Biocehm 3.3 Protein Activity PDF
Document Details
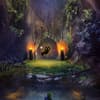
Uploaded by iiScholar
Arizona State University
Tags
Related
Summary
This document discusses ion channels and their roles in biological processes. It details the movement of ions across cell membranes and the factors influencing these processes. The document also includes examples, diagrams and equations.
Full Transcript
ame charge as K+ and a smaller ionic radius, yet Na+ ions cannot pass through a potassium channel pore (see Figure 3.17). This is because potassium channels form specific interactions that result in K+ ion desolvation. In other words, a K+ ion dissociates from the water molecules surrounding it and...
ame charge as K+ and a smaller ionic radius, yet Na+ ions cannot pass through a potassium channel pore (see Figure 3.17). This is because potassium channels form specific interactions that result in K+ ion desolvation. In other words, a K+ ion dissociates from the water molecules surrounding it and instead binds to residues within the pore. In contrast, solvated Na+ ions are too large to enter the pore, while desolvated Na+ ions are too small to be stabilized by the protein residues in the pore. Chapter 3: Nonenzymatic Protein Activity114Figure 3.17 A potassium ion desolvates, moves through an ion channel, and resolvates. Other cations cannot form the specific protein-ligand interactions that stabilize potassium.Solutes can move through channels in either direction. However, if one side of the membrane contains a greater solute concentration than the other, then it is more likely that a solute on the high-concentration side will encounter the pore. Consequently, for uncharged solutes, more solutes move through the pore from high concentration to low concentration than the other way around. Accordingly, the net flow of uncharged solutes through a pore is down the concentration gradient (ie, from high concentration to low concentration). In other words, channels mediate the process of.However, most channels facilitate passage of ions, which are charged particles. Consequently, the exerted by these charges must be considered. For instance, consider a membrane-bound sac (ie, a vesicle) that contains a high concentration of potassium chloride on the inside and a low concentration on the outside. The membrane contains a channel that allows potassium to flow, but not chloride. Under these conditions, potassium will flow from the high concentration inside to the low concentration outside.Initially, both sides of the membrane have a net charge of 0 as each potassium ion cancels each chloride ion. Once potassium begins to flow out, however, positive charges build up on the outer side of the membrane. The positive charges resist additional flow and drive potassium ions back toward the high-concentration inner side of the membrane.Simultaneously, negative charges build up inside the vesicle due to the anions that remain. These negative charges keep potassium ions away from the channel. Eventually, flow occurs at equal rates in both directions and net flow stops, even though the potassium concentrations on each side of the membrane are not equal. Figure 3.18 depicts cation flow and charge buildup across a membrane. Chapter 3: Nonenzymatic Protein Activity115Figure 3.18 As ions flow through a channel, charge separation produces a counteracting force that resists additional flow.Therefore, rather than flowing down their concentration gradient alone, ions flow down their , which involves both concentration and charge. The resulting separation of charge across a cell membrane gives rise to a resting membrane potential (ie, a voltage across the membrane at electrochemical equilibrium). The voltage required to maintain an uneven distribution of a given ion across a membrane (ie, the equilibrium potential) is given by the Nernst equation:𝐸=𝑅𝑇𝑧𝐹ln\[X\]out\[X\]inin which R is the , T is the , z is the charge per ion, and F is Faraday\'s constant (\~96,500 C/mol). \[X\]out is the extracellular ion concentration, and \[X\]in is the intracellular ion concentration. At a physiological temperature of 310 K, the R, T, and F components can be combined, and the ln function can be converted to log10 by dividing by log10(e). This yields𝐸=0.0615 V𝑧×log10\[X\]out\[X\]inConverting this value to millivolts (mV) yields𝐸=61.5 mV𝑧×log10\[X\]out\[X\]inBased on this equation, when the intracellular concentration of a positive ion is greater than the extracellular concentration, the equilibrium potential is negative. This is because cation outflow causes negative charges to build up on the inside until net flow stops (ie, equilibrium is reached). The opposite is true for negative ions: increased intracellular anion concentrations correspond to a positive membrane potential due to anion outflow. Chapter 3: Nonenzymatic Protein Activity116Concept Check 3.10A vesicle contains a sodium concentration of 100 mM in its interior. The vesicle is then immersed in a solution containing 10 mM sodium. If a Na+ ionophore (ie, a molecule that allows sodium to cross the membrane) is added, what membrane potential is required to prevent sodium ions from diffusing out of the vesicle?In general, cells exchange more than one type of ion with their environment through various transmembrane proteins. Therefore, the actual membrane potential is a function of all the different ions contributing to the charge separation, along with the permeability (P) of each. An ion that cannot cross the membrane has a permeability of 0. Because it cannot cross the membrane, it has no ion flow and therefore no direct effect on membrane potential. As channels permit more flow of a certain ion, the permeability for that ion increases.Commonly, membrane potential is modeled on the assumption that sodium (Na+), potassium (K+), and chloride (Cl−) are the primary contributing ions. Therefore, the equation to determine the membrane potential of a typical cell is given as𝐸=61.5 mV×log10𝑃Na+\[Na+\]out+𝑃K+\[K+\]out+𝑃Cl-\[Cl-\]in𝑃Na+\[Na+\]in+𝑃K+\[K+\]in+𝑃Cl-\[Cl-\]outIn this equation, the chloride ion uses the in:out ratio rather than the out:in ratio because chloride is negatively charged. Typically, cells have a negative resting membrane potential, meaning the interior of the cell is more negatively charged (or less positively charged) than the exterior.Concept Check 3.11A certain cell membrane is permeable to both K+ and Cl−, each with permeability constants of 0.2. Initially, the membrane is fully impermeable to Na+. The intracellular concentration of Na+ is less than that of the extracellular concentration. If a stimulus is added that increases the permeability of Na+ to 5.0, what effect will this have on the membrane potential?3.3.02 Types of ChannelsTo establish a resting membrane potential, some ions must be allowed to diffuse into or out of cells while others are restricted. Therefore, ion flow must be carefully controlled. Consequently, many channels are gated, meaning they are closed under resting conditions and do not allow ion flow. Upon receipt of a particular signal, the channel opens through a conformational change, and ions flow until the gate closes again (eg, due to signal removal). Ion channels are categorized by whether they are gated and what type of signal is required to open the gate. Various channel types are shown in Figure 3.19. Chapter 3: Nonenzymatic Protein Activity117Figure 3.19 Various types of ion channels open in response to different stimuli.Ungated Ion ChannelsSome ion channels are ungated. They are always open and their specific ions constantly flow through them. These channels are also known as leak channels.Most cells contain ungated potassium channels in their membranes. Typically, the intracellular concentration of potassium is higher than that of extracellular potassium, and ungated potassium channels allow potassium to flow out of the cell. However, because of the resting membrane potential and efforts made by the cell to actively pump potassium into the cytosol (see Concept 3.3.03), ungated potassium channels usually do not cause potassium to come to equal concentrations on both sides of the membrane.Other ungated channels are commonly found in the membranes of intracellular organelles. These channels allow ions to flow between the cytosol and the lumen of the organelle.Ligand-Gated Ion ChannelsLigand-gated ion channels open in response to a ligand binding to the extracellular portion of the protein. In this way, these channels act as receptor proteins, with the response being the opening of the gate. Prolonged exposure to the ligand can result in desensitization, which causes the gate to close even in the continued presence of ligand. However, channels that have not been desensitized remain open as long as the ligand is bound. Once the ligand dissociates from the channel, the gate closes and flow stops.As an example, muscle cells commonly receive signals from neurons in the form of acetylcholine. When acetylcholine binds to channels on the muscle cell membrane, the channels open and allow sodium ions to flow into the cell, which produces a more positive membrane potential. Because the resting membrane potential is negative, this is called depolarization. A strong enough depolarization can trigger a cascade that results in muscle contraction (see Biology Chapter 17).Voltage-Gated Ion ChannelsVoltage-gated ion channels are sensitive to membrane potential. Each voltage-gated channel has a threshold membrane potential at which it opens. Voltage-gated channels typically open in response to membrane potentials caused by other channels. The opening of a voltage-gated channel may either augment or counteract the effect of other channels on membrane potential. Chapter 3: Nonenzymatic Protein Activity118For example, when acetylcholine causes ligand-gated sodium channels to open in muscle cells, the resulting increase in membrane potential (ie, depolarization) causes voltage-gated sodium channels to open, allowing faster entry of sodium into the cell to enhance the depolarization.Eventually, the voltage-gated sodium channels inactivate and close, and the slower-responding voltage-gated potassium channels open. Potassium flows out of the cell, decreasing the membrane potential to resting level (repolarization) or beyond (hyperpolarization).Mechanically Gated Ion ChannelsMechanically gated ion channels open as a result of physical stimuli such as membrane stretching. These channels are involved in sensations such as touch, in which channels open in response to pressure.Channels for Uncharged ParticlesMost channels facilitate the flow of ions across a membrane, but some channels facilitate the flow of uncharged particles. For example, aquaporins (Figure 3.20) are highly conserved channels that allow the passage of water across a membrane. These channels are critical for osmosis and therefore for regulation of osmotic pressure.Figure 3.20 Aquaporins are membrane channels that allow water molecules to cross the membrane. Chapter 3: Nonenzymatic Protein Activity119Concept Check 3.12Two channels, Channel A and Channel B, are tested to determine how they are gated. Cells that express neither channel, one channel, or both channels in their membranes are exposed to Molecule X, an electrically neutral molecule, and the effect on membrane potential was measured. The results are summarized in the following table.Cell membrane containsMembrane potentialNeither channelDoes not changeChannel A onlyIncreasesChannel B onlyDoes not changeChannels A and BIncreases then decreasesBased on this information, how are Channels A and B most likely gated?3.3.03 Carrier ProteinsLike channel proteins, carrier proteins are transmembrane proteins that help move solutes from one side of the cell membrane to the other. However, when channels are open, they allow continuous solute flow. In contrast, carrier proteins move a discrete number of molecules across the membrane each time they operate. In addition, while channels only move solutes down their electrochemical gradient, carriers may move solutes down or up their gradients (Figure 3.21). Chapter 3: Nonenzymatic Protein Activity120Figure 3.21 Comparison of channel and carrier proteins.The simplest mechanism for transport by a carrier involves a single solute. Upon binding to the solute (either extracellular or intracellular, depending on the system), the carrier undergoes a conformational change that moves the solute into or out of the cell. Once the solute is released into its new location, the carrier can revert to its resting conformation. Transport of one solute in one direction is called uniport.More complicated mechanisms exist for carriers that transport more than one solute per action. A carrier may bind two solutes, both on the same side of the membrane, and move them to the other side of the membrane. This general mechanism, in which both solutes move in the same direction, is called symport. Another mechanism, called antiport, involves transport of one solute into the cell while another is transported out of the cell (Figure 3.22).Figure 3.22 Uniport, antiport, and symport.Carrier proteins may move solutes against their gradient (ie, from low concentration to high concentration) by coupling the movement to a source of energy. This energy coupling often involves hydrolysis of an NTP (eg, ATP, GTP). Carriers that follow this mechanism are classified as translocase enzymes (see Concept 4.2.08), and they facilitate.The is an example of an antiporter that uses primary active transport. This carrier transports three sodium ions out of the cell and two potassium ions into the cell, using ATP hydrolysis to pump both sodium and potassium against their electrochemical gradients.Other carrier proteins gain the energy needed to move a solute against its gradient by moving another solute down its gradient. This mechanism is called. Typically, the solute that moves down its gradient was previously pumped against its gradient by a translocase (ie, through primary active transport). Secondary active transport may occur through symport or antiport.In addition to ions, carrier proteins also transport larger polar molecules across the membrane. GLUT2 and GLUT4, which transport glucose into cells, are carrier proteins. GLUT2 also carries glucose out of liver cells during (see Chapter 11). Other transporters facilitate movement of amino acids, water-soluble vitamins, and other nutrients.