Lecture 10: Electron Transport, Oxidative Phosphorylation, and Oxygen Metabolism PDF
Document Details
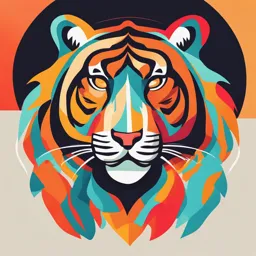
Uploaded by ProfoundBrown
Tags
Summary
This document is a lecture on electron transport, oxidative phosphorylation, and oxygen metabolism in biochemistry. It provides detailed information about the mitochondrial processes essential to cellular respiration. The document covers major aspects including glycolysis, the citric acid cycle, and the respiratory chain.
Full Transcript
1 Lecture 10 Electron Transport, Oxidative Phosphorylation, and Oxygen Metabolism The Mitochondrion: Scene of the Action 2 The average adult human synthesizes ATP at a rate of nearly 1021 molecules per second, equivalent to producing his or her own weight in...
1 Lecture 10 Electron Transport, Oxidative Phosphorylation, and Oxygen Metabolism The Mitochondrion: Scene of the Action 2 The average adult human synthesizes ATP at a rate of nearly 1021 molecules per second, equivalent to producing his or her own weight in ATP every day. Glycolysis and the citric acid cycle by themselves generate relatively little ATP directly. However, under aerobic conditions, six dehydrogenation steps: o One in glycolysis o One in pyruvate dehydrogenase o Four in the citric acid cycle Collectively reduce 10 moles of NAD+ to NADH and 2 moles of FAD to FADH2 per mole of glucose. Reoxidation of these reduced electron carriers in the process termed cellular respiration generates most of the energy needed for ATP synthesis. Oxidation of 1 mole of NADH by the respiratory chain provides sufficient energy for synthesis of ~2.5 moles of ATP from ADP. The Mitochondrion: Scene of the Action 3 In eukaryotic cells NADH and FADH2 are reoxidized by electron transport proteins bound to the inner mitochondrial membrane. A series of linked oxidation and reduction reactions occurs, with electrons being passed along a series of electron carriers—the electron transport chain. The final step is reduction of O2 to water. The overall electron transport sequence is quite exergonic. One pair of reducing equivalents, generated from 1 mole of NADH, suffices to drive the synthesis of between 2 and 3 moles of ATP from ADP and by a process termed oxidative phosphorylation. The Mitochondrion: Scene of the Action 4 Localization of respiratory processes in the mitochondrion: a) A mitochondrion from a pancreatic cell. b) Overview of oxidative phosphorylation: Reduced electron carriers, produced by cytosolic dehydrogenases and mitochondrial oxidative pathways, become reoxidized by enzyme complexes bound in the inner membrane. These complexes actively pump protons outward, creating an energy gradient whose discharge through complex V drives ATP synthesis. The Mitochondrion: Scene of the Action 5 Electron Transport 6 Embedded within the inner membrane are the protein carriers that constitute the respiratory chain. Electron Transport 7 Embedded within the inner membrane are the protein carriers that constitute the respiratory chain. Complex I and complex II receive electrons from the oxidation of NADH and succinate, respectively, and pass them along to a lipid-soluble electron carrier, coenzyme Q, which moves freely in the membrane. Complex III catalyzes the transfer of electrons from the reduced form of coenzyme Q to cytochrome c, a protein electron carrier that is also mobile within the intermembrane space. Complex IV catalyzes the oxidation of cytochrome c, reducing O2 to water. The energy released by these exergonic reactions creates a proton gradient across the inner membrane, with protons being pumped from the matrix into the intermembrane space. Protons then re-enter the matrix through a specific channel in complex V. The energy released by this exergonic process drives the endergonic synthesis of ATP from ADP and inorganic phosphate. Electron Transport 8 Respiratory electron carriers in the mitochondrion: The sequence of electron carriers that oxidize succinate and NAD-linked substrates in the inner membrane. The respiratory chain catalyzes the transport of electrons from low-potential carriers to high-potential carriers. Electron Transport 9 Electron Carriers in the Respiratory Chain: Flavoproteins o Contain tightly bound FMN or FAD as redox cofactors. o Each flavoprotein provides a different microenvironment for the isoalloxazine ring, conferring a unique standard reduction potential on the flavin. o Flavin nucleotides can act as transformers between two-electron and one-electron processes due to their ability to exist as stable one- electron reduced semiquinone intermediates. Iron–sulfur proteins o Iron–sulfur clusters consist of nonheme iron complexed to sulfur in four known ways. Coenzyme Q Cytochromes Electron Transport 10 Structures of iron–sulfur clusters: The top panel illustrates the covalent attachment of a pair of clusters in a subunit of complex I from Thermus thermophilus 11 Electron Transport The cytochrome Q carrier was found to be a benzoquinone linked to a number of isoprene units, usually 10 in mammalian cells and 6 in bacteria. Because the substance is ubiquitous in living cells, one group of researchers named it ubiquinone, while another called it coenzyme Q, or Q. Electron Transport 12 The hemes found in cytochromes: a)The covalent bond formed between heme and the protein component in cytochromes c and c1. The vinyl groups on heme are linked to the thiol groups of two cysteine residues (red). b) Heme A, the form found in cytochromes a and a3. Note the modified side chains—a formyl group (red) and an isoprenoid side chain (blue). b) Four-helix bundle structure of cytochrome b562 from E. coli Electron Transport 13 Standard reduction potentials of the major respiratory electron carriers: Three reactions in the respiratory chain have DGo’ values greater than -30.5 kJ/mol, the DGo’ for ATP hydrolysis: FMN à Q; cyt b à cyt c1; and cyt a à O2. Electron Transport 14 Sites of action of some respiratory inhibitors and artificial electron acceptors: This schematic of the respiratory chain from NADH to O2 shows the sites of action of some useful inhibitors (red) and some artificial electron acceptors (blue). Each acceptor is positioned according to its value (in parentheses), identifying the most likely site at which an acceptor will withdraw electrons from the respiratory chain when added to mitochondria. 15 Electron Transport The order of action of electron carriers in the respiratory chain was explained by: o difference spectrophotometry o analysis of respiratory inhibitors o properties of membrane complexes. Electron Transport 16 Multiprotein complexes in the mitochondrial respiratory assembly: The subscripts for the b cytochromes denote their spectral maxima. The two b hemes in complex III, identified as cyt b562 and cyt b566, are bound to the same polypeptide chain. The yellow arrows denote the energy released by the actions of complexes I, III, and IV used to drive the synthesis of ATP by complex V (ATP synthase). Electron Transport 17 The overall reaction catalyzed by the NADH dehydrogenase complex is shown below. Because the electrons are subsequently used to reduce coenzyme Q, a more descriptive name for this complex is NADH–coenzyme Q reductase. Electron transport through complex I is coupled to the pumping of protons from the matrix to the intermembrane space. 18 Electron Transport Structure and function of complex I (NADH–coenzyme Q reductase): Model of complex I from the yeast Yarrowia lipolytica X-ray structure of the entire complex I from the archaea Thermus thermophilus. Proposed model of proton translocation by complex I. NADH binds near FMN. Proposed model for coupling of electron transport to proton pumping by complex I. Electron Transport 19 Structure of complex II (succinate dehydrogenase): The enzyme is composed of two hydrophilic subunits extending into the matrix, the FAD-binding subunit (blue) and the iron–sulfur subunit (yellow), and two transmembrane subunits (pink and gold). The path of electron transport from FAD through the three iron–sulfur clusters to coenzyme Q (UQ) is shown on the right. Succinate binds near FAD in the blue subunit. Electron Transport 20 Coenzyme Q collects electrons from multiple flavoproteins. This lipid-soluble mobile redox cofactor serves as the electron acceptor for at least four mitochondrial flavoprotein dehydrogenases. Electrons from NADH and succinate are delivered via complex I and complex Il (succinate dehydrogenase), respectively. Electron-transferring flavoprotein (ETF): ubiquinone oxidoreductase catalyzes the transfer of electrons from reduced ETF to Q. These electrons originate in the acyl- CoA dehydrogenase step of fatty acid beta oxidation. Glycerol-3-phosphate dehydrogenase, located on the intermembrane face of the inner membrane, delivers electrons from glycerol-3-phosphate to coenzyme Q. Electrons from reduced QH2 eventually pass to complex Ill of the respiratory chain. Electron Transport 21 Structure of Complex III (coenzyme Q:cytochrome c oxidoreductase): a)X-ray structure of the dimeric complex a)Cartoon of the complex III dimer illustrating the arrangement of the subunits and redox carriers. The approximate location of the complex in the inner membrane is indicated. 22 Electron Transport Structure of cytochrome c oxidase (complex IV): Four electrons are donated, one at a time, by reduced cyt c, to the CuA center, through heme a, and on to the catalytic site (binuclear a3–CuB site) where one molecule of O2 is reduced, yielding two molecules of H2O. Protons are pumped from the matrix side to the intermembrane space (IMS) side of the membrane. 23 Electron Transport Model of proposed supercomplex of complexes I, III, and IV: Oxidative Phosphorylation 24 Experimental identification of “coupling sites.” Electron transport is restricted to particular parts of the chain by use of selected electron donors, electron acceptors, and respiratory inhibitors, as indicated. For each segment of the chain that is thus isolated, P/O ratios are determined, allowing identification of coupling sites. Oxidative Phosphorylation 25 Chemiosmotic coupling of electron transport and ATP synthesis: Protons are pumped by complexes I, III, and IV as electrons flow through the complexes, generating an electrochemical gradient across the membrane (protonmotive force, pmf). Proton re-entry to the matrix, through the F0 channel of ATP synthase (complex V), provides the energy to drive ATP synthesis. Chemiosmotic coupling refers to the use of a transmembrane proton gradient to drive endergonic processes like ATP synthesis. Oxidative Phosphorylation 26 Uncouplers Act by Dissipating the Proton Gradient The lipophilic weak acids 2,4-dinitrophenol (DNP) and trifluorocarbonylcyanide phenylhydrazone (FCCP) are called uncoupling agents, or uncouplers. Uncoupling agents, when added to mitochondria, permit electron transport along the respiratory chain to O2 to occur in the absence of ATP synthesis. They uncouple the process of electron transport from the process of ATP synthesis. Oxidative Phosphorylation 27 Structure of the F0F1 complex: The F0F1 complex, also called ATP synthase or complex V, contains an F1 knob projecting into the mitochondrial matrix and connected by a central stalk to the F0 base. The globular F1 knob contains three ab dimers, arranged about the central stalk, which is made up of g, d and e subunits. The F0 base is composed of 10–12 c subunits (the c-ring) and one subunit a. The peripheral stalk (subunits b, d, F6, and OSCP) is attached to the F0 base via subunit a and at least four other minor membrane-embedded subunits that are not shown. The central stalk and the c-ring compose the “rotor” of ATP synthase. The remainder of the subunits make up the “stator,” a structure that prevents the rotation of the three dimers of F1. Oxidative Phosphorylation 28 Structure of the mitochondrial ATP synthase complex: Oxidative Phosphorylation 29 Binding change model for ATP synthase: The nucleotide binding site (catalytic site) of the three dimers exists in three different conformations, termed loose (L), tight (T), and open (O). The subunit rotates counterclockwise, driven by the passage of protons through channels in F0, while the dimer assemblies are held stationary by a stator. Step 1 represents 120° rotation of the subunit, leading to a conformational change in all three dimers, such that the T site changes to an O site, leading to ATP release, and an O site changes to an L site, binding ADP and Pi. The third site changes from a L conformation, with loosely bound ADP and Pi, to a T conformation, where the substrates are tightly bound, leading to ATP formation in step 2. Oxidative Phosphorylation 30 The experimental system that permits observation of rotation in the F1 component of F1F0 ATP synthase: The cloned gene encoding the F1b subunit was modified by adding a sequence that encodes an oligohistidine sequence that binds to a nickel-coated bead (Ni-NTA coated bead) in the conformation shown. Thus, the complex was immobilized on the bead and attached to a glass coverslip. Streptavidin is a protein used to couple fluorescent- tagged actin to the subunit. Oxidative Phosphorylation 31 The experimental system that permits observation of rotation in the F1 component of F1F0 ATP synthase: Fluorescence microscopic examination showed that, following addition of ATP and its subsequent hydrolysis by the catalytic subunits, the actin molecule was rotating, which proved that the subunit itself was rotating. The time interval between images is 133 ms. Oxidative Phosphorylation 32 Proton-driven rotation of the c-ring of the component of ATP synthase: This model is based on the X-ray structure of the E. coli ATP synthase. Oxidative Phosphorylation 33 Experimental demonstration of respiratory control: Oxygen uptake is monitored in carefully prepared coupled mitochondria. The addition of an exogenous oxidizable substrate (glutamate) stimulates respiration only slightly, unless ADP + Pi is added as well. Both ADP additions represent limiting amounts; the second addition is twice the amount of the first, to show that the magnitude of oxygen uptake is stoichiometric. The slow oxygen uptake at the beginning results from endogenous substrates in the mitochondria. ADP stimulates respiration only until all of the ADP + Pi has been converted to ATP. Oxygen uptake is recorded in moles O, because one pair of electrons reduces one atom of O, not one molecule of O2. Oxidative Phosphorylation 34 In most aerobic cells the level of ATP exceeds that of ADP by 4- to 10-fold. It is convenient to think of respiratory control as a dependence of respiration on ADP as a substrate for phosphorylation. If the energy demands on a cell cause ATP to be consumed at high rates, the resultant accumulation of ADP will stimulate respiration, with concomitant activation of ATP resynthesis. Conversely, in a relaxed and well-nourished cell, ATP accumulates at the expense of ADP, and the depletion of ADP limits the rate of both electron transport and its own phosphorylation to ATP. Thus, the energy-generating capacity of the cell is closely attuned to its energy demands. Oxidative Phosphorylation 35 Oligomycins created by Streptomyces - poisonous to other organisms.They have use as antibiotics. Oligomycin A inhibits ATP synthase by blocking its proton channel (F0 subunit) Effects of an inhibitor and an uncoupler on oxygen uptake and ATP synthesis. DNP uncouples respiration from phosphorylation so that O2 uptake is stimulated even in the presence of oligomycin, but ATP synthesis remains blocked. Oxidative Phosphorylation 36 Reversibility of F1F0 ATP synthase: a)In normally respiring mitochondria, the pmf is high and ATP synthase operates in the direction of ATP synthesis. The ATP is exchanged for cytoplasmic ADP via the adenine nucleotide translocase (ant). a)During hypoxia, the pmf falls, and the cell relies mainly on homolactic (or alcoholic) fermentation for ATP production. This ATP enters the matrix in exchange for ADP. F1F0 ATP synthase operates as a proton-translocating ATPase, pumping protons out of the matrix, temporarily sustaining the pmf to support other processes, such as metabolite transport. 37 Mitochondrial Transport Systems Major inner membrane transport systems for respiratory substrates and products: The ADP/ATP carrier and the phosphate translocase move substrates for oxidative phosphorylation (ADP and Pi) into the mitochondrion and the product (ATP) out. Other transport systems move substrates and products for citric acid cycle oxidation into or out of the matrix, as dictated by the metabolic needs of the cell. Mitochondrial Transport Systems 38 Electrons are transported into mitochondria by metabolic shuttles. Reducing equivalents are shuttled from cytosol into mitochondria. a)The dihydroxyacetone phosphate/glycerol-3-phosphate shuttle. a)The malate/aspartate shuttle. 39 Energy Yields from Oxidative Metabolism The complete oxidation of 1 mole of glucose generates about 30–32 moles of ATP synthesized from ADP. Oxygen as a Substrate for 40 Other Metabolic Reactions The term oxidase is applied to enzymes that catalyze the oxidation of a substrate without incorporation of oxygen atom into the product. A two-electron oxidation is usually involved, so the oxygen is converted to H2O2. Most oxidases utilize either a metal or a flavin coenzyme. D-Amino acid oxidases, for example, use FAD as a cofactor. Oxygen as a Substrate for 41 Other Metabolic Reactions Oxygenases are enzymes that incorporate oxygen atoms from into the oxidized products; there are two classes—monooxygenases and dioxygenases. Dioxygenases, which incorporate both atoms of into one substrate, are of limited distribution. An example is tryptophan 2,3-dioxygenase, which contains a heme cofactor and catalyzes the first reaction in tryptophan catabolism: Oxygen as a Substrate for 42 Other Metabolic Reactions Far more widely distributed are monooxygenases, which incorporate one atom from into a product and reduce the other atom to water. A monooxygenase has one substrate that accepts oxygen and another that furnishes the two H atoms that reduce the other oxygen to water. Because two substrates are oxidized, enzymes of this class are also called mixed-function oxidases. The general reaction catalyzed by monooxygenases is as follows: Because the substrate AH usually becomes hydroxylated by this class of enzymes, the term hydroxylase is also used. An example of this type of reaction is the hydroxylation of steroids. Here the reductive cofactor, NADPH, appears as BH2. Oxygen as a Substrate for 43 Other Metabolic Reactions Cytochrome P450: a)Active site of human cytochrome CYP2A6 in complex with the anticoagulant coumarin. a)The flavoprotein enzyme P450 oxidoreductase (POR) delivers electrons one at a time from NADPH to cytochrome P450. Copyright © 2013 Pearson Canada Inc. 15 - 43 Oxygen as a Substrate for 44 Other Metabolic Reactions Cytochromes P450 are involved in hydroxylating a large variety of compounds. These reactions include the hydroxylations in steroid hormone biosynthesis and the synthesis of hydroxylated fatty acids and fatty acid epoxides. In addition, cytochromes P450 act upon thousands of xenobiotics. Hydroxylation of foreign substances usually increases their solubility and is an important step in their detoxification, or metabolism and excretion. However, some of these reactions result in activation of potentially carcinogenic substances to more reactive species, as shown for aflatoxin B1 which is converted to more reactive species either by hydroxylation or epoxidation. Reactive Oxygen Species, 45 Antioxidant Defenses, and Human Disease Reactive oxygen species (ROS): The generation and interconversion of the most common reactive oxygen species are shown: o Superoxide o Hydroxyl radical o Nitric oxide o Peroxynitrite o Oxidized coenzyme Q Reactive Oxygen Species, 46 Antioxidant Defenses, and Human Disease Hydroxyl radical is the most active mutagen derived from ionizing radiation. Hydroxyl radical is also produced from in the Fenton reaction: Reactive Oxygen Species, 47 Antioxidant Defenses, and Human Disease Superoxide per se is relatively nontoxic. Because it contains an unpaired electron, it is a free radical, and it combines readily with another free radical, nitric oxide, a biological signaling agent that is produced in many animal tissues. The product is peroxynitrite also considered a reactive oxygen species. Peroxynitrite causes lipid peroxidation and also causes nitration of tyrosyl hydroxyl groups in proteins, a reaction particularly damaging to membrane proteins. Reactive Oxygen Species, 48 Antioxidant Defenses, and Human Disease Hydroxyl radical also damages nucleic acids, both by causing polynucleotide strand breakage (double-stranded DNA breaks are lethal) and by changing the structure of DNA bases. About 20 different base changes, or DNA lesions, are known to result from reactions of hydroxyl radical with DNA. Some lesions are mutagenic because the altered base created, such as 8-oxoguanine, forms non-Watson–Crick base pairs during DNA replication. Other lesions, such as thymine glycol, are potentially lethal because, unless the lesion is repaired their occurrence in DNA blocks replication past that site. Reactive Oxygen Species, 49 Antioxidant Defenses, and Human Disease Uncontrolled overproduction of reactive oxygen species has the potential to inflict considerable damage on the tissues in which they are produced, a situation called oxidative stress. A series of elaborate mechanisms has evolved to minimize its harmful consequences. The nonenzymatic protection is afforded by antioxidant compounds: o Glutathione o Vitamins C and E o Uric acid These compounds can scavenge ROS before they can cause damage, or they can prevent oxidative damage from spreading. Reactive Oxygen Species, 50 Antioxidant Defenses, and Human Disease Among enzymatic mechanisms the first line of defense is superoxide dismutase (SOD), a family of metalloenzymes that catalyze a dismutation (a reaction in which two identical substrate molecules have different fates). Here, one molecule of superoxide is oxidized and one is reduced. Hydrogen peroxide is metabolized either by peroxiredoxins, a ubiquitous family of thiol proteins, catalase, another widely distributed enzyme, or by a more limited family of peroxidases. Reactive Oxygen Species, 51 Antioxidant Defenses, and Human Disease Catalase is a heme protein with an extremely high turnover rate (> 40,000 molecules per second). It catalyzes the following reaction: 2 H2O2 à 2 H2O + O2 Peroxidases, which are widely distributed in plants, reduce H2O2 to water at the expense of oxidation of an organic substrate. An example of a peroxidase is found in erythrocytes, which are especially sensitive to peroxide accumulation. Within erythrocytes is glutathione peroxidase, an enzyme that reduces H2O2 to water, along with the oxidation of glutathione. 2 GSH + H2O2 à GSSG + 2 H2O 52