Chapter 19 Oxidative Phosphorylation PDF
Document Details
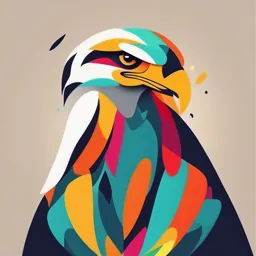
Uploaded by TrustworthyAgate6970
2021
David L. Nelson • Michael M. Cox
Tags
Summary
This document is a chapter on oxidative phosphorylation, part of a biochemistry textbook. It covers principles, the chemiosmotic theory, and questions related to the mitochondrial respiratory chain. It also includes diagrams and descriptions of the components of the electron transport chain.
Full Transcript
19 Oxidative Phosphorylation © 2021 Macmillan Learning Principle 1 (1 of 5) Mitochondria play a central role in eukaryotic aerobic metabolism. ATP production is not the only important mitochondrial function. Mitochondria play host to the citric acid cycle, the fatty acid β-...
19 Oxidative Phosphorylation © 2021 Macmillan Learning Principle 1 (1 of 5) Mitochondria play a central role in eukaryotic aerobic metabolism. ATP production is not the only important mitochondrial function. Mitochondria play host to the citric acid cycle, the fatty acid β-oxidation pathway, and the pathways of amino acid oxidation. The mitochondria also act in thermogenesis, steroid synthesis, and apoptosis (programmed cell death). The discovery of these diverse and important roles of mitochondria has stimulated much current research on the biochemistry of this organelle. Principle 2 (1 of 4) Mitochondria trace their evolutionary origin to bacteria. Over 1.45 billion years ago, an endosymbiotic relationship arose between bacteria and a primitive eukaryote or eukaryotic progenitor. Mitochondria are ubiquitous in modern eukaryotes, and their bacterial origin is evident in almost every aspect of their structure and function. Principle 3 (1 of 6) Electrons flow from electron donors (oxidizable substrates) through a chain of membrane-bound carriers to a final electron acceptor with a large reduction potential. The final acceptor is molecular oxygen, O2. The appearance of oxygen in the atmosphere some 2.3 billion years ago, and its harnessing in living systems via the evolution of oxidative phosphorylation, made more complex life forms possible. Principle 4 (1 of 5) The free energy made available by “downhill” (exergonic) electron flow is coupled to the “uphill” transport of protons across a proton-impermeable membrane. The free energy of fuel oxidation is thus conserved as a transmembrane electrochemical potential. Principle 5 (1 of 7) The transmembrane flow of protons back down their electrochemical gradient through specific protein channels provides the free energy for synthesis of ATP. This process is catalyzed by a membrane protein complex (ATP synthase) that couples proton flow to phosphorylation of ADP. The Chemiosmotic Theory chemiosmotic theory = transmembrane differences in proton concentration are the reservoir for the energy extracted from biological oxidation reactions 19.1 The Mitochondrial Respiratory Chain Mitochondria Have Two Membranes outer membrane is readily permeable to small molecules and ions – transport occurs through porins inner membrane is impermeable to most small molecules and ions – transport requires specific transporters Principle 1 (2 of 5) Mitochondria play a central role in eukaryotic aerobic metabolism. ATP production is not the only important mitochondrial function. Mitochondria play host to the citric acid cycle, the fatty acid β-oxidation pathway, and the pathways of amino acid oxidation. The mitochondria also act in thermogenesis, steroid synthesis, and apoptosis (programmed cell death). The discovery of these diverse and important roles of mitochondria has stimulated much current research on the biochemistry of this organelle. The Mitochondrial Matrix the mitochondrial matrix contains: – the pyruvate dehydrogenase complex – enzymes of the citric acid cycle – enzymes of the fatty acid β-oxidation pathway – enzymes of the pathways of amino acid oxidation the inner mitochondrial membrane segregates the intermediates and enzymes of cytosolic and matrix metabolic pathways Clicker Question 1 Which of these dehydrogenase enzymes is NOT found in the mitochondrial matrix? A. malate dehydrogenase B. glutamate dehydrogenase C. acyl-CoA dehydrogenase D. lactate dehydrogenase Clicker Question 1, Response Which of these dehydrogenase enzymes is NOT found in the mitochondrial matrix? D. lactate dehydrogenase The mitochondrial matrix contains enzymes of the citric acid cycle (malate dehydrogenase), the β-oxidation pathway (acyl-CoA dehydrogenase), and amino acid oxidation (glutamate dehydrogenase). The enzymes of glycolysis and fermentation (lactate dehydrogenase) are located in the cytosol. Mitochondrial Cristae cristae = convolutions in the inner membrane of the mitochondrion – mitochondria of cells with high metabolic activity have more cristae Principle 2 (2 of 4) Mitochondria trace their evolutionary origin to bacteria. Over 1.45 billion years ago, an endosymbiotic relationship arose between bacteria and a primitive eukaryote or eukaryotic progenitor. Mitochondria are ubiquitous in modern eukaryotes, and their bacterial origin is evident in almost every aspect of their structure and function. The Effect of Stress on Mitochondria during cell growth and division, mitochondria divide by fission stressful conditions can trigger: – mitochondrial fission – mitophagy = the breakdown of mitochondria and recycling of the amino acids, nucleotides, and lipids as stress is relieved, small mitochondria fuse to form long, thin, tubular organelles Electrons Are Funneled to Universal Electron Acceptors respiratory chain = series of electron carriers dehydrogenases collect electrons from catabolic pathways and funnel them into universal electron acceptors: – nicotinamide nucleotides (NAD+ or NADP+) – flavin nucleotides (FMN or FAD) Nicotinamide Nucleotide- Linked Dehydrogenases nicotinamide nucleotide-linked dehydrogenases = catalyze reversible reactions of the general types: reduced substrate + NAD+ ⇄ oxidized substrate + NADH + H+ reduced substrate + NADP+ ⇄ oxidized substrate + NADPH + H+ two hydrogen atoms are removed from the substrates: – one is transferred as a hydride ion (:H-) to NAD(P)+ – one is released as H+ in the medium Important Reactions Catalyzed by NAD(P)+-Linked Dehydrogenases Clicker Question 2 Which enzyme would NOT be expected to contribute electron carriers to oxidative phosphorylation? A. alcohol dehydrogenase B. malate dehydrogenase C. succinate dehydrogenase D. glucose 6-phosphate dehydrogenase E. glyceraldehyde 3-phosphate dehydrogenase Clicker Question 2, Response Which enzyme would NOT be expected to contribute electron carriers to oxidative phosphorylation? D. glucose 6-phosphate dehydrogenase Glucose 6-phosphate dehydrogenase produces NADPH, which does not contribute electrons to the respiratory chain. Principle 3 (2 of 6) Electrons flow from electron donors (oxidizable substrates) through a chain of membrane-bound carriers to a final electron acceptor with a large reduction potential. The final acceptor is molecular oxygen, O2. The appearance of oxygen in the atmosphere some 2.3 billion years ago, and its harnessing in living systems via the evolution of oxidative phosphorylation, made more complex life forms possible. Flavoproteins flavoproteins = contain a very tightly, sometimes covalently, bound flavin nucleotide (FMN or FAD) the oxidized flavin nucleotide can accept either: – one electron (yielding the semiquinone form) or – two electrons (yielding FADH2 or FMNH2) electron transfer occurs because the flavoprotein has a higher E° than the compound oxidized – E′° of a flavin nucleotide depends on the protein with which it is associated Electrons Pass through a Series of Membrane-Bound Carriers three types of electron transfers occur in oxidative phosphorylation: – direct transfer of electrons – transfer as a hydrogen atom (H+ + e−) – transfer as a hydride ion (:H−) reducing equivalent = a single electron equivalent transferred in an oxidation-reduction reaction Clicker Question 3 Which ion, atom, or molecule constitutes one reducing equivalent? A. proton (H+) B. hydrogen atom (H+ + e−) C. hydride ion (:H−) D. NADH Clicker Question 3, Response Which ion, atom, or molecule constitutes one reducing equivalent? B. hydrogen atom (H+ + e−) The term reducing equivalent is used to designate a single electron equivalent transferred in an oxidation-reduction reaction. A proton cannot act as a reducing equivalent because it has no electrons. A hydrogen atom acts as one reducing equivalent. A hydride ion or NADH molecule acts as two reducing equivalents. Principle 3 (3 of 6) Electrons flow from electron donors (oxidizable substrates) through a chain of membrane-bound carriers to a final electron acceptor with a large reduction potential. The final acceptor is molecular oxygen, O2. The appearance of oxygen in the atmosphere some 2.3 billion years ago, and its harnessing in living systems via the evolution of oxidative phosphorylation, made more complex life forms possible. Electron-Carrying Molecules in the Respiratory Chain five types of electron-carrying molecules: – NAD – flavoproteins – ubiquinone (coenzyme Q or Q) – cytochromes – iron-sulfur proteins Ubiquinone ubiquinone (coenzyme Q or Q) = a lipid-soluble benzoquinone with a long isoprenoid side chain – can accept one or two electrons – freely diffusible within the inner mitochondrial membrane – plays a central role in coupling electron flow to proton movement Cytochromes cytochromes = proteins with characteristic strong absorption of visible light due to their iron-containing heme prosthetic groups – one-electron carriers – 3 classes in mitochondria: a, b, and c hemes of a and b are not covalently bound to associated proteins c is covalently attached through Cys residues Prosthetic Groups of Cytochromes Clicker Question 4 Which statement is false about the cytochrome electron carriers? A. Cytochromes a, b, and c are distinguished by differences in their light-absorption spectra. B. Soluble cytochrome c associates with the outer surface of the inner membrane through electrostatic interactions. C. The heme of cytochrome c is tightly, but not covalently, bound to its associated protein. D. Cytochromes a and b are integral proteins of the inner mitochondrial membrane. Clicker Question 4, Response Which statement is false about the cytochrome electron carriers? C. The heme of cytochrome c is tightly, but not covalently, bound to its associated protein. The hemes of a and b cytochromes are tightly, but not covalently, bound to their associated proteins; the hemes of c-type cytochromes are covalently attached through Cys residues. Iron-Sulfur Proteins iron-sulfur proteins = proteins that contain iron in association with inorganic sulfur atoms and/or with the sulfur atoms of Cys residues in the protein – participate in one-electron transfers Rieske iron-sulfur proteins = proteins in which one Fe atom is coordinated to two His residues Clicker Question 5 Which compound is NOT an electron carrier involved in the respiratory chain? A. NADH B. iron-sulfur proteins C. cytochromes D. coenzyme A Clicker Question 5, Response Which compound is NOT an electron carrier involved in the respiratory chain? D. coenzyme A Coenzyme A serves multiple metabolic functions in both anabolic and catabolic pathways; however, it does not act as an electron carrier in the respiratory chain. Methods for Determining The Sequence of Electron Carriers determine the E′° of the individual electron carriers reduce the entire chain of carriers by omitting O2 and then measure the oxidation rate of each electron carrier when O2 is reintroduced measure the effects of inhibitors of electron transfer on the oxidation state of each carrier Standard Reduction Potentials of Individual Electron Carriers electrons tend to flow spontaneously from carriers of lower E′° to carriers of higher E′° Table 19-2 Standard Reduction Potentials of Respiratory Chain and Related Electron Carriers Redox reaction (half-reaction) E′° (V) 2H+ + 2e−→H2 −0.414 NAD+ + H+ + 2e−→NADH −0.320 NADP+ + H+ + 2e−→NADPH −0.324 NADH dehydrogenase (FMN) + 2H+ + 2e−→NADH dehydrogenase (FMNH2) −0.30 Ubiquinone + 2H+ + 2e−→ubiquinol 0.045 Cytochrome b (Fe3+) + e−→cytochrome b (Fe2+) 0.077 Cytochrome c1 (Fe3+) + e−→cytochrome c1 (Fe2+) 0.22 Cytochrome c (Fe3+) + e−→cytochrome c (Fe2+) 0.254 Cytochrome a (Fe3+) + e−→cytochrome a (Fe2+) 0.29 Cytochrome a3 (Fe3+) + e−→cytochrome a3 (Fe2+) 0.35 ½ O2 + 2H+ + 2e−→H2O 0.817 The Sequence of Electron Carriers the order of carriers is: NADH → Q → cytochrome b → cytochrome c1 → cytochrome c → cytochrome a → cytochrome a3 → O2 order has been confirmed by all three approaches Principle 3 (4 of 6) Electrons flow from electron donors (oxidizable substrates) through a chain of membrane-bound carriers to a final electron acceptor with a large reduction potential. The final acceptor is molecular oxygen, O2. The appearance of oxygen in the atmosphere some 2.3 billion years ago, and its harnessing in living systems via the evolution of oxidative phosphorylation, made more complex life forms possible. Electron Carriers Function in Multienzyme Complexes four unique electron-carrier complexes catalyze electron transfer through a portion of the chain: – Complex I (from NADH to ubiquinone) – Complex II (from succinate to ubiquinone) – Complex III (from ubiquinone to cytochrome c) – Complex IV (from cytochrome c to O2) Clicker Question 6 Which electron-carrier complex in the respiratory chain oxidizes ubiquinone? A. Complex I B. Complex II C. Complex III D. Complex IV Clicker Question 6, Response Which electron-carrier complex in the respiratory chain oxidizes ubiquinone? C. Complex III Complex III carries electrons from reduced ubiquinone to cytochrome c. Protein Components of the Mitochondrial Respiratory Chain Table 19-3 The Protein Components of the Mitochondrial Respiratory Chain Enzyme complex/protein Mass (kDa) Number of subunits Prosthetic group(s) I NADH dehydrogenase 850 45 (14) FMN, Fe-S II Succinate dehydrogenase 140 4 FAD, Fe-S III Ubiquinone: cytochrome c oxidoreductase 250 11 Hemes, Fe-S Cytochrome c 13 1 Heme IV Cytochrome oxidase 204 13 (3–4) Hemes; CuA, CuB Separation of Functional Complexes of the Respiratory Chain Complex I: NADH to Ubiquinone Complex I (NADH:ubiquinone oxidoreductase, NADH dehydrogenase) = large L-shaped enzyme with >40 polypeptide chains – FMN-containing flavoprotein accepts 2 electrons from NADH – 8+ Fe-S centers pass elections to ubiquinone Clicker Question 7 How many electrons would enter Complex I from complete oxidation of myristic acid, 14:0? A. 18 B. 40 C. 42 D. 52 E. 54 Clicker Question 7, Response How many electrons would enter Complex I from complete oxidation of myristic acid, 14:0? E. 54 Myristic acid undergoes 6 rounds of β oxidation, producing 7 acetyl-CoA and 6 NADH (1 NADH per round). Each acetyl-CoA yields 3 NADH in the citric acid cycle. 6 NADH + (7 acetyl-CoA×3 NADH/acetyl-CoA) = 27 NADH Each NADH molecule donates 2 electrons to Complex I. 27 NADH×2e−/NADH = 54e− Complex I Catalyzes Two Simultaneous and Obligately Coupled Processes Complex I catalyzes: – the exergonic transfer of a hydride ion from NADH and a proton from the matrix to ubiquinone NADH + H+ + Q → NAD+ + QH2 (19-1) – the endergonic transfer of 4 protons from the matrix to the intermembrane space Principle 4 (2 of 5) The free energy made available by “downhill” (exergonic) electron flow is coupled to the “uphill” transport of protons across a proton-impermeable membrane. The free energy of fuel oxidation is thus conserved as a transmembrane electrochemical potential. Complex I Is a Proton Pump Complex I is a proton pump driven by the energy of electron transfer Complex I catalyzes a vectorial reaction (moves protons in a specific direction from one location to another) The Overall Reaction of Complex I the overall reaction is: NADH + 5H+N + Q → NAD+ + QH2 + 4H+P (19-2) where the subscript letters indicate the location of the protons: P indicates the positive side (the intermembrane space) and N indicates the negative side (the matrix) Agents that Inhibit Oxidative Phosphorylation inhibitors of Complex I: – amytal (a barbiturate drug) – rotenone (an insecticide) – piericidin A (an antibiotic) Clicker Question 8 Which statement is false about Complex I? A. It consists of more than 40 different polypeptide chains. B. It has an FMN-containing flavoprotein. C. It catalyzes the transfer of a hydride from NADH and a proton from the matrix to ubiquinone (Q). D. Its activity makes the matrix more positively charged. Clicker Question 8, Response Which statement is false about Complex I? D. Its activity makes the matrix more positively charged. Complex I transfers four protons from the matrix to the intermembrane space. The loss of positively charged protons makes the matrix more negative. Complex II: Succinate to Ubiquinone Complex II (succinate dehydrogenase) = couples the oxidation of succinate with the reduction of ubiquinone – electrons pass from FAD to Fe-S centers to Q – no proton pumping – also functions to convert succinate to fumarate in the citric acid cycle Heme b of Complex II heme b reduces the frequency with which electrons “leak” out of the system, moving from succinate to molecular oxygen to produce the reactive oxygen species (ROS) H2O2 and the superoxide radical Clicker Question 9 Which electron carrier or prosthetic group would NOT function after site-directed mutagenesis substituted Pro for Cys in succinate dehydrogenase? A. cytochrome c B. iron-sulfur center C. flavin adenine dinucleotide D. ubiquinone E. FMN Clicker Question 9, Response Which electron carrier or prosthetic group would NOT function after site-directed mutagenesis substituted Pro for Cys in succinate dehydrogenase? B. iron-sulfur center Subunits A and B contain three iron-sulfur (2Fe-2S) centers, in which the iron is present in association with the sulfur atoms of Cys residues in the protein. Principle 3 (5 of 6) Electrons flow from electron donors (oxidizable substrates) through a chain of membrane-bound carriers to a final electron acceptor with a large reduction potential. The final acceptor is molecular oxygen, O2. The appearance of oxygen in the atmosphere some 2.3 billion years ago, and its harnessing in living systems via the evolution of oxidative phosphorylation, made more complex life forms possible. Principle 4 (3 of 5) The free energy made available by “downhill” (exergonic) electron flow is coupled to the “uphill” transport of protons across a proton-impermeable membrane. The free energy of fuel oxidation is thus conserved as a transmembrane electrochemical potential. Complex III: Ubiquinone to Cytochrome c Complex III (cytochrome bc1 complex, ubiquinone: cytochrome c oxidoreductase) = couples the transfer of 2 electrons from ubiquinol to cytochrome c with the vectorial transport of four protons to the intermembrane space – contains cytochrome b, cytochrome c1, and the Rieske iron-sulfur protein The Path of Electrons through Complex III ubiquinone diffuses through the inner mitochondrial membrane and transfers the electrons to cytochrome b electrons pass to an Fe-S center which passes electrons, one at a time, through cytochrome c The Q Cycle The Net Equation for the Redox Reactions of the Q Cycle the net equation is: QH2 + 2 cyt c (oxidized) + 2H+N → (19-3) Q + 2 cyt c (reduced) + 4H+P Clicker Question 10 Which statement is false about Complex III? A. It holds ubiquinone on the matrix side of the inner mitochondrial membrane throughout the redox process. B. Its functional unit consists of two dimers made up of cytochrome b, cytochrome c1, and the Rieske iron-sulfur protein. C. It couples the oxidation of two molecules of reduced ubiquinone (QH2) with the reduction of two molecules of cytochrome c. D. It catalyzes the net movement of two protons from the N side to the P side of the inner mitochondrial membrane. Clicker Question 10, Response Which statement is false about Complex III? A. It holds ubiquinone on the matrix side of the inner mitochondrial membrane throughout the redox process. The two cytochrome b monomers surround a cavern in the middle of the membrane in which ubiquinone is free to move from the matrix side of the membrane (site QN on one monomer) to the intermembrane space (site QP on the other monomer) as it shuttles electrons and protons across the inner mitochondrial membrane. Principle 3 (6 of 6) Electrons flow from electron donors (oxidizable substrates) through a chain of membrane-bound carriers to a final electron acceptor with a large reduction potential. The final acceptor is molecular oxygen, O2. The appearance of oxygen in the atmosphere some 2.3 billion years ago, and its harnessing in living systems via the evolution of oxidative phosphorylation, made more complex life forms possible. Principle 4 (4 of 5) The free energy made available by “downhill” (exergonic) electron flow is coupled to the “uphill” transport of protons across a proton-impermeable membrane. The free energy of fuel oxidation is thus conserved as a transmembrane electrochemical potential. Complex IV: Cytochrome c to O2 Complex IV (cytochrome oxidase) = carries electrons from cytochrome c to molecular oxygen, reducing it to H2O – large dimeric enzyme – 3 subunits have been conserved through evolution – contains cytochromes a and a3 – contains 2 Cu ions Cytochrome c cytochrome c: – moves through the intermembrane space – carries a single electron from the cytochrome bc1 complex to Complex IV – absorbs visible light Path of Electrons Through Complex IV The Overall Reaction of Complex IV the net equation is: 4 cyt c (reduced) + 8H+N + O2 → (19-4) 4 cyt c (oxidized) + 4H+P + 2H2O Clicker Question 11 Which complex does NOT transport H+ from the mitochondrial matrix to the intermembrane space? A. Complex I B. Complex II C. Complex III D. Complex IV Clicker Question 11, Response Which complex does NOT transport H+ from the mitochondrial matrix to the intermembrane space? B. Complex II Electron transfer through Complex II is not accompanied by proton pumping across the inner membrane, although the QH2 produced by succinate oxidation will be used by Complex III to drive proton transfer. Mitochondrial Complexes Associate in Respirasomes respirasome = supercomplex containing Complexes I, III, and IV – may facilitate electron transfers or limit ROS production cytochrome c and ubiquinone readily diffuse between supercomplexes Other Pathways Donate Electrons to the Respiratory Chain via Ubiquinone Electrons Derived from the Oxidation of Fatty Acids Pass to Ubiquinone acyl-CoA dehydrogenase = flavoprotein that catalyzes the first step of the β oxidation of fatty acyl–CoA – electrons pass from the substrate to the FAD of the dehydrogenase to electron-transferring flavoprotein (ETF) ETF = passes its electrons to ETF:ubiquinone oxidoreductase – reduces Q in the inner mitochondrial membrane to QH2 The Oxidation of Glycerol Phosphate and Dihydroorotate glycerol 3-phosphate dehydrogenase = flavoprotein located on the outer face of the inner mitochondrial membrane – Q accepts electrons, and the QH2 produced enters the pool of QH2 in the membrane dihydroorotate dehydrogenase = located on the outside of the inner mitochondrial membrane – donates electrons to Q in the respiratory chain Clicker Question 12 Which compound is NOT a direct source of electrons for the respiratory chain? A. cytosolic NADH B. dihydroorotate dehydrogenase C. glycerol 3-phosphate dehydrogenase D. FADH2 Clicker Question 12, Response Which compound is NOT a direct source of electrons for the respiratory chain? A. cytosolic NADH The inner mitochondrial membrane is not permeable to NADH. Thus special shuttle systems carry reducing equivalents from cytosolic NADH into mitochondria by an indirect route. The Energy of Electron Transfer Is Efficiently Conserved in a Proton Gradient the net equation for the transfer of two electrons from NADH through the respiratory chain to molecular oxygen is: 2 NADH + 2H+ + O2 → 2 NAD+ + 2H2O (19-5) The Change in Standard Reduction Potential the standard reduction potential change is: E′° for NAD+/NADH is −0.320 V ∆E′° = E′° (electron acceptor) − E′° (electron donor) E′° for O2/H2O is = 0.816 V − (−0.320 V) 0.816 V = 1.14 V The Net Reaction is Highly Exergonic the standard free-energy change is: ∆G′° = −nF ∆E′° (19-6) = −2(96.5 kJ/V mol)(1.14 V) = −220 kJ/mol (of NADH) Clicker Question 13 Calculate the standard free-energy change for the transfer of two electrons from FADH2 through the respiratory chain to molecular oxygen (E′° for FAD/FADH2 is 0.050 V in succinate dehydrogenase and E′° for O2/H2O is 0.816 V). A. −220 kJ/mol B. −150 kJ/mol C. 150 kJ/mol D. 220 kJ/mol Clicker Question 13, Response Calculate the standard free-energy change for the transfer of two electrons from FADH2 through the respiratory chain to molecular oxygen (E′° for FAD/FADH2 is 0.050 V in succinate dehydrogenase and E′° for O2/H2O is 0.816 V). B. −150 kJ/mol ∆E′° = E′° (electron acceptor) − E′° (electron donor) = 0.816 V − 0.050 V = 0.766 V ∆G′° = −nF ∆E′° = −2(96.5 kJ/V mol)(0.766 V) = −150 kJ/mol Summary of the Flow of Electrons and Protons through the Respiratory Chain much of the free energy generated is recovered and stored in the form of an electrochemical proton gradient across the mitochondrial inner membrane The Net Vectorial Equation the net vectorial equation is: 2 NADH + 22H+N + O2 → (19-7) 2 NAD+ + 20H+P + 2H2O Principle 4 (5 of 5) The free energy made available by “downhill” (exergonic) electron flow is coupled to the “uphill” transport of protons across a proton-impermeable membrane. The free energy of fuel oxidation is thus conserved as a transmembrane electrochemical potential. Proton-Motive Force proton-motive force = the energy stored in an electrochemical proton gradient across the mitochondrial inner membrane – composed of chemical and electrical potential energy Clicker Question 14 The electron-transfer chain generates ATP by: A. creating a proton-motive force. B. substrate-level phosphorylation. C. activating uncoupling protein 1 (UCP1). D. pumping phosphate ions into the intermembrane space. E. adding electrons to ADP to form ATP. Clicker Question 14, Response The electron-transfer chain generates ATP by: A. creating a proton-motive force. The flow of electrons through Complexes I, III, and IV results in the pumping of protons across the inner mitochondrial membrane, making the matrix alkaline relative to the intermembrane space. This proton gradient provides the energy, in the form of the proton-motive force, for ATP synthesis from ADP and Pi. Clicker Question 15 Which statement regarding the proton-motive force is false? A. It is a result of electron flow through the respiratory chain. B. It is used by ATP synthase to synthesize ATP. C. It results from an [H+] gradient across the outer mitochondrial membrane. D. It is both chemical and electrical potential energy. Clicker Question 15, Response Which statement regarding the proton-motive force is false? C. It results from an [H+] gradient across the outer mitochondrial membrane. The proton-motive force results from an [H+] gradient across the inner, not the outer, mitochondrial membrane. The Free-Energy Change for the Creation of an Electrochemical Gradient by an Ion Pump the free-energy change is: ∆G = RT ln(C2/C1) + ZF ∆ψ (19-8) where C2 and C1 are the concentrations of an ion in two regions, Z is the absolute value of its electrical charge, and ∆ψ is the transmembrane difference in electrical potential (in volts) Calculating the Proton-Motive Force the free-energy change is: ∆G = RT ln(C2/C1) + ZF ∆ψ = RT (2.3(log[H+]P − log[H+]N) + ZF ∆ψ = RT (2.3(pHN − pHp) + ZF ∆ψ = RT (2.3 ∆pH) + ZF ∆ψ = 2.3RT ∆pH + ZF ∆ψ (19-9) Clicker Question 16 Calculate the free-energy change for the creation of an electrochemical gradient by a proton pump if the pH of the matrix is 0.75 units more alkaline than that of the intermembrane space, the transmembrane difference in electrical potential is 0.20 V, and the temperature is 37 °C. A. −24 kJ/mol B. −15 kJ/mol C. 15 kJ/mol D. 24 kJ/mol Clicker Question 16, Response Calculate the free-energy change for the creation of an electrochemical gradient by a proton pump if the pH of the matrix is 0.75 units more alkaline than that of the intermembrane space, the transmembrane difference in electrical potential is 0.20 V, and the temperature is 37 °C. D. 24 kJ/mol ∆G = 2.3RT ∆pH + ZF ∆ψ = 2.3(0.008315 kJ/mol K)(310 K)(0.75) + (+1)(96.5 kJ/V mol)(0.20 V) = 19.3 kJ/mol + 4.45 kJ/mol = 24 kJ/mol Reactive Oxygen Species Are Generated during Oxidative Phosphorylation superoxide dismutase = catalyzes the conversion of superoxide and two protons to H2O2 and O2 glutathione peroxidase = converts H2O2 to H2O low ROS levels serve as signals reflecting insufficient O2 Clicker Question 17 Why are reactive oxygen species (ROS) generated? A. Molecular oxygen recombines with hydrogen to produce dihydrogen oxide. B. Stray electrons bind to oxygen, creating a free radical oxygen species. C. There are high ADP levels. D. There is a high NAD+/NADH ratio. E. Transfer of a H+ across the inner mitochondrial membrane is uncoupled from ATP synthase. Clicker Question 17, Response Why are reactive oxygen species (ROS) generated? B. Stray electrons bind to oxygen, creating a free radical oxygen species. Some intermediates in the electron-transfer system, such as the partially reduced ubisemiquinone ( QH), can react directly with oxygen to form the superoxide radical ( O2−) as an intermediate.The superoxide radical forms when a single electron is passed to O2 in the reaction: O 2 + e− → O 2 − Clicker Question 18 How is production of reactive oxygen species (ROS) controlled? A. through increased ADP content in the matrix B. through an increase in the NAD+/NADH ratio C. with expression of superoxide dismutase D. with expression of glutathione peroxidase E. All of the answers are correct. Clicker Question 18, Response How is production of reactive oxygen species (ROS) controlled? E. All of the answers are correct. The formation of ROS is favored when mitochondria are not making ATP (for lack of ADP or O2) and when there is a high NADH/NAD+ ratio in the matrix. ROS produced in mitochondria are inactivated by a set of protective enzymes, including superoxide dismutase and glutathione peroxidase. 19.2 ATP Synthesis In the Chemiosmotic Model, Oxidation and Phosphorylation Are Obligately Coupled chemiosmotic model = describes the coupling of ATP synthesis to an electrochemical proton gradient (the proton- motive force) ATP Synthesis ATP synthase = drives the synthesis of ATP as protons flow passively back into the matrix through its proton pore to emphasize the role of the proton-motive force, the equation for ATP synthesis is: ADP + Pi + nH+P → ATP + H2O + nH+N (19-10) Clicker Question 19 The chemiosmotic model: A. is supported by the observation that, when isolated mitochondria have an artificial electrochemical gradient (higher pH in the matrix) imposed on them, electrons spontaneously flow through the respiratory chain. B. requires an intact outer mitochondrial membrane. C. requires that mitochondrial ATP synthesis and electron flow through the respiratory chain be obligately coupled. D. is supported by the observation that isolated mitochondria that are actively respiring cause the pH of the solution they are in to increase. Clicker Question 19, Response The chemiosmotic model: C. requires that mitochondrial ATP synthesis and electron flow through the respiratory chain be obligately coupled. The chemiosmotic model, proposed by Peter Mitchell, is the paradigm for energy coupling. Here, “coupling” refers to the obligate connection between mitochondrial ATP synthesis and electron flow through the respiratory chain. Principle 5 (2 of 7) The transmembrane flow of protons back down their electrochemical gradient through specific protein channels provides the free energy for synthesis of ATP. This process is catalyzed by a membrane protein complex (ATP synthase) that couples proton flow to phosphorylation of ADP. Coupling of Electron Transfer and ATP Synthesis in Mitochondria inhibitors of electron transfer block ATP synthesis inhibitors of ATP synthesis block electron transfer (unless oxidation and phosphorylation are uncoupled) Clicker Question 20 The addition of oligomycin, an inhibitor of ATP synthase, to mitochondria suspended in a buffered medium blocks both ATP synthesis and respiration. What would happen if 2,4-dinitrophenol (DNP) were also added to this suspension? A. Respiration and ATP synthesis would both resume. B. Respiration would resume without ATP synthesis. C. ATP synthesis would resume without respiration. D. Neither respiration nor ATP synthesis would resume. Clicker Question 20, Response The addition of oligomycin, an inhibitor of ATP synthase, to mitochondria suspended in a buffered medium blocks both ATP synthesis and respiration. What would happen if 2,4-dinitrophenol (DNP) were also added to this suspension B. Respiration would resume without ATP synthesis. Dinitrophenol (DNP) uncouples respiration from ATP synthesis by entering the matrix in the protonated form and releasing a proton, thus dissipating the proton gradient. This allows respiration to continue without ATP synthesis. Chemical Uncouplers of Oxidative Phosphorylation physical shear or detergent uncouples electron transfer from ATP synthesis DNP and FCCP = weak acids that carry protons across the inner mitochondrial membrane – dissipates the proton gradient Proton-Motive Force Alone Drives ATP Synthesis in the absence of an oxidizable substrate, the proton-motive force alone drives ATP synthesis Principle 2 (3 of 4) Mitochondria trace their evolutionary origin to bacteria. Over 1.45 billion years ago, an endosymbiotic relationship arose between bacteria and a primitive eukaryote or eukaryotic progenitor. Mitochondria are ubiquitous in modern eukaryotes, and their bacterial origin is evident in almost every aspect of their structure and function. ATP Synthase Has Two Functional Domains, Fo and F1 mitochondrial ATP synthase is an F-type ATPase – similar in structure and mechanism to the ATP synthases of bacteria and chloroplasts Principle 5 (3 of 7) The transmembrane flow of protons back down their electrochemical gradient through specific protein channels provides the free energy for synthesis of ATP. This process is catalyzed by a membrane protein complex (ATP synthase) that couples proton flow to phosphorylation of ADP. Mitochondrial ATP Synthase contains two distinct components: – Fo = an integral membrane protein with a proton pore – F1 (originally F1 ATPase) = a peripheral membrane protein catalyzes hydrolysis of ATP when isolated ATP Is Stabilized Relative to ADP on the Surface of F1 on the surface of F1, the reaction ADP + Pi ⇌ ATP + H2O is readily reversible ATP synthase stabilizes ATP relative to ADP + Pi by binding ATP more tightly, releasing enough energy to counterbalance the cost of making ATP The Proton Gradient Drives the Release of ATP from the Enzyme Surface the free energy required for the release of ATP is provided by the proton-motive force Clicker Question 21 What is the major energy barrier for ATP synthase? A. binding of ADP to the enzyme B. binding of Pi to the enzyme C. formation of ATP D. release of ATP from the enzyme Clicker Question 21, Response What is the major energy barrier for ATP synthase? D. release of ATP from the enzyme In a typical enzyme-catalyzed reaction, reaching the transition state between substrate and product is the major energy barrier to overcome. In the reaction catalyzed by ATP synthase, release of ATP from the enzyme, not formation of ATP, is the major energy barrier. Each β Subunit of ATP Synthase Can Assume Three Different Conformations mitochondrial F1 has the composition α3β3γδε – each of the three β subunits has one catalytic site for ATP synthesis – each β subunit has a different conformation: β-ATP β-ADP β-empty The Structure of the Fo Complex mitochondrial Fo has the composition ab2cn – n ranges from 8 to 17 depending on the species c ring = arrangement of c subunits into two concentric circles – the c subunits rotate together as a unit The Structure of the FoF1 Complex Principle 5 (4 of 7) The transmembrane flow of protons back down their electrochemical gradient through specific protein channels provides the free energy for synthesis of ATP. This process is catalyzed by a membrane protein complex (ATP synthase) that couples proton flow to phosphorylation of ADP. Clicker Question 22 The β subunits of ATP synthase: A. have three distinct isozymes. B. are each associated with a δ subunit. C. have three distinct conformations. D. will act as an ATPase if protons flow through the Fo domain into the mitochondrion. Clicker Question 22, Response The β subunits of ATP synthase: C. have three distinct conformations. The F1 complex has three nonequivalent adenine nucleotide–binding sites, one for each pair of α and β subunits. At any given moment, one of these sites is in the β-ATP conformation (which binds ATP tightly), a second is in the β-ADP (loose-binding) conformation, and a third is in the β-empty (very loose-binding) conformation. Rotational Catalysis Is Key to the Binding- Change Mechanism for ATP Synthesis rotational catalysis = mechanism by which the flow of protons through Fo causes the c ring to rotate and, in turn, trigger the subunit conformational changes in F1 The Binding-Change Model binding-change model = the active site of each β subunit cycles through the three conformations: – β-ATP – tight binding – β-ADP – loose binding – β-empty – very loose binding Binding-Change Model for ATP Synthase the three active sites of F1 take turns catalyzing ATP synthesis translocation of three protons fuels synthesis of one ATP each complete rotation synthesizes three ATP Clicker Question 23 With each rotation of 120°, the γ subunit of FoF1 comes into contact with a different β subunit, forcing that β subunit: A. into the β-empty conformation. B. into the β-ADP conformation. C. into the β-ATP conformation. D. to catalyze the synthesis of ATP. Clicker Question 23, Response With each rotation of 120°, the γ subunit of FoF1 comes into contact with a different β subunit, forcing that β subunit: A. into the β-empty conformation. The β subunit changes to the β-empty conformation when it comes into contact with the γ subunit. Because this conformation has very low affinity for ATP, the newly synthesized ATP leaves the enzyme surface. Coupling Proton Translocation to ATP Synthesis proton translocation causes a rotation of F0 and γ – causes a conformational change within all the three αβ pairs, one of which promotes condensation of ADP and Pi into ATP. Experimental Demonstration of Rotation of Fo and γ the γ subunit rotates in one direction when FoF1 is synthesizing ATP and in the opposite direction when hydrolyzing ATP Clicker Question 24 Which statement regarding ATP synthase is false? A. The γ subunit is stationary as the αβ dimers rotate around it. B. Protons flow through the a and c subunits. C. When the F1 domain is isolated, it functions as an ATPase. D. It is considered to have an Fo domain and an F1 domain. Clicker Question 24, Response Which statement regarding ATP synthase is false? A. The γ subunit is stationary as the αβ dimers rotate around it. The streaming of protons through the Fo pore causes the c ring and the attached γ subunit to rotate about the long axis of γ, which is perpendicular to the plane of the membrane. The γ subunit passes through the center of the α3β3 spheroid, which is held stationary relative to the membrane surface. Principle 5 (5 of 7) The transmembrane flow of protons back down their electrochemical gradient through specific protein channels provides the free energy for synthesis of ATP. This process is catalyzed by a membrane protein complex (ATP synthase) that couples proton flow to phosphorylation of ADP. A Model for Proton-Driven Rotation of the c Ring the a subunit is stationary and the c ring rotates transient protonation of a key Glu residue in each c subunit elicits conformation changes that drive rotation and transmit protons Species Differences in Number of c Subunits in the c Ring of the Fo Complex Chemiosmotic Coupling Allows Nonintegral Stoichiometries of O2 Consumption and ATP Synthesis the overall reaction equation for ATP synthesis is: x ADP + xPi + ½ O2 + H+ + NADH → (19-11) x ATP + H2O + NAD+ P/O ratio (P/2e− ratio) = the value of x – there is no theoretical requirement for P/O to be integral The P/O Ratio the P/O ratio is: – ~2.5 when electrons enter the respiratory chain at Complex I – ~1.5 when electrons enter at ubiquinone the ratio varies among species, depending on the number of c subunits in the Fo complex Clicker Question 25 Succinate dehydrogenase is dysfunctional in a species of garden slug. While its metabolism is compromised on a number of levels, it can still undergo oxidative phosphorylation. What is the maximal P/O ratio for these organisms if NADH is used as an electron source? A. 1 B. 1.5 C. 2 D. 2.5 E. 4 Clicker Question 25, Response Succinate dehydrogenase is dysfunctional in a species of garden slug. While its metabolism is compromised on a number of levels, it can still undergo oxidative phosphorylation. What is the maximal P/O ratio for these organisms if NADH is used as an electron source? D. 2.5 If 10 protons are pumped out per NADH and 4 must flow in to produce 1 ATP, the proton-based P/O ratio is 2.5 for NADH as the electron donor. The Proton-Motive Force Energizes Active Transport adenine nucleotide translocase = antiporter that moves ADP into the matrix and ADP out phosphate translocase = promotes symport of one H2PO4- and one H+ into the matrix ATP synthasome = a translocation of a fourth complex of ATP synthase proton per ATP is required and both translocases to facilitate cotransport Shuttle Systems Indirectly Convey Cytosolic NADH into Mitochondria for Oxidation the inner mitochondrial membrane is impermeable to NADH and NAD NADH equivalents are moved from the cytosol to the matrix by: – the malate-asparate shuttle – the glycerol 3-phosphate shuttle The Malate-Aspartate Shuttle NADH equivalents moved in by the malate- aspartate shuttle enter the respiratory chain at Complex I and yield a P/O ratio of 2.5 The Glycerol 3-Phosphate Shuttle NADH equivalents moved in by the glycerol 3- phosphate shuttle enter the respiratory chain at Complex III and yield a P/O ratio of 1.5 Clicker Question 26 Why is the malate-aspartate shuttle necessary for oxidative phosphorylation in the liver? A. to bring malate into the mitochondrial matrix B. to bring aspartate out of the mitochondrial matrix C. to bring the reducing equivalents of NADH into the mitochondrial matrix D. to convert oxaloacetate into malate E. to convert glutamate into α-ketoglutarate Clicker Question 26, Response Why is the malate-aspartate shuttle necessary for oxidative phosphorylation in the liver? C. to bring the reducing equivalents of NADH into the mitochondrial matrix NADH dehydrogenase (Complex I) of the inner mitochondrial membrane can accept electrons only from NADH in the matrix; however, the inner membrane is not permeable to NADH. Thus special shuttle systems carry reducing equivalents from cytosolic NADH into mitochondria by an indirect route. Clicker Question 27 Why is the glycerol 3-phosphate shuttle necessary for oxidative phosphorylation in the brain? A. to bring malate into the mitochondrial matrix B. to bring aspartate out of the mitochondrial matrix C. to bring the reducing equivalents of NADH into the mitochondrial matrix D. to convert oxaloacetate into malate E. to convert glutamate into α-ketoglutarate Clicker Question 27, Response Why is the glycerol 3-phosphate shuttle necessary for oxidative phosphorylation in the brain? C. to bring the reducing equivalents of NADH into the mitochondrial matrix NADH dehydrogenase (Complex I) of the inner mitochondrial membrane can accept electrons only from NADH in the matrix; however, the inner membrane is not permeable to NADH. Thus special shuttle systems carry reducing equivalents from cytosolic NADH into mitochondria by an indirect route. Clicker Question 28 Shuttles are used to transport reducing equivalents and molecules, which themselves have no specific transporter, through the inner mitochondrial membrane. Which molecule CANNOT move through the membrane directly? A. pyruvate B. malate C. glutamate D. α-ketoglutarate E. oxaloacetate Clicker Question 28, Response Shuttles are used to transport reducing equivalents and molecules, which themselves have no specific transporter, through the inner mitochondrial membrane. Which molecule CANNOT move through the membrane directly? E. oxaloacetate In the matrix, malate passes two reducing equivalents to NAD+, and the resulting NADH is oxidized by the respiratory chain. The oxaloacetate formed from malate cannot pass directly into the cytosol and must first be transaminated to aspartate, which can leave via the glutamate-aspartate shuttle. 19.3 Regulation of Oxidative Phosphorylation ATP Yield from Complete Oxidation of Glucose Table 19-5 ATP Yield from Complete Oxidation of Glucose Process Direct product Final ATP Glycolysis 2 NADH (cytosolic) 3 or 5 2 ATP 2 Pyruvate oxidation (two per glucose) 2 NADH (mitochondrial matrix) 5 Acetyl-CoA oxidation in citric acid cycle (two per glucose) 6 NADH (mitochondrial matrix) 15 2 FADH2 3 2 ATP or 2 GTP 2 Total yield per glucose 30 or 32 glycolysis under anaerobic conditions (lactate fermentation) yields only 2 ATP per glucose Oxidative Phosphorylation Is Regulated by Cellular Energy Needs the acceptor control of respiration = ADP – the rate of O2 consumption depends on the availability of ADP, the Pi acceptor the acceptor control ratio = the ratio of the maximal rate of ADP-induced O2 consumption to the basal rate in the absence of ADP – at least 10 in some animal tissues The Mass-Action Ratio is a Measure of a Cell’s Energy Status mass-action ratio = [ATP]/([ADP][Pi]) – usually this ratio is very high – when lowered (and more ADP is available), the rate of respiration increases Clicker Question 29 Which molecule is part of an important mass-action ratio in most cells and is a modulator of the three major ATP-producing pathways? A. ADP B. NAD+ C. AMP D. acetate E. NADH Clicker Question 29, Response Which molecule is part of an important mass-action ratio in most cells and is a modulator of the three major ATP-producing pathways? A. ADP The mass-action ratio of the ATP-ADP system is [ATP]/([ADP][Pi ]). With more ADP available for oxidative phosphorylation, the rate of respiration increases, causing regeneration of ATP. This continues until the mass-action ratio returns to its normal high level, at which point respiration slows again. Principle 5 (6 of 7) The transmembrane flow of protons back down their electrochemical gradient through specific protein channels provides the free energy for synthesis of ATP. This process is catalyzed by a membrane protein complex (ATP synthase) that couples proton flow to phosphorylation of ADP. An Inhibitory Protein Prevents ATP Hydrolysis IF1 = protein inhibitor that simultaneously binds 2 ATP molecules to inhibit enzyme activity in both directions – active in its dimeric form, which occurs when pH < 6.5 in hypoxic (oxygen-deprived) cells, IF1 binds and prevents a drastic drop in [ATP]. Hypoxia Leads to ROS Production and Several Adaptive Responses defenses against ROS: – glutathione peroxidase system – regulation of pyruvate dehydrogenase phosphorylated (inactive) under hypoxic conditions – modification of a subunit of Complex IV (COX4-1 to COX4-2) acts more efficiently under hypoxic conditions Regulation of Gene Expression by HIF-1 HIF-1 = the hypoxia- inducible factor – accumulates in hypoxic cells – acts as a transcription factor – master regulator of O2 homeostasis – mediates changes in glucose transport and glycolytic enzymes that produce the Warburg effect Clicker Question 30 In hypoxic cells: A. a subunit of Complex IV is replaced with another subunit optimized for activity under hypoxic conditions. B. PDH activity increases. C. flux through glycolysis decreases. D. levels of reactive oxygen species decline. Clicker Question 30, Response In hypoxic cells: A. a subunit of Complex IV is replaced with another subunit optimized for activity under hypoxic conditions. One means of preventing reactive oxygen species formation is the replacement of one subunit of Complex IV, known as COX4-1, with another subunit, COX4-2, that is better suited to hypoxic conditions. ATP-Producing Pathways Are Coordinately Regulated ATP and ADP relative concentrations control the rates of: – electron transfer – oxidative phosphorylation – the citric acid cycle – pyruvate oxidation – glycolysis Regulation of ATP-Producing Pathways Clicker Question 31 How is oxidative phosphorylation inhibited during anaerobic conditions? A. A lack of terminal electron acceptor blocks the electron- transfer chain. B. ADP levels decrease. C. A buildup of glycolytic products (pyruvic acid, lactic acid) lowers pH. D. Inhibitory protein IF1 becomes functionally dimeric under acidic conditions and binds the ATP synthase inhibiting it. E. All of the answers are correct. Clicker Question 31, Response How is oxidative phosphorylation inhibited during anaerobic conditions? E. All of the answers are correct. Under anaerobic conditions, the main source of ATP becomes glycolysis, and the pyruvic or lactic acid thus formed lowers the pH in the cytosol and the mitochondrial matrix. This favors IF1 dimerization, leading to inhibition of ATP synthase. The rate of oxidative phosphorylation also decreases when less ADP (the substrate) and O2 (the terminal electron acceptor) are available. 19.4 Mitochondria in Thermogenesis, Steroid Synthesis, and Apoptosis Principle 1 (3 of 5) Mitochondria play a central role in eukaryotic aerobic metabolism. ATP production is not the only important mitochondrial function. Mitochondria play host to the citric acid cycle, the fatty acid β-oxidation pathway, and the pathways of amino acid oxidation. The mitochondria also act in thermogenesis, steroid synthesis, and apoptosis (programmed cell death). The discovery of these diverse and important roles of mitochondria has stimulated much current research on the biochemistry of this organelle. Principle 5 (7 of 7) The transmembrane flow of protons back down their electrochemical gradient through specific protein channels provides the free energy for synthesis of ATP. This process is catalyzed by a membrane protein complex (ATP synthase) that couples proton flow to phosphorylation of ADP. Uncoupled Mitochondria in Brown Adipose Tissue Produce Heat brown adipose tissue (BAT) = adipose tissue in newborn mammals that functions to generate heat through fuel oxidation uncoupling protein 1 (UCP1) = a long-chain fatty acid/H+ symporter that provides a path for protons to return to the matrix without passing through the FoF1 complex – results in energy of oxidation being dissipated as heat hydrolysis of phosphocreatine also liberates heat Two Mechanisms of Mitochondrial Thermogenesis Hibernating Animals hibernating animals depend on the activity of uncoupled BAT mitochondria to generate heat during their long dormancy Clicker Question 32 Which statement regarding uncoupling protein 1 (UCP1) is false? A. It is found in brown adipose tissue. B. It forms proton-conducting pores in the inner mitochondrial membrane. C. It provides a path for protons to return to the matrix without passing through the FoF1 complex. D. It allows for ATP generation in addition to heat formation. Clicker Question 32, Response Which statement regarding uncoupling protein 1 (UCP1) is false? D. It allows for ATP generation in addition to heat formation. UCP1, an uncoupling protein in the mitochondria of brown adipose tissue, causes the energy conserved by proton pumping to be dissipated as heat by providing an alternative route for protons to reenter the mitochondrial matrix. Because the protons passing through UCP1 bypass the FoF1 complex, ATP is not generated. Principle 1 (4 of 5) Mitochondria play a central role in eukaryotic aerobic metabolism. ATP production is not the only important mitochondrial function. Mitochondria play host to the citric acid cycle, the fatty acid β-oxidation pathway, and the pathways of amino acid oxidation. The mitochondria also act in thermogenesis, steroid synthesis, and apoptosis (programmed cell death). The discovery of these diverse and important roles of mitochondria has stimulated much current research on the biochemistry of this organelle. Mitochondrial P-450 Monooxygenases Catalyze Steroid Hydroxylations mitochondria are the site of biosynthetic reactions that produce steroid hormones – occur in steroidogenic tissues (adrenal gland, gonads, liver, and kidney) The Cytochrome P-450 Family cytochrome P-450 family = monoxygenases that catalyze a series of hydroxylation reactions to synthesize steroid hormones from a sterol – have a critical heme group cytochrome P-450 enzymes catalyze the reaction R–H + O2 +NADPH + H+ → R–OH + H2O +NADP+ Path of Electron Flow in Mitochondrial Cytochrome P-450 Reactions a flavoprotein and an iron-sulfur protein carry electrons from NADPH to the P-450 heme all P-450 enzymes have a heme that interacts with O2 and a substrate-binding site that confers specificity P-450 Enzymes in the ER of Hepatocytes catalyze similar reactions with hydrophobic compounds, including xenobiotics xenobiotics = compounds not found in nature but synthesized industrially Mitochondria Are Central to the Initiation of Apoptosis apoptosis (programmed cell death) = process in which individual cells die for the good of the organism can be triggered: – during normal embryonic development – in response to an external signal – by internal events (DNA damage, viral infection, oxidative stress from the accumulation of ROS, heat shock) Principle 1 (5 of 5) Mitochondria play a central role in eukaryotic aerobic metabolism. ATP production is not the only important mitochondrial function. Mitochondria play host to the citric acid cycle, the fatty acid β-oxidation pathway, and the pathways of amino acid oxidation. The mitochondria also act in thermogenesis, steroid synthesis, and apoptosis (programmed cell death). The discovery of these diverse and important roles of mitochondria has stimulated much current research on the biochemistry of this organelle. Clicker Question 33 Which statement does NOT describe a function associated with mitochondria? A. In brown adipocytes, electron transport is uncoupled from ATP synthesis. B. They make xenobiotic compounds more soluble for excretion. C. They release cytochrome c in the process of apoptosis. D. They are responsible for the production of steroid hormones by cytochrome P-450 enzymes. Clicker Question 33, Response Which statement does NOT describe a function associated with mitochondria? B. They make xenobiotic compounds more soluble for excretion. The P-450 enzymes that make hydrophobic compounds, including xenobiotics, more soluble are found in the endoplasmic reticulum of hepatocytes. These enzymes catalyze reactions similar to the mitochondrial P-450 reactions. Role of Cytochrome c in Apoptosis permeability transition pore complex (PTPC) = a multisubunit membrane protein that opens to increase permeability Apaf-1 (apoptosis protease activating factor-1) = monomers that interact with cytochrome c molecules to form an apoptosome caspases = proteases involved in apoptosis 19.5 Mitochondrial Genes: Their Origin and the Effects of Mutations Mitochondrial Genes and Mutations 13 mitochondrial proteins are encoded by the mitochondrial genome and synthesized in mitochondria ~1,200 mitochondrial proteins are encoded by nuclear genes and imported into mitochondria Respiratory Proteins Encoded by Mitochondrial Genes in Humans Clicker Question 34 The human mitochondrial genome: A. is less susceptible to DNA damage than is nuclear DNA. B. is a linear chromosome of 16,569 base pairs. C. encodes 37 genes, 13 of which code for polypeptide chains. D. is inherited in two copies per mitochondrion, one of paternal lineage and the other of maternal lineage. Clicker Question 34, Response The human mitochondrial genome: C. encodes 37 genes, 13 of which code for polypeptide chains. A small proportion of human mitochondrial proteins, 13 in all, are encoded by the mitochondrial genome and synthesized in mitochondria. The mitochondrial genome is circular and less efficient at repairing DNA damage than the nuclear genome. Moreover, the mitochondrial genome is derived almost exclusively from the maternal lineage. Principle 2 (4 of 4) Mitochondria trace their evolutionary origin to bacteria. Over 1.45 billion years ago, an endosymbiotic relationship arose between bacteria and a primitive eukaryote or eukaryotic progenitor. Mitochondria are ubiquitous in modern eukaryotes, and their bacterial origin is evident in almost every aspect of their structure and function. Mitochondria Evolved from Endosymbiotic Bacteria mitochondria arose from aerobic bacteria that entered into an endosymbiotic relationship with primitive eukaryotes – endosymbiotic bacteria eventually became mitochondria the chemiosmotic mechanism likely evolved before the emergence of eukaryotes Respiration-Linked Extrusion of Protons Drives Other Processes uptake of extracellular nutrients against a concentration gradient occurs in symport with protons the rotary motion of bacterial flagella is driven by the transmembrane electrochemical potential Mutations in Mitochondrial DNA Accumulate throughout the Life of the Organisms defects in mtDNA occur over time because: – of the greatest exposure to ROS – the mtDNA replication system is less effective than the nuclear system at correcting mistakes and damage animals inherit essentially all mitochondria from the female parent Heteroplasmy and Homoplasmy heteroplasmy = different types of mitochondrial genomes within a cell – results in mutant phenotypes of varying degrees of severity homoplasmy = the mitochondrial genome is the same within a cell Clicker Question 35 Which statement is true about heteroplasmic cells (and tissues) in which some of the mitochondria have a gene mutation? A. All of the cells (and tissues) will have the mutant phenotype. B. All of the cells (and tissues) will have the wild-type phenotype. C. Cells (and tissues) containing mostly wild-type mitochondria will have the wild-type phenotype. D. Cells (and tissues) containing mostly wild-type mitochondria will have the mutant phenotype. Clicker Question 35, Response Which statement is true about heteroplasmic cells (and tissues) in which some of the mitochondria have a gene mutation? C. Cells (and tissues) containing mostly wild-type mitochondria will have the wild-type phenotype. Cells (and tissues) containing mostly wild-type mitochondria are essentially normal. Other heteroplasmic cells have intermediate phenotypes, some almost normal, others (with a high proportion of mutant mitochondria) abnormal. Some Mutations in Mitochondrial Genomes Cause Disease mitochondrial encephalomyopathies = a group of genetic diseases that primarily affect the brain and skeletal muscle – inherited from the mother Leber hereditary optic neuropathy (LHON) = rare disease that affects the central nervous system – single amino acid substitution in Complex I causes the mitochondria to be partially defective in electron transfer from NADH to ubiquinone Myoclonic Epilepsy with Ragged- Red Fibers (MERRF) Syndrome myoclonic epilepsy with ragged-red fibers (MERRF) syndrome = caused by a mutation in the gene that encodes a tRNA specific for lysine skeletal muscle fibers of affected individuals have abnormally shaped mitochondria that sometimes contain paracrystalline structures Mitochondrial Donation mitochondrial donation = transplantation of a prospective mother’s nuclear genes into an enucleated ovum from a donor with healthy mitochondria – creates an ovum free of a mutation that would have led to a mitochondrial disease – raises ethical issues A Rare Form of Diabetes Results from Defects in the Mitochondria of Pancreatic β Cells mitochondrial defects in pancreatic β cells can limit ATP production when glucose levels are high compromises insulin release and gives rise to a form of type 2 diabetes Clicker Question 36 Which statement regarding mutations in mitochondrial DNA is false? A. Type 1 diabetes is due to dysfunctional β-cell mitochondria resulting from mutations in the mitochondrial tRNA genes. B. Impaired ATP production due to mutations in mitochondrial genes can result in human diseases. C. Mutations in mitochondrial genes accumulate through the life of an organism. D. An individual cell may have mitochondria that are not genetically identical to each other. Clicker Question 36, Response Which statement regarding mutations in mitochondrial DNA is false? A. Type 1 diabetes is due to dysfunctional β-cell mitochondria resulting from mutations in the mitochondrial tRNA genes. Type 2, not type 1, diabetes mellitus is common in individuals with mutations in the mitochondrial tRNALys or tRNALeu genes (although such cases make up a very small fraction of all cases of diabetes).