Biophysics Lectures PDF
Document Details
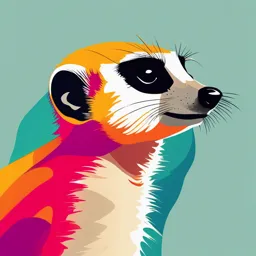
Uploaded by SnappyPolonium6292
سمارت الدولية
Tags
Summary
These lecture notes cover biophysics topics, including cell characteristics, eukaryotic and prokaryotic cell types, plasma membrane, membrane proteins, transport across the plasma membrane, active transport mechanisms, and signal transduction.
Full Transcript
🧬 Biophysics: Lectures Lecture 1: “The Cell” Characteristics of All Cells: 1. A surrounding Membrane 2. Protoplasm “thick fluid that contains the Cell” 3. Organelles 4. Control Center With DNA Ce...
🧬 Biophysics: Lectures Lecture 1: “The Cell” Characteristics of All Cells: 1. A surrounding Membrane 2. Protoplasm “thick fluid that contains the Cell” 3. Organelles 4. Control Center With DNA Cell Types 1. Prokaryotic 2. Eukaryotic Eukaryotic cells: It’s a nucleus bound by a membrane that possesses many organelles Example: Fungi, Protists, Plant and Animal Cells Biophysics: Lectures 1 Ordinary Animal Cell Plasma Membrane “Phospholipid Bilayer” It Contains Cell Contents It’s a Double Layer of Phospholipid Bilayer Phospholipids Biophysics: Lectures 2 Polar Hydrophilic Head بيحب المياة Hydrophobic Tail بيكره المياة Interacts with water Membrane Proteins 1. Channels Or Transporters (Move Molecules in one direction) “One Way Bridge” Example: Leak Channels 2. Receptors (Recognize certain chemicals) “Lock and Key” Example: Ligand Gated Channels 3. Glycoproteins (Identify Cell types) “Cell Fingerprint” 4. Enzymes (Catalyze Production Of Substances) “”بوتوجاز Cytoplasm Interconnected Filaments & Fibers Organelles Storage substances Viscous fluid containing Organelles ؟organelles طب من غير ال Cytosol يبقى اسمه Nuclear Envelope Separates Nucleus From Rest of Cell Is Porous “Has Pores” Double Membrane “10-50 nm apart” The outer membrane is continuous with the Rough Endoplasmic Reticulum DNA Biophysics: Lectures 3 Hereditary Material Chromosomes are originated from DNA Nucleolus Most cells Have 2 or more, has direct synthesis with RNA “Single Strand of DNA”, forms ribosomes Endoplasmic Reticulum Helps move substances within cells Network of Interconnected membranes There are two types of endoplasmic reticulum 1. Rough Endoplasmic Reticulum 2. Smooth Endoplasmic Reticulum Rough Endoplasmic Reticulum “RER” Ribosomes attached to Surface Manufactures Proteins Not all ribosomes attached to RER Smooth Endoplasmic Reticulum “SER” No attached ribosomes Has enzymes that help build Carbohydrates & Lipids Golgi Apparatus “Amazon” involved in synthesis of plant cell wall Packaging & Shipping Station of the cell Responsible for making vesicles that are used in Endocytosis & Exocytosis Lysosomes “”بيليث الدنيا Biophysics: Lectures 4 Contain Digestive Enzymes Functions: 1. Aid in Cell Renewal 2. Break down Old Cell parts 3. Digests Invaders Mitochondria “Power house of the cell” Generates ATP that is used by the cell as a source of chemical energy Have Their own DNA Bound by Double Membrane Used in Signaling, Cellular Differentiation, Control Of Cell Cycle and Cell Growth Breaks Down Fuel Molecules “Glucose, Fatty Acids” and Releases ATP in the form of energy Lecture 2: “Plasma Membrane, Membrane Proteins & Transport Across the Plasma Membrane” 1. Cell Membrane Basics Must be flexible to avoid breaking Also known as: plasma membrane or phospholipid bilayer Forms boundaries between cell and surroundings (protective function) Responsible for bioelectricity through ion movement Organizes chemical activities Facilitates communication (signaling) between cells Controls trafficking of molecules in and out of the cell Biophysics: Lectures 5 Exhibits selective permeability 2. Structure of Plasma Membrane Phospholipid arrangement: hydrophilic heads, hydrophobic tails Arrangement: heads-tail-tail-heads Fluid Mosaic Model of PM A membrane is a mosaic of components Proteins and other molecules are embedded in a framework “ ”نطاقof phospholipids Most protein and phospholipid molecules can move laterally “”بالعرض Structure of the phospholipid tail: (بتاعت الدكتور عشان ماتتزاولوشpdf لا انا مش بستنتن في الصور انا بس بوريكوا الصور )بتاعت ال Structure of the membrane: Biophysics: Lectures 6 4. Membrane Proteins 4.1 Integrins Integrated proteins in the phospholipid bilayer Can be peripheral or integral Strengthen the membrane 4.2 Integral Proteins Transfer substances in and out of the cell 4.3 Glycoproteins Carbohydrate chains on the cell surface attached to a protein Act as recognition modules for the cell (cellular "fingerprint") Glycoproteins pass through the membrane transversely 💡Fun fact: COVID-19 was recognized by its glycoprotein in vaccine development Biophysics: Lectures 7 Intercellular Junction proteins help like cells stick together to form tissues and to communicate. Intercellular junction proteins form channels that allow small molecules to pass between the adjacent cells. Subunits of these channels are connexins that are assembled together to make connexons. The connexons from 2 cells join together to make a gap junction. Connexins are a family of structurally related transmembrane proteins that assemble to form gap junctions 4.4 Gap Junctions Adjacent cells each contribute a hemichannel Full channel = gap junction Each channel consists of 12 units Each hemichannel consists of 6 units Simple diffusion Simple diffusion is the movement of molecules from an area of high concentration to an area of low concentration without the need for energy or carrier proteins. The concentration gradient provides the driving force for this movement. Biophysics: Lectures 8 💡 Nonpolar, hydrophobic molecules diffuse directly through the phospholipid bilayer because they are hydrophobic meaning they can dissolve in the phospholipid bilayer “membrane” and the phospholipid bilayer is made of non polar hydrophobic molecules therefore its naturally compatible with nonpolar molecules Simple diffusion does not require the use of transport proteins. Examples: O2, CO2, steroids Polar, hydrophilic substances cannot pass directly through the lipid bilayer , they’ll need specific transport proteins (sodium, potassium or Na/K pump) Examples: water, ions, carbohydrates 💡 the concentration gradient is the primary factor controlling simple diffusion. Facilitated diffusion 💡 In facilitated diffusion small polar molecules and ions diffuse through passive transport proteins. No energy needed Most passive transport proteins are solute specific Example: glucose enter/leaves cells through facilitated diffusion Osmosis Biophysics: Lectures 9 Osmosis – diffusion of water across a selectively permeable membrane Water moves from an area of higher water concentration to an area of lower water conc. Water travels in/out of the cell through aquaporins (integral membrane proteins that forms pores in the biological membranes) Hypertonic lsolution with a relatively high concentration of solute Hypotonic lsolution with a relatively low concentration of solute Isotonic lsolutions with the same solute concentration Cells placed in distilled water swell and then burst but the cells placed in concentrated salt solution shrivel ()بتنكمش 4.5 Signal Transduction Proteins bind hormones and other substances on the outside of the cell. Binding triggers a change inside the cell Called signal transduction Example: The binding of insulin to insulin receptors causes the cell to put glucose transport proteins into the membrane. Receive and transfer signals without cell translocation Biophysics: Lectures 10 The yellow semi triangle shapes are the Messenger proteins and the purple semi spheres are the Receptors 5. Transport Across the Membrane 5.1 Active Transport Proteins Require energy 5.2 Passive Transport Proteins Do not Require energy Types: simple diffusion, facilitated diffusion, osmosis 💡Important: Ions cannot pass directly through the phospholipid bilayer Lecture 3: “Active Transport” Biophysics: Lectures 11 1. Definition and Basics A- Bulk transport: It is a mode of active transport by which large molecules or large amount of small particles are transported across the plasma membrane. Vesicles are used to transport large particles across the plasma membrane “Vesicle containing cells” Transport against concentration gradient (low to high concentration) Requires energy from ATP (adenosine triphosphate) ATP hydrolysis releases energy 2. Protein Phosphorylation Molecule gains phosphate: becomes "phosphorylated" Molecule loses phosphate: becomes "dephosphorylated" 3. Protein Conformation Conformation: spatial arrangement of protein Changes in conformation affect protein function Structure “elements” remains the same, but active sites may change 4. Types of Active Transport 4.1 Bulk Transport Exocytosis (outwards): The type of bulk transport by which materials are transported from the inside to the outside of the cell in membrane-bound Biophysics: Lectures 12 vesicles that fuse with the plasma membrane. in this picture: we see the vesicle containing cells getting expelled out of the membrane (purpose of it not being rigid) exploding the vesicle out of the membrane, Cytoplasmic vesicle merges with the Plasma membrane and releases its contents Examples? Golgi body vesicles merge with the PM and release their contents How neurons release neurotransmitters Endocytosis (inwards): The mode of transport in which particles are transferred into a cell by enclosing them in a vesicle made out of plasma membrane. Biophysics: Lectures 13 in this picture: we see cells getting engulfed in the membrane (purpose of it not being rigid) then forming a cytoplasmic vesicle inside the membrane Phagocytosis: “solid substances” Membrane sinks in and captures solid particles for transport into the cell Examples: Solid particles often include: bacteria, cell or food Pinocytosis “liquid substances” Same thing as Phagocytosis but instead of solid substances it’s liquid Notes: Phagocytosis and pinocytosis are not selective ”Membrane sinks inward and captures whatever particles/fluid present.” Receptor-mediated endocytosis: specific cell uptake 1. Receptor proteins on PM bind specific substances (vitamins, hormones..) 2. Membrane sinks in and forms a pit – Called a coated pit 3. Pit gets closed to form a vesicle around bound substances Cytoskeleton aids in pulling in the membrane and vesicle formation Biophysics: Lectures 14 4.2 Ion and Small Molecule Transport Specific ions and molecules through channels 5. ATP Function ATP + H2O → ADP + Pi + free energy Energy release drives active transport 6. Sodium-Potassium Pump (Na+/K+ ATPase) A- Function: The Sodium-Potassium Pump The mechanism responsible for the transport of Potassium and Sodium into and out of the cell Sodium-potassium pump (Na+/K+-pump ), is a protein that has been identified in many cells. It is an enzyme that is also called Na+/K+-ATPase (sodium potassium adenosine triphosphatase) Biophysics: Lectures 15 It maintains the internal concentration of potassium ions [K+] higher than that in the surrounding medium (blood, body fluid, water) and the internal concentration of sodium ions [Na+] lower than that of the surrounding medium using the power of ATP. IMPORTANT: For each ATP broken down, The pump moves 3 sodium ions out and 2 potassium ions in against their concentration gradient Transfers Na+ and K+ against concentration gradients Process: 1. Na+ binding (high affinity) 2. Protein phosphorylation 3. Conformation change 4. Na+ release, K+ binding (high affinity) 5. Dephosphorylation 6. Return to original state 7. Types of Ion Channels Leak channels: always open Gated channels: open and close via stimuli Voltage-gated: opens/closes with voltage changes Ligand-gated: opens when ligand attaches Mechanically-gated: responds to mechanical stimuli Note: Gated channels facilitate passive transport in a controlled manner, following concentration gradients. Biophysics: Lectures 16 Lecture 4 summary: “Generation of membrane potential” Cations: positively charged ions (K+, Na+, Ca2+). “ Cat”ions are “paw”sitive. Anions: negatively charged ions (Cl-, proteins). Electrical gradient: difference in electrical charge across a membrane. Chemical (concentration) gradient: difference in ion concentration across a membrane. General rules: Movement of ions across the cell membrane 1. Ions move from higher to lower concentration. 2. Ions attract opposite charges and repel like charges. 3. Cell membrane permeability to ions determines their movement and depends on the number of leak channels. The Actual Lesson: Membrane Potential or Membrane voltage is the electrical potential generated as a result of unequal distribution of ions (charges) across the plasma membrane. Unequal ion distribution across the membrane creates a charge imbalance (more negative inside, more positive outside). Net charge is determined by the predominant ion type. “Here: we see the net charge inside is negative and outside is positive” Biophysics: Lectures 17 Membrane voltage results from unequal ion distribution across cell membranes. Most cells have a negative resting membrane potential of -70 mV (in the absence of stimuli). The negative sign of the membrane potential means that the cell has more negative charge in the inside of the cell The membrane potential is determined by: 1. concentration gradients of ions across the membrane 2. membrane permeability to each type of ion. The biggest factor to membrane potential is largely by Na “Mostly outside of the cell” and K “Mostly inside of the cell” since they come with high concentration Biophysics: Lectures 18 Cell membranes contain many constantly open ion channels called "leak channels" refer back to Lesson 3 Ions have to use integral proteins that provide a hydrophilic tunnel across the membrane forming ion channels in order to move Our focus is on sodium (Na+) and potassium (K+) channels. Potassium tends to leak out of the cell using the leak channels Proteins, being large molecules, remain trapped inside the cell. Cells maintain a high potassium concentration “due to their concentration gradient” inside and high sodium concentration outside. The anions inside the cells have tendency to follow the cations. The predominant anions inside the cells are the large negatively charged proteins which are too large to diffuse out. Potassium leak channels are 60 times more numerous than sodium leak channels therefore Potassium outflow significantly exceeds sodium intake. This ion movement disturbs the concentration gradient, which must be maintained at specific levels. Consequently, a net positive charge accumulates outside the cell, and a net negative charge inside. Key terms: Resting state: +70 mV outside, -70 mV inside Hyperpolarized: +90 mV outside, -90 mV inside Depolarized: +20 mV outside, -20 mV inside Biophysics: Lectures 19 Depolarization occurs when the potential becomes more positive than the resting potential. Hyperpolarization occurs when it becomes more negative than the resting potential. Cells actively maintain ion balance. The sodium-potassium pump expels 3 sodium ions for every 2 potassium ions it brings in. Protein deficiencies , can disrupt both concentration gradients and membrane potential. Resting Membrane Potential (RMP): The cell's electrical state at rest, without stimuli. Stimuli can alter this membrane potential. When more than one ion channel is present and open in the plasma membrane, the membrane potential can be calculated by using the Goldman-Hodgkin-Katz equation (GHK equation). Calculations of RMP: The Goldman- Hodgkin-Katz “GHK” equation: OOI / IIO Em: Membrane potential. R: Universal gas constant (8.314 J·K⁻¹·mol⁻¹). T: Temperature in Kelvin (K = °C + 273.15). F: Faraday's constant (96485 C·mol⁻¹). Z: = valence (charge) of ion Biophysics: Lectures 20 Pₖ: Membrane permeability for K⁺. Pₙₐ: Relative membrane permeability for Na⁺. PCl: Relative membrane permeability for Cl⁻. I: Inside of the cell. O: Outside of the cell. Permeability factor: Permeability is multiplied by the concentration of ions. For a typical neuron at rest, the relative permeability ratio is: K⁺: Na⁺: Cl⁻ = 1: 0.05: 0.45. Permeability factor is multiplied with concentration of the ions Permeability factor: Typical neuron at rest K: Na: Cl = 1:0.05:0.45 The Permeability Factor Permeability refers to the ease at which ions cross the membrane. It is directly proportional to the total number of open channels for a given ion in the membrane. If many K+ channels are open, PK will be high. If only a few K+ channels are open, PK will be small. The permeability values are reported as relative permeabilities with PK having the reference value of One (because in most cells at rest PK is larger than PNa and PCl) Leak Channels: In a cell with no leak channels, the permeability values for K⁺, Na⁺, and Cl⁻ would all be zero (0: 0: 0). As a result, no membrane potential would exist. 💡 Permeability value is unitless Lecture 5 summary “Nernst Potential” : 1.Factors defining the movement of ions across the cell membrane: Biophysics: Lectures 21 Concentration gradient: ions move from areas of higher concentration to lower concentration Electrical gradients: ions move away from like charges and toward opposite charges Number of ion channels: the permeability of the cell membrane to the ions define their movements across the cell membrane The equilibrium potential, or Nernst potential “Measured in Millivolts (mV)” Definition: It’s the voltage which would balance out the unequal concentration across the membrane for that ion type It’s the voltage across a cell membrane where there is no net movement of a specific ion, like sodium or potassium, across the membrane Calculates the voltage needed to cancel out the net movement of an ion in its concentration gradient “normal state of the cell” Different States Of The Cell Observation Voltage States - 70 mV Outside: All positive ions span all of the outside part of the membrane and → -10 mv +ve the negative ions span all of the inside part of the membrane ,when slowly going from negative to positive voltage, the ions slowly starts Biophysics: Lectures 22 Observation Voltage States Inside: - losing its ions one by one so the positive and negative side would ve slowly start decreasing in number one by one until it vanishes at 0 mV Outside: None 0 mv No side would favor any charge whether inside or outside Inside: None The normal state of the cell would be flipped, so that the positive Outside: +10 mV ions are on the inside and the negative ions are on the outside and it -ve → +70 will slowly start filling up, when it reaches +90 mv the outside part Inside: mV will be completely filled with negative ions and the inside part will be +ve completely filled with positive ions “Cell when it has a voltage of -70 mV” Positive ions are on the outside and the negative ions are on the inside Biophysics: Lectures 23 “Cell when it has a voltage of 0 mV” no ions take place here “cell when it has a voltage of +70 mV” positive ions are on the inside and negative ions are on the outside Biophysics: Lectures 24 Considering Sodium ions: cell بتاعت الحركة بتاعته بتقل ناحية جوة الintensity كل ما بتتجه ناحية الزيرو كل ما ال هتعمل ايه بقى لو انا قولتلك اني عكست البوتنشال؟ If the membrane potential is reversed, The electrical gradient is now acting against the concentration gradient trying to stop the inflow of the Na+ ions. When the electrical gradient becomes in balance with the concentration gradient, it will stop the inflow of the Na+ ions طب لو انا جيت قولتلك اني زودته اكتر من الرينج اللى كنا كاتبينه؟ Biophysics: Lectures 25 و بالتالي حركة الصوديوم هتتعكسelectrical gradientهيبقى اضعف من ال concentration gradient وقتها ال potassium طب يا اخي نيجي لل عليت يبقى التدفق بتاع ايونات البوتاسيوم هيقلnegativity لو ال If the membrane potential is changed (the negativity increased), The electrical gradient will counterbalance the concentration gradient and stops the flow of the K+ ions. طب لو علي؟ If the membrane potential is changed further, The electrical gradient will overcome the concentration gradient and reverse the direction of flow of the K+ ions. The Equilibrium Potential (The Nernst Potential) For a specific ion, the electrical potential difference that exactly counterbalances diffusion due to the concentration gradient is called the equilibrium potential for that specific ion. For a channel that is permeable to a single ion, the equilibrium potential is also called the reversal potential and it is defined the same as the equilibrium potential. The equilibrium potential of a specific ion can be calculated by the Nernst equation. Calculation of equilibrium potential: Biophysics: Lectures 26 R = gas constant (8.314 J/K.mol) F = Faraday constant (95,484.56 C/mol) T = temperature (K) (K= OC + 273.15) Z = valence (charge) of ion Potassium: Biophysics: Lectures 27 R = gas constant (8.314 J/K.mol) F = Faraday constant (95,484.56 C/mol) K+: z = +1 T = 37 OC = 310.15 K [K+]out = 5 mM [K+]in = 150 mM EK = - 0.091 V = -91 mV EK ~ -90 mV Sodium: Na+: z = +1 T = 37 OC = 310.15 K [Na+]out = 150 mM [Na+]in = 15 mM Biophysics: Lectures 28 ENa = 0.062 V = 62 mV Chlorine Cl-: z = -1 T = 37 OC = 310.15 K [Cl-]out = 120 mM [Cl-]in = 10 mM Eeq.Cl = - 0.0669 V = 66.9 V Eeq.Cl ~ -67 mV An Important Rule: “When the membrane conductance increases for a particular ion, the membrane potential will move toward the Nernst potential for that ion.” Electrochemical driving force of an ion: The driving force is the net electromotive force that acts on the ion. Biophysics: Lectures 29 The magnitude of the driving force indicates how far the membrane potential (Vm) is from the electrochemical equilibrium (Eeq.) of an ion. Thus, the magnitude of the driving force indicates how far an ion is from its equilibrium When an ion is not at its equilibrium, electrochemical driving force (DF) acts on the ion causing the net movement of the ion across the membrane down its electrochemical gradient. Electrochemical Driving force The total forces acting upon ions across a membrane is a combination of both chemical and electrical forces and is referred to as the electrochemical driving force if the chemical and electrical forces are in the same direction, no analysis is needed equilibrium potential > membrane potential ; chemical force is larger and electrochemical driving force is in the chemical force’s direction equilibrium potential < membrane potential ; electrical force is larger and electrochemical driving force is in the electrical force’s direction DF = Em − Eeq.ion E.eqNa = +62mV E.eqK = −90mV In Case of Na Ions if the result of the driving force is -ve, flow of Na ions will be into the cell Example: DF = −70 − (+62) = −132mV Na Direction: Into the Cell if the result of the driving force is +ve, flow of Na ions will b reversed and go out of the cell Example: DF = +80 − (+62) = +18mV Na Direction: Out of the cell In Case Of K Ions if the result of the driving force is -ve, flow of K ions will be into the cell Example: DF = −70 − (−90) = +20mV K Direction: Into the cell Biophysics: Lectures 30 if the result of the driving force is +ve, flow of K ions will be reversed and go out of the cell Example: DF = −100 − (−90) = +10mV K Direction: Out of the cell In Case Of Na & K Ions If the Membrane Potential “MP” is at +62 mV “ E.eqNaOf sodium” , Then There will be High Driving Force for K and flow of K ions, and No Driving Force or net flow of Na If the Membrane Potential “MP” is at -90 mV “ E.eqK Of Potassium” , Then There will be High Driving Force for Na and flow of Na ions, and No Driving Force or net flow of K Lecture 6 summary “Action Potential” Ion Channels Revisited Ion channels are transmembrane proteins responsible for transporting ions across cell membranes. They are categorized into two types: gated channels and open channels. Gated Channels: These channels open and close in response to specific stimuli. Types include: Voltage-gated ion channels Ligand-gated ion channels Ligand-Gated Channels Ligand-gated channels are a class of transmembrane proteins that open when a specific ligand binds to the receptor on the membrane. These channels are less selective, often allowing multiple ion types to pass through the Channel Pore. They are commonly found in electrically excitable cells, like neurons. Voltage-Gated Channels Voltage-gated channels are a class of integral proteins activated by changes in membrane potential. When the membrane undergoes depolarization or Biophysics: Lectures 31 repolarization, these channels permit the flow of only one type of ion, essential for processes like signal transmission, muscle contraction, neurotransmitter release, and hormone secretion. Mechanism Action (States of Voltage Gated Channels:) 1- Closed State “Resting State”: It’s the state that’s before any change in the membrane potential 2- Open State: The State That’s Activated when there’s a change in the membrane potential , the channel allows ion transfer in and out of the cell 3- Inactivation State: When the membrane potential is restored to it’s origin, The Channel gets Physically blocked with the Ball and Chain Mechanism and it stays that way until a change in protein conformation to go back to being in a closed state “resting state” after a while then the ball and chain’s lock is released. Why is this even a thing? To control direction of signal propagation Biophysics: Lectures 32 Closed State: The channel is initially closed with no ions flowing through, waiting for a change in membrane potential Open State: When the membrane potential changes (depolarization), the channel opens, allowing ions to flow in and out of the cell. Inactivated State: After a short period, even if the stimulus is still present, the channel temporarily stops ion flow by closing through the ball and chain mechanism This cycle helps control the direction and flow of electrical signals in the cell. When channels close after activation, they prevent the signal from going backward, which allows for a one-way propagation of the action potential along the neuron. Action Potential An action potential is a rapid rise and subsequent fall in membrane potential across a cell membrane, following a characteristic pattern. In order for the Action Potential to happen , it must pass the threshold “-55 mV” otherwise the action potential wont happen and it will be considered as a “Failed Initiation” Action Potential obeys the “All or None” Law, when the stimulus is more than or equal the threshold Amplitude , Duration , Shape Do Not Depend on the power of the stimulus Action Potentials travel along the cell membrane without losing their characteristics. Biophysics: Lectures 33 Action Potential have refractory periods Action Potential Stages: 1. Resting State 2. Depolarization State 3. Repolarization State 4. Hyperpolarization State 1-Resting State: The state before any change in membrane voltage The membrane is polarized because of the present -70 mV waiting for a voltage in the membrane to meet the threshold “-55 mV” to activate the Na Channels and for the action potential to fire Biophysics: Lectures 34 2- Depolarization Stage: The state in which the Membrane Voltage Goes Past the Minimum Threshold “-55 mV” therefore, making Sodium “Na” Channels Activate “Open” because of the electrical gradient inside the cell is negatively charged, attracting Positive Na Ions , Letting the Na ions go from outside the cell to inside the cell going from -55 mV to +40 mV 3- Repolarization Stage: The state in which Potassium “K” Channels open, Letting the K Ions go from inside the cell to outside because of the electrical gradient that’s now positive inside the cell, therefore repelling “Pushing” the Positive K Ions out of the cell , making the voltage drop from +40 mV to -70 mV 4- Hyperpolarization Stage: The state in which Excess “way too much” Potassium getting out of the cell because of the slow closure of the K Channels, making the cell go to -90 mV until the sodium and potassium completely switch places Returning back to Resting State Process: Biophysics: Lectures 35 Now that the sodium and potassium are completely switched “sodium more than normal inside the cell when it should be outside” “potassium more than normal outside the cell when it should be inside” so now it should return back to normal, and this is where Sodium Potassium “Na/K Pump” Comes into Play. Action Potential States “During Action” State Voltage Time Resting State < -55 mV it stays in this state until triggered Depolarization -55 mV → +40 mV 2 milliseconds Repolarization +40 mV → - 70 mV 2 milliseconds Hyperpolarization -70 mV → -80 mV or -90 mV 3.5- 4 milliseconds Refractory Periods “Caused by changes in the state of sodium and potassium channel molecules” It’s the period thats immediately after a stimulus in which other stimulus have no effect Refractory Periods are the Recovery times of an excited membrane to be ready for another stimulus once it returns to its resting state each Action potential has a refractory period, which can be divided into two types 1. Absolute Refractory Period: Impossible to start another action potential 2. Relative Refractory Period: Stronger than usual stimulus 1-Absolute Refractory Period “1-2 ms” It’s the time during which another stimulus given “no matter how strong” will not lead to another action potential After an Action Potential, Sodium Channels enter the inactivated state using the “Ball and Chain Mechanism” Relative Refractory Period Biophysics: Lectures 36 It’s the time during which another stimulus given “must be stronger than the initial one and when the sodium channels become ready from inactivation” it will generate another action potential. Lecture 7 “Generation of Nerve Signal” Definition: Neurons are nerve cells that send messages all over your body to allow you to do everything from breathing, talking, eating, walking, and thinking. Nerve Cell “Neurons” Structure: 🚧 The Nervous System Contains Millions of Neurons The nervous system is made up of millions of neurons. Each neuron has three main parts: 1. Dendrites: Bushy-like structures that receive information from other neurons. They carry these signals to the cell body. 2. Cell Body (Soma): Contains the cell nucleus. Biophysics: Lectures 37 Relays the signal from the dendrites to the axon. 3. Axon: A long, thin structure that transmits signals from the cell body to the axon terminal. Axons can be: Myelinated: Covered with Schwann cells, enabling faster signal conduction. Unmyelinated: Do not have Schwann cells, so signals travel slower. Synapse Between Two Neurons Syanpse is: The space between neurons and each other The space where neurotransmitters diffuse Biophysics: Lectures 38 First neuron releases neurotransmitter to second neuron that responds to it 1st neuron is PREsynaptic neuron 2nd neuron is POSTsynaptic neuron Neurotransmitters: are chemical substances that live in the axon terminal. Communicate with other close neurons by binding to their receptors. ⚠️ After signaling, they are either neutralized by an enzyme or taken back up by the neuron in a RE-UPTAKE process At least 50 of them were identified but the most well known are: Acetylcholine Biophysics: Lectures 39 Serotonin Dopamine Endorphins Synaptic Transmission Process The neurotransmitters are released from the vesicles and then attach to receptors located on the postsynaptic neuron. These neurotransmitters are in contact with the dendrite of the postsynaptic neuron only briefly. The chemical is almost immediately destroyed or reabsorbed. Action Potential in Neurons The neurotransmitters are released from the vesicles and then attach to receptors located on the postsynaptic neuron. Binding of the neurotransmitters to the receptors on the postsynaptic neuron allows opening ion channels that allow movement of ions in and out of the cells. (A chemical signal is inverted into an electrical signal) Resulting in changing the membrane potential If the membrane potential reaches the threshold voltage at the axon hillock, voltage-gated Na+ and K+ channels open and an action potential is initiated The action potential then propagates through the cell membrane along the axon of the neuron Lecture 8 “the mechanism of muscle contraction” Neuromuscular Junction Motor neuron: Biophysics: Lectures 40 Motor Unit is a group of muscle fibers controlled by ONE motor neuron. Small motor units are found in muscles that require fine control “