Electron Transport Chain Biochem 12.2 PDF
Document Details
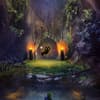
Uploaded by iiScholar
Arizona State University
Tags
Summary
This document provides an overview of the electron transport chain (ETC), a series of oxidation-reduction reactions in the inner mitochondrial membrane. The reactions begin with the oxidation of NADH or FADH2 and transfer electrons through a series of intermediates to molecular oxygen. It also discusses various complexes and shuttles involved in the process.
Full Transcript
# The Electron Transport Chain ## Introduction The electron transport chain (ETC) is a series of oxidation-reduction reactions catalyzed by protein complexes in the inner mitochondrial membrane. These reactions begin with the oxidation of either NADH or FADH2, which were produced by the citric aci...
# The Electron Transport Chain ## Introduction The electron transport chain (ETC) is a series of oxidation-reduction reactions catalyzed by protein complexes in the inner mitochondrial membrane. These reactions begin with the oxidation of either NADH or FADH2, which were produced by the citric acid cycle and other upstream metabolic processes. The electrons from NADH and FADH2 are passed through a series of intermediates, with molecular oxygen (O2) being the final electron acceptor. Oxygen is converted to water by this process. Figure 12.17 provides an overview of the ETC. ## Overview of the Electron Transport Chain Three of the complexes in the ETC use the energy released by the reactions they catalyze to pump protons out of the mitochondrial matrix and into the intermembrane space (ie, the region between the inner and outer mitochondrial membranes). This produces a proton gradient such that the matrix has a lower proton concentration (and therefore a higher pH) than the intermembrane space. This gradient is critical for the production of ATP by ATP synthase, as discussed in Lesson 12.3. This lesson explores the functions of each of the four complexes in the ETC. ## Complex I ### The Complex I Reaction: The first complex of the ETC (Complex I) is named NADH:ubiquinone oxidoreductase or, alternatively, NADH dehydrogenase. As these names suggest, Complex I catalyzes the oxidation of NADH by dehydrogenation (specifically, by removal of a hydride ion). The hydride is transferred to ubiquinone (also called coenzyme Q, UQ, or Q), which becomes ubiquinol, also called UQH2 or QH2. The NADH becomes NAD+, which can then return to the citric acid cycle (or other pathways) to again be reduced to NADH. The general reaction between NADH and ubiquinone is shown in Figure 12.18. ### Complex I & NADH NADH is a relatively hydrophilic molecule. Therefore, this molecule is found in aqueous environments such as the cytosol or, in the context of the ETC, the mitochondrial matrix. Ubiquinone, on the other hand, is highly hydrophobic and is found within the inner mitochondrial membrane. Accordingly, NADH interacts with the portion of Complex I that faces the aqueous mitochondrial matrix. The two electrons are passed to the transmembrane portion of the complex through a series of reactions, where they are finally passed to ubiquinone, as depicted in Figure 12.19. ## The Malate-Aspartate Shuttle Lesson 11.1 explains that glycolysis produces NADH, which must be converted back to NAD+ for glycolysis to continue. Under low oxygen conditions, NAD+ is regenerated by fermentation. In contrast, when oxygen is present, NAD+ may be regenerated by the action of Complex I of the electron transport chain. However, the NAD+ and NADH associated with glycolysis are found in the cytosol of eukaryotes, whereas the NAD+ and NADH associated with the ETC are found in the mitochondrial matrix. The inner mitochondrial membrane is not permeable to either molecule, so NADH generated by glycolysis cannot interact with Complex I directly. To allow regeneration of cytosolic NAD+ without using fermentation, cells may use the malate-aspartate shuttle, shown in a simplified form in Figure 12.20. ## Complex II ### The Complex II Reaction: The citric acid cycle produces two reduced cofactors that enter the electron transport chain: NADH and FADH2. Complex I is the entry point of NADH into the ETC, and Complex II is the entry point for FADH2. As discussed in Lesson 12.1, Complex II is the succinate dehydrogenase enzyme found in the citric acid cycle. When this enzyme oxidizes succinate to fumarate, it reduces FAD to FADH2 in the process. This FAD/FADH2 unit is a prosthetic group of the enzyme. FADH2 passes its electrons through a series of reactions that converts FADH2 back to FAD. As with Complex I, the electrons in Complex II are eventually transferred to ubiquinone (Q) in the inner membrane (see Figure 12.21). ## The Glycerol-3-Phosphate Shuttle The glycerol-3-phosphate shuttle is another shuttle that allows electron transfer from cytosolic NADH to mitochondrial electron acceptors. In this shuttle, the cytosolic isoform of the enzyme glycerol-3-phosphate dehydrogenase removes two electrons from NADH and passes them to dihydroxyacetone phosphate (DHAP), an intermediate of glycolysis. The result is NAD+ and glycerol 3-phosphate. Glycerol 3-phosphate then transfers two electrons to an FAD prosthetic group within a different isozyme of glycerol-3-phosphate dehydrogenase, this one a transmembrane enzyme of the inner mitochondrial membrane. This process yields FADH2 and restores DHAP. The resulting FADH2 then passes the electrons to ubiquinone. The overall effect of this process is synthesis of ubiquinol without pumping any protons from the mitochondrial matrix to the intermembrane space. Therefore, although this shuttle does not use Complex II directly, it produces the same result. Figure 12.22 shows this shuttle. ## Complex III ### The Complex III Reaction: Complexes I and II produce ubiquinol (the reduced form of ubiquinone) in their reactions. Other enzymes in other pathways also produce ubiquinol in the inner mitochondrial membrane (eg, the glycerol-3-phosphate shuttle). Once produced, ubiquinol can interact with Complex III of the ETC, also known as ubiquinone:cytochrome c oxidoreductase. This complex facilitates transfer of electrons from ubiquinol to a protein called cytochrome c (abbreviated as cyt c), which is a soluble protein often found associated with the outer leaflet of the inner membrane (ie, cyt c is in the intermembrane space). Figure 12.23 depicts the overall reaction carried out by Complex III. ## The Q Cycle The Q cycle starts with two coenzyme Q molecules: one in its reduced form (ubiquinol, QH2), carrying the high-energy electrons, and the other in its oxidized form (ubiquinone, Q). As shown in Step 1 of Figure 12.24, ubiquinol (QH2) passes one electron to cyt c and another electron to ubiquinone (Q). The original QH2 molecule has therefore become fully oxidized to Q and leaves the cycle. Meanwhile, the original Q molecule becomes a partially reduced form called semiquinone, denoted • Q-. The single dot indicates that semiquinone is a radical, meaning it has an unpaired electron. In Step 2 of Figure 12.24, another QH2 molecule then enters the cycle and passes one electron to a cyt c molecule and the other electron to the • Q-. The original QH2 from this step becomes Q, and • Q- becomes fully reduced to QH2. The net result from the two steps of this cycle is that one QH2 transfers its electrons to two oxidized cyt c molecules, yielding one Q and two reduced cyt c molecules, as described by the following summed equations: ## Oxidative Stress Oxygen gas must cross into the hydrophobic portion of the inner mitochondrial membrane before it can interact with Complex IV (see Concept 12.2.04). The various forms of coenzyme Q, including semiquinone, are found within this membrane. The semiquinone produced in Complex III is highly reactive and may react with oxygen to form reactive oxygen species (ROS) such as superoxide (•O2-). Superoxide can lead to the condition of oxidative stress, in which components of a cell that should not be oxidized become oxidized. The resulting damage to various components of the cell can lead to apoptosis (ie, controlled cell death, see Figure 12.25). In this process, the cyt c molecules that are normally associated with the inner mitochondrial membrane are released into the cytosol, where they activate proteases such as caspase. These proteases then degrade specific proteins, and the cell dies. ## Complex IV ### The Complex IV Reaction: The reduced cyt c produced by Complex III migrates to Complex IV, also known as cytochrome c oxidase. Oxygen gas (O2) binds tightly to a heme group within Complex IV, and four cyt Cred molecules successively pass their electrons to the bound oxygen through a series of intermediates. Each oxygen atom also reacts with two protons, and the result is two water molecules per molecular oxygen (O2) reduced, as shown in Figure 12.26. Note that the four cyt Cred shown in this figure must have come from two molecules of either NADH or FADH2. ## Electron Transport Chain and the Citric Acid Cycle Because oxygen is the final electron acceptor in the chain, the ETC cannot function in the absence of oxygen. In anaerobic conditions (ie, no oxygen or low oxygen), reduced cyt c cannot lose an electron and therefore remains in reduced form. This results in a buildup of cyt Cred and depletion of cyt Cox by Complex III. When cyt cox is depleted, ubiquinol is unable to donate its electrons and remains in reduced form. Therefore, ubiquinol builds up and ubiquinone is depleted. This in turn results in a buildup of NADH and FADH2, which inhibits the citric acid cycle as no NAD+ or FAD is available to receive electrons. For this reason, both the ETC and the citric acid cycle function only under aerobic conditions (see Figure 12.27), and fermentation is used under anaerobic conditions. ## Concept Check 12.4 If the citric acid cycle is inhibited, what effect will this have on the ratio of ubiquinone to ubiquinol in the inner mitochondrial membrane? ## Net Proton Yield of the ETC For each oxygen molecule that is reduced to water, Complex IV pumps four protons into the intermembrane space. Note, however, that full reduction of O2 requires four electrons, whereas each NADH or FADH2 molecule contributes only two electrons. Therefore, two NADH molecules, two FADH2 molecules, or one NADH molecule and one FADH2 molecule are required to fully reduce a single O2 molecule. In other words, Complex IV pumps two protons for every NADH or FADH2 molecule that enters the ETC. Every NADH molecule that enters the ETC causes four protons to be pumped into the intermembrane space by Complex I, another four protons by Complex III, and another two protons by Complex IV, for a total of 10 protons per NADH. FADH2 also results in four protons pumped by Complex III and two protons pumped by Complex IV, for a total of six protons per FADH2. The number of protons pumped per reduced cofactor is shown in Figure 12.28.