Biochem 3.2 Receptor Proteins PDF
Document Details
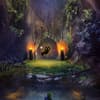
Uploaded by iiScholar
Arizona State University
Tags
Summary
This document details nonenzymatic protein activity, focusing on receptor proteins, including ligands, agonists, and antagonists. It explains how these molecules interact and influence cellular responses. Examples of signal transduction pathways are also described.
Full Transcript
Chapter 3: Nonenzymatic Protein Activity103Lesson 3.2Receptor ProteinsIntroductionCells must constantly monitor and adapt to the conditions of their surroundings. For example, muscle cells typically respond to high levels of extracellular glucose by bringing glucose into the cell and either storing...
Chapter 3: Nonenzymatic Protein Activity103Lesson 3.2Receptor ProteinsIntroductionCells must constantly monitor and adapt to the conditions of their surroundings. For example, muscle cells typically respond to high levels of extracellular glucose by bringing glucose into the cell and either storing it or breaking it down for energy. In contrast, under low-glucose conditions, cells break down previously stored glucose or begin to use other sources (eg, fat stores) for energy. Many other extracellular conditions (ie, extracellular signals) cause a variety of intracellular responses. Each response is mediated by one or more receptor proteins.A receptor protein is any protein that receives an extracellular signal and, as a result, induces an intracellular response. These extracellular signals may be chemicals that bind the receptor protein (ie, ligands), light that induces a chemical reaction in the receptor, electrical impulses that alter a protein\'s optimal conformation, or mechanical pressure that distorts the receptor\'s shape. This lesson focuses primarily on chemical signals, although other signals produce similar responses.3.2.01 Ligand Binding by Receptor ProteinsAlthough receptor proteins respond to a variety of signals, the most common signal is the binding of a ligand. Ligands that bind receptor proteins and induce the primary biological response are called agonists. In general, receptor proteins are highly specific to their agonist, although similar molecules that bind and induce a response may be synthesized. Interactions between natural or synthetic agonists and a receptor protein are shown in Figure 3.11. Figure 3.11 Agonist interactions with a receptor induce a cell response. Chapter 3: Nonenzymatic Protein Activity104Like any protein-ligand interaction, the strength with which an agonist binds its receptor can be measured by the. An agonist that binds its receptor with a small Kd value (ie, tight binding) can cause a significant response when only a small amount of agonist is present. Agonists that bind with large Kd values must be present in larger quantities to produce a significant response.Receptor protein activity can be regulated or altered by another type of ligand called an antagonist, which impedes agonist binding (see Figure 3.12). Importantly, binding by an antagonist does not cause the same biological response as binding by an agonist.Figure 3.12 Agonists bind to receptors but do not cause the normal biological response.Antagonists may bind at the same binding site as agonists. In Figure 3.12, the antagonist directly competes with the agonist for binding---when the antagonist is bound, the agonist is physically blocked from binding the receptor, and vice versa. Consequently, adding more agonist can displace the antagonist and reverse its effects.Alternatively, antagonists may bind receptors at an. This may either interfere with agonist binding or with the ability of bound agonist to transmit the signal, or both. Additional agonist does not necessarily displace allosteric antagonists.Note that allosteric antagonist binding may impede agonist binding without completely abolishing it. In other words, rather than fully blocking agonist binding, an antagonist may merely reduce the affinity of the receptor for its agonist or reduce the intensity of a receptor response. Chapter 3: Nonenzymatic Protein Activity105Concept Check 3.7A scientist measures a certain cellular response in cells cultured with different levels of Compound 1 and Compound 2, each of which binds the same receptor protein. The results are shown in the following graph. Based on the graph, identify Compounds 1 and 2 as an agonist or an antagonist, and determine whether the antagonist most likely binds at the primary binding site or an allosteric site.3.2.02 Transmembrane ReceptorsMany extracellular signals are hydrophilic molecules, which cannot easily cross the hydrophobic interior of the membrane. These signals are received by transmembrane receptor proteins (see Figure 3.13). The extracellular domain of the receptor binds the signal (ie, the agonist), which induces a conformational change that extends to the intracellular domain.The change in shape causes the intracellular domain to interact differently with nearby molecules. For instance, the intracellular domain may bind or release certain ligands or may gain or lose catalytic activity in response to an agonist binding the extracellular domain. Regardless of the details, the intracellular domain eventually modifies the behavior of an intracellular molecule and activates one or more small molecules called second messengers. The second messengers then continue the pathway toward the biological response. Chapter 3: Nonenzymatic Protein Activity106Figure 3.13 Schematic of an agonist binding a receptor and activating a second messenger.One of the most abundant and best characterized classes of receptor protein is the G protein--coupled receptor (GPCR). GPCRs have seven transmembrane (7-TM) regions and interact with a class of proteins called G proteins. In the absence of agonist-GPCR interactions, the G protein binds guanosine diphosphate (GDP) and is inactive. Upon agonist binding, the GPCR intracellular domain undergoes a conformational change that affects the G protein\'s affinities toward guanosine nucleotides.As a result of this change, the G protein then releases its GDP molecule and in its place binds guanosine triphosphate (GTP). The G proteins consist of three different subunits (α, β, and γ), making them heterotrimers. When bound to GTP, the α subunit dissociates from the other subunits and gains the ability to interact with an enzyme (ie, the G protein is activated). This interaction alters the activity of the enzyme, ultimately leading to various physiological outcomes. Eventually, the α subunit converts GTP into GDP, reverting itself to its inactive form. A typical GPCR mechanism is shown in Figure 3.14. Chapter 3: Nonenzymatic Protein Activity107Figure 3.14 General mechanism of GPCR action.The α subunit may bind different proteins and have , although one of the most common effects is activation of adenylyl cyclase. This enzyme produces cyclic AMP, which acts as a second messenger in many signaling pathways.GPCRs and other receptors often cause signal cascades such as the MAP kinase pathway. In this pathway, agonist binding to a receptor protein leads to phosphorylation of an intracellular protein. This protein then becomes capable of phosphorylating other proteins, which become capable of phosphorylating still more proteins. As a result, a single agonist binding its receptor can cause the phosphorylation of thousands of proteins, yielding a large cellular response. This phenomenon is called signal amplification (see Figure 3.15). Chapter 3: Nonenzymatic Protein Activity108Figure 3.15 Signal cascades amplify the effect of the initial signal.The result of a signal cascade often involves direct activation or inhibition of existing proteins, or upregulation or downregulation of gene expression. For example, insulin binding to its receptor on a liver cell leads to dephosphorylation of the enzyme phosphofructokinase-2, which activates it and increases glucose consumption.Simultaneously, insulin binding causes modifications that reduce synthesis of the enzyme phosphoenolpyruvate carboxykinase (PEPCK). Decreased PEPCK leads to decreased glucose production. Chapter 3: Nonenzymatic Protein Activity109Concept Check 3.8Based on the given diagram, match the following molecules with their role in the signaling pathwayA.DAG + IP31.AgonistB.Fz2.GPCRC.Wnt3.Second Messenger3.2.03 Cytosolic ReceptorsSome extracellular signals are hydrophobic molecules (eg, , see Lesson 9.3). Because of their hydrophobicity, these molecules dissolve poorly in water. As such, they require carrier proteins to transport them through the bloodstream or other aqueous environments. Once these hydrophobic signals arrive at their target cells, the carrier protein releases them and they readily cross the cell membrane. Therefore, hydrophobic signal molecules do not require transmembrane receptors or second messengers.Instead, hydrophobic signal molecules commonly interact with receptor proteins in the cytosol. Upon binding, cytosolic receptors are transported into the nucleus, where they act as transcription factors to alter expression of one or more genes (see Figure 3.16). Chapter 3: Nonenzymatic Protein Activity110Figure 3.16 Hydrophobic agonists often bind cytosolic receptor proteins.For instance, cortisol (a steroid hormone) binds to a cytosolic receptor and induces transcription of PEPCK, which stimulates glucose production. Consequently, cortisol in this way has an opposite metabolic effect to that of insulin.In general, cytosolic receptors act by altering gene expression, which is a relatively slow process. In contrast, signal cascades often lead to much faster responses by activating or inactivating proteins that are already present in the cell. Note that although hydrophobic signals do not require transmembrane receptors, they can interact with transmembrane proteins and may induce signal cascades.