitation along the conduction pathway? The Wiggers Diagram Commonly includes: ▪ Pressures in the left ventricle, aorta, and left atrium ▪ ECG ▪ Left ventricular volume ▪ Phonocardiogram The Wiggers Diagram - Pressures Examine the atrial pressure tracing (bottom dashed line) What causes the following phenomena in the left atrium? ▪ a, c, and v waves ▪ x and y descent x descent y descent The Wiggers Diagram - Pressures Hint – note the location of the P- wave in the ECG ▪ a wave ▪ x-descent ▪ c wave x descent ▪ v wave y descent ▪ y-descent Atrial Pressure Tracing A Wave – Atrial contraction (atrial systole) C Wave – Bulging of tricuspid X Wave – Atrial relaxation (atrial diastole) V Wave – Passive filling of the atria (ventricular systole) Y Wave – Emptying of atria into the ventricles with the opening of AV valves (early diastole). https://www.ncbi.nlm.nih.gov/books/NBK2213/ Ventricular pressure and volume curves Important terms: Isovolumetric – there is a change in pressure, but no change in volume ▪ are valves open or closed? Systole – when the chamber applies pressure work to blood through contraction Diastole – when the chamber no longer applies pressure work to blood through contraction Ventricular pressure and volume curves The Wiggers diagram is a good way to illustrate that the ventricle cannot: ▪ eject blood into the great artery until its pressure is greater than that in the artery ▪ accept blood from the atria unless its pressure is less than that in the atria Valves ensure that blood only flows one direction despite the large changes in ventricular pressure Ventricular pressure and volume curves Note the rapid and slower phases of ventricular filling, as well as the final “bump” in volume as the atria contract Rapid ventricular filling is due to the rapid expansion of the ventricle and the drop in volume that ensues ▪ Also known as passive filling, and it is responsible for 80% of ventricular filling at rest ▪ Passive filling takes time – decreased with increased heart rates Aortic pressure curve Aortic pressure oscillates between systolic and diastolic pressure After the aortic valve closes, a secondary wave can be seen ▪ The division between these two waves is known as the dicrotic notch Aortic pressure curve The dicrotic notch is likely the most accurate marker of aortic valve closure ▪ The secondary wave is thought to be formed by the elastic recoil of the aorta against a closed aortic valve ▪ Likely also partially impacted by complex vibrations due to the “weird” shape of the aorta Phonocardiogram S3 – often found in healthy young adults and children ▪ New emergence is usually pathological in adults (often indicator of myocardial ischemia) ▪ Blood enters a non- compliant or “not-fully- relaxed” ventricle during rapid filling “Kentucky” (ee is S3) S4 – usually pathologic ▪ Ventricle “straining” as the atria contract and “force” blood into a non-compliant ventricle “Tennessee” (Tenn is S4) How about events in the right side of the heart? Almost identical Wiggers diagrams Note the lower pressures that develop in the right ventricle and pulmonary arteries Pulmonary artery pressure is ~ 25/7 mm Hg Slightly lower atrial pressures in the right vs. left Summary of normal pressures within the cardiac chambers and great vessels Higher of the two pressure values in the right ventricle (RV) and left ventricle (LV) represent the normal peak pressures during ejection Lower pressure values in ventricles represent normal end-diastolic pressures Pressures in the right atrium (RA) and left atrium (LA) represent values at the end of ventricular filling ▪ Just as atrial contraction is ending Arterial pressures are systolic on diastolic Cardiac calculations and parameters End diastolic volume (EDV) – the volume in the ventricle at the end of diastole End systolic volume (ESV) – the volume in the ventricle at the end of systole ▪ Look at the Wiggers diagram – what is the approximate volume of each? Stroke volume – the volume ejected with each heartbeat ▪ SV = EDV – ESV Cardiac output is the volume ejected by each systole X heart rate ▪ CO = SV X HR Ejection fraction is the proportion of EDV that is ejected each beat ▪ EF = SV/EDV = (EDV – ESV)/EDV Cardiac calculations and parameters EDV corresponds to preload ▪ Optimal preload → greater force of contraction due to optimal overlap of actin and myosin in sarcomeres Stroke volume is impacted by three major parameters: ▪ Preload ▪ Contractility (same as inotropy) – dependent on calcium handling within the cardiomyocyte – intrinsic ability ▪ Afterload - The pressure that the heart must overcome to eject blood into the great arteries. Factors that increase afterload – aortic stenosis, elevated blood pressure Cardiac output is one of the major factors that determines delivery of oxygen and nutrients to tissues ▪ Other major factor → vascular tone in the tissue receiving blood Ejection fraction is often measured as an “estimate” of heart function in heart failure ▪ More in heart failure lecture The Pressure-Volume Loop of the Ventricle Useful to measure a number of parameters: ▪ Total workload of the heart (ventricle) ▪ Contractility ▪ Compliance of the heart itself ▪ Basic hemodynamic parameters EDV ESV SV Systolic pressure curve – with a given volume, pressure generated during systole is recorded Diastolic pressure curve – The pressure is recorded during diastole for the specified volume The Ventricular Pressure-Volume Loop How do you read this thing? 1. Start from point A – this is where the ventricle is at its lowest volume and is just starting to be filled 2. As it fills (in a relaxed state) the pressure increases somewhat towards point B ▪ Even though it’s not contracting, the hydrostatic pressure of the blood within it exerts force against the wall 3. From B → C, the ventricle contracts, but no change in volume ▪ What do we call that? 4. From C → D, the ventricle ejects blood… and its volume falls 5. From D → A, the ventricle relaxes, but does not yet fill ▪ What do we call that? The Ventricular Pressure-Volume Loop How do you read this thing? Make sure you read it counter-clockwise ▪ If you go the other direction, you will get confused Stroke volume is the distance between line AD and CB Preload is point B (also known as EDV) The inotropy (contractility) is represented by the tangent line at close to point D ▪ This is known as the ESPVR – the end- systolic pressure-volume relationship ▪ The more vertical it is, the higher the inotropic state Afterload is represented by the purple line ▪ The more vertical it is, the higher the afterload The total area within the curve is the cardiac workload The Ventricular Pressure-Volume Loop A word about workload Hearts that “work too hard for too long” endure histologic and molecular changes that will be discussed in our heart failure lecture ▪ Changes save energy, but often compromise force of contraction The majority of work done by the ventricle is pressure-volume work ▪ Indicated by the area within the P-V loop The minority of cardiac work is due to actually ejecting blood from the ventricle into the artery (kinetic energy) The Pressure- Volume Loop Here are some diagrams of the cardiac physiology changes that occur as you change the major determinants of stroke volume: ▪ Contractility ▪ Afterload ▪ Preload The next slides will illustrate these with more physiologic examples Pressure-Volume loop – Increased Afterload Note the higher pressures that accompany increases in afterload ▪ Increasing afterload decreases stroke volume and ejection fraction ▪ Increasing the afterload in the heart tends to increase the myocardial oxygen demand more than increasing inotropy or overall force of contraction… Hypertension is energetically expensive Pressure-Volume loop – A word about afterload Afterload is technically determined NOT by the systolic blood pressure, but by the tension that the ventricle needs to develop to open the aortic valve Remember Laplace’s law? ▪ Wall stress = ▪ P = pressure ▪ r = radius ▪ h = wall thickness 𝑷 ∙𝒓 What happens to wall tension as the 𝝈= ventricle enlarges in radius? In thickness? 𝒉 Pressure-Volume loop – Increased Preload Note the higher end diastolic volume and resulting greater stroke volume ▪ The heart is an elegant machine with elegant fail- safes ▪ If the heart’s strength of contraction decreases, there’s “more left” at the end of diastole… ▪ This leads to increased preload → ▪ Increased stroke volume Pressure-Volume loop – Increased Contractility Note the lower stroke volume as inotropy decreases ▪ As it decreases, then the amount left at the end of systole also decreases… Representing a decrease in ejection fraction ▪ A normal ejection fraction is > 50% What is the ejection fraction for each one of these curves? Pressure Volume Loops The previous changes in the P-V loops that accompanied changes in preload, afterload, and contractility were done in “non-physiologic” situations ▪ Isolated heart preparations, outside of the organism The P-V loops below are examples found in intact hearts, showing the interdependence of the factors that impact stroke volume Pressure Volume Loops Curve A – increased venous return → increased preload → increased stroke volume… but also a slight increase in afterload as the wall tension increases (increase in pressure) Curve B – afterload increases → decreased stroke volume → increased preload → a new “steady state” with slightly elevated preload but a greater increase in afterload Curve C – inotropy increases → increased stroke volume → decreased volume left after systole → a slightly lower preload Modifiers of cardiac output How do the following affect cardiac output? ▪ Catecholamines? ▪ Parasympathetic stimulation? ▪ Stretching of the ventricles? ▪ Isolated increases in heart rate (chronotropic and inotropic)? ▪ Increases or decreases in central blood volume? ▪ Increases or decreases in systolic blood pressure? Recall the equation for cardiac output as you work on this Additional FYI points: ▪ Fever increases cardiac output – for every degree, heart rate increases approximately 10 beats/min ▪ As you stretch the right atrium, a reflex is initiated (Bainbridge reflex) that activates the cardiac sympathetic nervous system Sympathetic Nervous System – impact on cardiac output Treppe effect = accumulation of calcium in the SR as HR increases Not enough time to “remove” calcium from the cell across the plasmalemma Modifiers of cardiac output … and back to preload Preload and venous return to the heart (closely related) may be the most determinant of cardiac output ▪ As the heart delivers more blood to peripheral tissues, then venous return increases ▪ Force of contraction will also increase as preload increases ▪ This will eventually be limited by the increase in afterload that occurs as blood pressure rises Use the concept of interdependence of preload and venous return to explain why the cardiac output of the right and left ventricle must be equal Question Which ventricle pumps more blood per minute? ▪ Right or left? Think about: the pressure that both ventricles develop, the thickness of the walls, vascular contractions Cardiac output must be equal between the left and right ventricles for multiple reasons: ▪ Proper tissue perfusion & oxygen delivery ▪ Preventing pulmonary congestion and systemic hypoperfusion PRELOAD OF ONE VENTRICLE DEPENDS ON THE CARDIAC OUTPUT OF THE OTHER.