Biomembranes: Structure and Transport Mechanisms PDF
Document Details
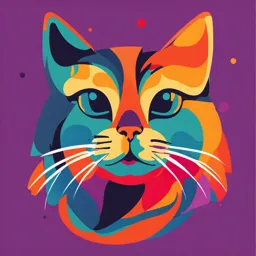
Uploaded by CongratulatorySwaneeWhistle
UWI, Mona
Dr. Icolyn Amarakoon
Tags
Summary
These lecture notes describe the structure and transport mechanisms of biomembranes, covering prokaryotic and eukaryotic membrane structures.
Full Transcript
BIOMEMBRANES STRUCTURE AND TRANSPORT MECHANISMS Dr. Icolyn Amarakoon Email: [email protected] 1 2 GENERAL OBJECTIVES Students should be able to: Understand the composition and structure of the biomembranes Understand how molecules and i...
BIOMEMBRANES STRUCTURE AND TRANSPORT MECHANISMS Dr. Icolyn Amarakoon Email: [email protected] 1 2 GENERAL OBJECTIVES Students should be able to: Understand the composition and structure of the biomembranes Understand how molecules and ions move across biomembranes 3 LEARNING OUTCOMES Describe the fluid-mosaic model of membrane structure and the factors that influence the permeability of lipid membranes. List the various types of lipids and proteins in the membrane and their functions. Predict the movement of molecules in diffusion and osmosis. Explain how molecules and ions get into and out of cells. Describe the structure and operation of specific examples of each class membrane transport. Explain how specific combinations of transport proteins in different subcellular membranes enable cells to carry out essential physiological processes 4 Membrane Structure: Prokaryotes Plasma membrane, but contain no internal membrane limited sub- compartments 5 Membrane Structure: Eukaryotes Partitioned into smaller organelles [largest is the nucleus]. each with one or more biomembranes. each organelle to carry out its characteristic cellular functions. 6 CELL ARCHITECTURE: Structure : Triacylglyceride 7 CELL ARCHITECTURE: Structure of Biomembranes R group 8 CELL ARCHITECTURE: Structure of Biomembranes Basic membrane structure are made up of mostly phospholipid 9 Structure of Biomembranes: Phospholipids Amphipathic (hydrophobic and hydrophilic) compounds Phosphatidylcholine Phosphatidylserine Phosphatidylethanolamine Phosphatidylinositol 10 Structure of Biomembranes: Phospholipid 11 Plasma membranes: Functions The lipid and proteins composition of a membrane determines its physical characteristics and functional properties. Isolate the cytoplasm from the external environment; acts as a permeability barrier Regulate the exchange of substances. helps the cell and the organism maintain a steady internal environment Communicate with other cells.- Signal transduction Identification : cell to cell recognition 12 Cellular membrane 13 Cellular membranes are fluid mosaics of lipids and proteins The fluid mosaic model, semi-permeable (selectively permeable), 14 double layer of phospholipids with embedded proteins Components of the Plasma Membrane Phospholipids are amphipathic: contain a hydrophilic head and a nonpolar hydrophobic tail, which creates a barrier Cholesterol - stiffens the membrane by connecting phospholipids Glycolipids - signal molecules Glycoproteins - have an attached chain of sugar (antibodies) Membrane carbohydrates are important in cell-cell recognition eg. the rejection of foreign cells by the immune system 15 Biomembranes: Lipid Composition and Structural Organisation Phospholipids in cells spontaneously form sheet like phospholipid bilayers. The hydrocarbon chains of the phospholipids in each layer, or leaflet, form a hydrophobic core 16 The Lipid Bilayer The lipid bilayer has two important properties: The hydrophobic core is an impermeable barrier that prevents the diffusion of water-soluble (hydrophilic) solutes across the membrane. The bilayer structure is stable. This is maintained by hydrophobic and van der Waals interactions between the lipid chains. 17 Three Classes of Lipids in Biomembranes A typical biomembrane is assembled from: phosphoglycerides sphingolipids steroids (cholesterol) All three classes of lipids are amphipathic molecules having a polar (hydrophilic) head group and hydrophobic tail. 18 Phosphoglycerides derivatives of glycerol 3- phosphate A typical phosphoglyceride consists of a hydrophobic tail composed of two fatty acyl chains esterified to the two hydroxyl groups in glycerol phosphate and a polar head group attached to the phosphate group. 19 2. Sphingolipids derived from sphingosine an amino alcohol with a long hydrocarbon chain, and contain a long- chain fatty acid attached to the sphingosine amino group sphingomyelin, the most abundant sphingolipid, phosphocholine is attached to the terminal hydroxyl group of sphingosine 20 3. Steroids: Cholesterol basic structure of steroids is a four-ring hydrocarbon it is amphipathic because its hydroxyl group can interact with water 21 Lipid Composition Influences the Physical Properties of Membranes Different types of membranes may have unique properties bestowed by its particular mix of lipids and proteins. Differences in lipid composition may also correspond to specialization of membrane function The ability of lipids to diffuse laterally in a bilayer indicates that it can act as a fluid 22 Lipid Composition of Membranes 23 Membrane fluidity The degree of bilayer fluidity depends on: the lipid composition structure of the phospholipid hydrophobic tails temperature 24 Factors that affect membrane fluidity Decreasing temperature: The temperature at which a membrane solidifies depends on the types of lipids it is made of. phospholipids with unsaturated hydrocarbon chains: The membrane remains fluid to a lower temperature if it is rich in phospholipids with unsaturated hydrocarbon tails. cholesterol: At relatively high temperatures [eg.at 37°C, the body temperature of humans] cholesterol makes the membrane less fluid by restraining phospholipid movement 25 Membrane Lipids Are Distributed Unequally in the Exoplasmic and Cytosolic Leaflets A characteristic of all membranes is an asymmetry in lipid composition across the bilayer. Although most phospholipids are present in both membrane leaflets, they are commonly more abundant in one or the other leaflet 26 Biomembranes: Protein Components and Basic Functions Membrane proteins are the mosaic part of the model Membrane proteins are defined by their location within or at the surface of a phospholipid bilayer The amount of proteins associated with biomembranes vary, depending on cell type and subcellular location. Some proteins are buried within the lipid-rich bilayer; other proteins are associated with the exoplasmic or cytosolic leaflet of the bilayer. 27 Protein Domains- Exoplasmic Protein domains on the extracellular surface of the plasma membrane generally: bind to other molecules, including external signaling proteins, ions, and small metabolites (e.g., glucose, fatty acids), and to adhesion molecules on other cells or in the external environment 28 Protein Domains- Cytosolic Domains within the plasma membrane, particularly those that form channels and pores, move molecules in and out of cells. Domains lying along the cytosolic face of the plasma membrane with a wide range of functions anchoring cytoskeletal proteins to the membrane triggering intracellular signaling pathways. 29 Proteins Interact with Membranes in Three Different Ways Membrane proteins can be classified into three categories: integral lipid-anchored, peripheral This is all on the basis of the nature of the membrane/protein interactions 30 Integral membrane proteins Also called transmembrane proteins Span a phospholipid bilayer and are built of three segments. The cytosolic and exoplasmic domains have hydrophilic exterior surfaces that interact with the aqueous solutions on the cytosolic and exoplasmic faces of the membrane. These domains resemble other water-soluble proteins in their amino acid composition and structure. 31 Integral membrane proteins cont. Most transmembrane proteins are glycosylated with a complex branched 32 sugar group attached to one or several amino acid side chains Lipid-anchored membrane proteins bound covalently to one or more lipid molecules. The hydrophobic carbon chain of the attached lipid is embedded in one leaflet of the membrane and anchors the protein to the membrane. The polypeptide chain itself does not enter the phospholipid bilayer. 33 Peripheral membrane proteins Bound to the membrane indirectly by interactions with integral membrane proteins or directly by interactions with lipid head groups. Peripheral proteins are localized to either the cytosolic or the exoplasmic face of the plasma membrane. 34 Cytoskeletal Filaments In addition proteins, are cytoskeletal filaments which are loosely associated with the cytosolic face, through one or more peripheral (adapter) proteins Associations with the cytoskeleton provide support for various cellular membranes they also play a role in the two-way communication between the cell interior and the cell 35 exterior. Secondary Structures in Transmembrane Proteins Soluble proteins exhibit hundreds of distinct localized folded structures, or motifs single-pass transmembrane protein contains only one membrane- spanning - helix. Eg Glycophorin A 36 Secondary Structures in Transmembrane Proteins Soluble proteins exhibit hundreds of distinct localized folded structures, or motifs multipass transmembrane proteins. Eg. Ion channels, G proteins 37 Covalently Attached Hydrocarbon Chains Anchor Some Proteins to Membranes In eukaryotic cells, several types of covalently attached lipids anchor some proteins to one or the other leaflet of the plasma membrane and certain other cellular membranes. In these lipid-anchored proteins, the lipid hydrocarbon chains are embedded in the bilayer, but the protein itself does not enter the bilayer 38 All Transmembrane Proteins and Glycolipids Are Asymmetrically Oriented in the Bilayer Lipid-anchored proteins are asymmetrically located with respect to the faces of cellular membranes. Each type of transmembrane protein has a specific orientation with respect to the membrane faces. 39 40 Proteins embedded in membrane serve different functions TRANSPORT: provide a hydrophilic channel across the membrane that is selective for a particular solute. shuttle a substance from one side to the other by changing shape. hydrolyze ATP as an energy source to actively pump substances across the membrane. 41 Protein Functions: Transport proteins Channel Proteins - have a hydrophilic channel that certain molecules or atomic ions use as a tunnel through the membrane. Aquaporins are channel proteins that facilitate the passage of water molecules through the membrane of certain cells 42 Protein Functions: Transport proteins Carrier Proteins- binding site on protein surface "grabs" certain molecules and pulls them into the cell, (gated channels). Carrier proteins basically hold on to their passengers and change shape in a way that shuttles them across the membrane 43 Protein Functions: Signal transduction Receptor Proteins - have binding site with a specific shape that fits the shape of a chemical messenger, (hormone). The external messenger (signaling molecule) may cause the protein to change shape, allowing it to relay the message to the inside of the cell, usually by binding to a cytoplasmic protein. 44 Protein Functions: Signal transduction receptor proteins that bind specific signaling molecules (e.g., hormones, growth factors, neurotransmitters), leading to various cellular responses. These proteins are critical for cell development and functioning. 45 Protein Functions: Cell- cell Recognition Proteins used as ID tags, to identify cells to the body's immune system. glycoproteins serve as ID tags that are specifically recognized by membrane proteins of other cells. 46 Protein Functions: Enzymatic activity A protein built into the membrane may be an enzyme with its active site exposed to substances in the adjacent solution. carry out metabolic reactions. several enzymes in a membrane are organized as a team that carries out sequential steps of a metabolic pathway. 47 Protein Functions: Intercellular joining Membrane proteins of adjacent cells may hook together in various kinds of junctions, such as gap junctions or tight junctions 48 Protein Functions: Attachment to the cytoskeleton and extracellular matrix (ECM). Microfilaments or other elements of the cytoskeleton may be non-covalently bound to membrane proteins, a function that helps maintain cell shape and stabilizes the location of certain membrane proteins. Proteins that can bind to ECM molecules can coordinate extracellular and intracellular changes. 49 Universal Functions of Plasma Membranes acts as a permeability barrier highly permeable to water but poorly maintain the proper ionic composition permeable to salts and small molecules and pH (≈7.2) of the cytosol. such as sugars and amino acids. membrane surrounding each organelle contains a unique set of proteins essential for its proper functioning 50 Universal Functions: Osmosis Due to osmosis, water moves across a semipermeable membrane from a solution of low solute (high water) concentration to one of high solute (low water) concentration until the water concentrations on both sides are equal. 51 Universal Functions: Osmosis Hypotonic solution: when cells are placed in a solution with a lower solute concentration than that of the cell interior, water flows into the cells, causing them to swell. 52 Universal Functions: Osmosis Isotonic solution: When most animal cells are placed in a solution with total concentration of solutes equal to that of the cell interior, there is no net movement of water into or out of cells. 53 Universal Functions: Osmosis Hypertonic solution: when cells are placed in a solution with a higher solute concentration than that of the cell interior, water flows out of cells, causing them to shrink. 54 Osmotic Pressure Causes Water to Move Across Membranes Osmotic pressure is the hydrostatic pressure required to stop the net flow of water across a membrane separating solutions of different compositions The osmotic pressure is directly proportional to the difference in the concentration of the total number of solute molecules on each side of the membrane 55 Osmotic Pressure Causes Water to Move Across Membranes Pure phospholipid bilayers are impermeable to water, but most cellular membranes contain water-channel proteins that facilitate the rapid movement of water in and out of cells. Such movement of water across the epithelial layer lining the kidney tubules of vertebrates is responsible for concentrating the urine. In higher plants, water and minerals are absorbed from the soil by the roots and move up the xylem; transpiration drives this movement of water. 56 57 TRANSPORT ACROSS MEMBRANES LEARNING OUTCOMES: 1. Briefly compare the three major classes of membrane proteins that increase the permeability of biomembranes. 58 2. Examine operation of the simplest type of transport protein to illustrate basic features of protein-mediated transport. TRANSPORT ACROSS MEMBRANES: Passive Transport Any small molecule can pass through an open aqueous opening 59 Passive Transport: Diffusion Simple Diffusion - water, oxygen and other molecules move from areas of high concentration to areas of low concentration, down a concentration gradient Facilitation Diffusion - diffusion that is assisted by proteins (channel or carrier proteins) 60 Passive Transport: Diffusion Few molecules cross membranes by passive diffusion Gases, such as O2 and CO2, and small, uncharged polar molecules, such as urea and ethanol, can readily move by passive (simple) diffusion across a membrane composed of pure phospholipid or of phospholipid and cholesterol. 61 Passive Transport: Diffusion The relative diffusion rate of any substance across a pure phospholipid bilayer is proportional to its concentration gradient across the layer and to its hydrophobicity and size charged molecules are also affected by any electric potential across the membrane. 62 TRANSPORT ACROSS MEMBRANES: Active Transport ATP-powered pumps (or simply pumps) are ATPases 63 Active Transport Active transport is used to take a molecule from a low concentration to a high concentration, which is abnormal and thus requires energy ATP-powered pumps (or simply pumps) are ATPases that use the energy of ATP hydrolysis to move ions or small molecules across a membrane against a chemical concentration gradient or electric potential or both. 64 Transport: Non-gated Ion Channels Channel proteins transport water or specific types of ions and hydrophilic small molecules down their concentration or electric potential gradients. Some ion channels are open much of the time; these are referred to as non-gated channels. 65 Non-gated Ion Channels An inside-negative electric potential (voltage) of 50–70 mV exists across the plasma membrane of all cells. In animal cells, the membrane potential is generated primarily by movement of cytosolic K+ ions through resting K+ channels to the external medium. non-gated K+ channels are usually open 66 Electrochemical Gradient If a transported substance carries a net charge, its movement is influenced by both its concentration gradient and the membrane potential, the electric potential (voltage) across the membrane. The combination of these two forces, called the electrochemical gradient, determines the energetically favorable direction of transport of a charged molecule across a membrane. 67 Gated channel proteins Most ion channels, however, open only in response to specific chemical or electrical signals; these are referred to as gated channels. 68 Transporters: Carriers & Channels Three types of transporters have been identified: Uniporters transport a single type of molecule down its concentration gradient via facilitated diffusion. Glucose and amino acids cross the plasma membrane into most mammalian cells with the aid of uniporters. 69 Uniporter: Glucose Transporter GLUT1 glucose concentration is higher in the extracellular medium (blood in the case of erythrocytes) than in the cell, GLUT1 generally catalyzes the net import of glucose from the extracellular medium into the cell. 70 Co-Transporters: Antiporters and Symporters When the transported molecule and co-transported ion move in the same direction, the process is called symport.When they move in opposite directions, the process is called antiport. Antiporters and symporters couple the movement of one type of ion or molecule against its concentration gradient with the movement of one or more different ions down its concentration gradient. 71 Co-transport by Symporters and Antiporters An important example of a cation co-transporter is the Na+/H+ antiporter, which exports H+ from cells coupled to the energetically favorable import of Na+. movement of a Na+ ion (the co-transported ion) into a cell across the plasma membrane, can be coupled to movement of the transported molecule (e.g., glucose) against its concentration gradient 72 SUMMARY - through the cell membrane Passive Transport Simple diffusion Diffusion of non polar, hydrophobic molecules Lipids HIGH LOW Concentration gradient Facilitated transport Diffusion of polar, hydrophilic molecules through a protein channel HIGH LOW Concentration gradient Active transport Diffusion against concentration gradient LOW HIGH Uses a protein pump ATP 73 Require ATP TRANSPORT SUMMARY 74 TRANSPORT ACROSS MEMBRANES: ACTIVE TRANSPORT MECHANISMS These proteins are called ATPases; they normally do not hydrolyze ATP into ADP and Pi unless ions or other molecules are simultaneously transported. All ATP-powered pumps are transmembrane proteins with one or more binding sites for ATP located on the cytosolic face of the membrane. 75 Different Classes of Pumps Exhibit Characteristic Structural and Functional Properties There are the four classes of ATP-powered pumps: P-class pumps, V-class proton pumps, F-class proton pumps, ABC superfamily The members of three classes (P, F, and V) transport ions only, whereas members of the ABC superfamily primarily transport small molecules. 76 ATP-Powered Pumps: P-class includes the Na+/K+ ATPase in the plasma membrane, which maintains the low cytosolic Na+ and high cytosolic K+ concentrations typical of animal cells. 77 ATP-Powered Pumps: P-class Certain Ca2+ ATPases pump Ca2+ ions out of the cytosol into the external medium; others pump Ca2+ from the cytosol into the endoplasmic reticulum or into the specialized ER called the sarcoplasmic reticulum, which is found in muscle cells. 78 ATP-Powered Pumps: P-class Another member of the P class, found in acid-secreting cells of the mammalian stomach, transports protons (H+ ions) out of and K+ ions into the cell. The H+ pump that generates and maintains the membrane electric potential in plant, fungal, and bacterial cells also belongs to this class 79 ATP-Powered Pumps: F-class & V-class The structures of these 2 class of ion pumps are similar to one another, but unrelated to and more complicated than P-class pumps. F- and V-class pumps contain several different transmembrane and cytosolic subunits 80 ATP-Powered Pumps: V-class V-class pumps generally function to maintain the low pH of plant vacuoles and of lysosomes and other acidic vesicles in animal cells by pumping protons from the cytosolic to the exoplasmic face of the membrane against a proton electrochemical gradient. 81 H+ Proton pump 82 ATP-Powered Pumps: F-class F-class pumps are found in bacterial plasma membranes and in mitochondria and chloroplasts. 83 ATP-Powered Pumps: F-class function to power the synthesis of ATP from ADP and Pi by movement of protons from the exoplasmic to the cytosolic face of the membrane down the proton electrochemical gradient. F-class proton pumps, commonly called ATP synthases are very important in ATP synthesis in chloroplasts and mitochondria. 84 ATP-Powered Pumps: F-class - mitochondria 85 ATP-Powered Pumps: F-class - chloroplast F-class proton pumps, commonly called ATP synthases are very important in ATP synthesis in chloroplasts and mitochondria. 86 ATP-Powered Pumps: ABC-superfamily contains more members and is more diverse than the other classes. Also called the ATP-binding cassette superfamily this class includes several hundred different transport proteins found in organisms ranging from bacteria to humans. 87 ATP-Powered Pumps: ABC-superfamily All ABC transport proteins share a structural organization consisting of four “core” domains: two transmembrane (T) domains, forming the passageway through which transported molecules cross the membrane. two cytosolic ATP-binding (A) domains. 88 ATP-Powered Pumps: ABC-superfamily Each ABC protein is specific for a single substrate or group of related substrates, which may be ions, sugars, amino acids, phospholipids, peptides, polysaccharides, or even proteins. 89 Ca ATPase 2+ Transporter The ER Ca2+ pump is called SERCA: Sarco(Endo)plasmic Reticulum Ca2+ ATPase 90 Ca2+ ATPase Pumps Ca2+ Ions from the Cytosol into the Sarcoplasmic Reticulum In skeletal muscle cells, Ca2+ions are concentrated and stored in the sarcoplasmic reticulum (SR) release of stored Ca2+ ions from the SR lumen into the cytosol causes contraction. 91 Ca2+ ATPase Transporter Ca2+ ATPase located in the SR membrane of skeletal muscle pumps Ca2+ from the cytosol into the lumen of the SR, thereby inducing muscle relaxation 92 Mechanism of Action of the Ca2+ ATPase: Step 1 When the protein is in the E1 conformation, two Ca2+ ions bind to two high-affinity binding sites accessible from the cytosolic side and 93 Mechanism of action of the Ca2+ ATPase: Step 2 An ATP binds to a site on the cytosolic surface. 94 Mechanism of action of the Ca2+ ATPase: Step 3 The bound ATP is hydrolyzed to ADP in a reaction that requires Mg2+, and the liberated phosphate is transferred to a specific aspartate residue in the protein, forming the high-energy acyl phosphate bond denoted by E1 ~ P. 95 Mechanism of action of the Ca2+ ATPase: Step 4 The protein then undergoes a conformational change that generates E2, which has two low-affinity Ca2+-binding sites accessible to the SR lumen. The Ca2+ ions spontaneously dissociate from the low-affinity sites to enter the SR lumen. 96 Mechanism of action of the Ca2+ ATPase: Step 5 The aspartyl-phosphate bond is hydrolyzed 97 Mechanism of action of the Ca2+ ATPase: Step 6 Dephosphorylation powers the E2 to E1 conformational change. E1 is ready to transport two more Ca2+ions. 98 P-class ion pumps All P-class ion pumps, regardless of which ion they transport, are phosphorylated on a highly conserved aspartate residue during the transport process. The operational model is applicable to all these ATP powered ion pumps. Na+/K+ ATPase H+/K+ ATPase Ca 2+ ATPase 99 Na /K + + ATPase Transporter Antiport pump 100 Na+/K+ ATPase Maintains the Intracellular Na + and K + Concentrations P-class ion pump present in the plasma membrane of all animal cells is the Na + /K + ATPase This ion pump is a tetramer of subunit composition 2β2. The amino acid sequence and predicted secondary structure of the catalytic -subunit are very similar to those of the muscle SR Ca2+ ATPase. The Na + /K + ATPase has a stalk on the cytosolic face that links domains containing the ATP-binding site and the phosphorylated aspartate to the membrane-embedded domain. 101 Na+/K+ ATPase The overall transport process moves three Na + ions out of and two K + ions into the cell per ATP molecule hydrolyzed 102 Mechanism of action of the Na + /K + ATPase Similar to that of the muscle calcium pump, except that ions are pumped in both directions across the membrane. 103 Mechanism of action of the Na + /K + ATPase In its E1 conformation, the Na + /K + ATPase has three high-affinity Na + binding sites and two low-affinity K + -binding sites accessible to the cytosolic surface of the protein. 104 Mechanism of action of the Na + /K + ATPase During the E1 to E2 transition, the three bound Na + ions become accessible to the exoplasmic face, and simultaneously the affinity of the three Na + - binding sites becomes reduced 105 Mechanism of action of the Na + /K + ATPase The three Na + ions, transported outward through the protein and now bound to the low-affinity Na + sites exposed to the exoplasmic face, dissociate one at a time into the extracellular medium despite the high extracellular Na + concentration. 106 Mechanism of action of the Na + /K + ATPase Transition to the E2 conformation also generates two high-affinity K + sites accessible to the exoplasmic face. during the E2 to E1 transition, the two bound K + ions are transported inward and then released into the cytosol. 107 Mechanism of action of the Na + /K + ATPase Certain drugs (e.g., ouabain and digoxin) bind to the exoplasmic domain of the plasma-membrane Na + /K + ATPase and specifically inhibit its ATPase activity. The resulting disruption in the Na + /K + balance of cells is strong evidence for the critical role of this ion pump in maintaining the normal K + and Na + ion concentration gradients. 108 Na+ /Glucose Symport Trans-epithelial transport: In the example shown, 3 carrier proteins accomplish absorption of glucose & Na+ in the small intestine. 109 Na + /Glucose Symport The Na+ pump, at the basal end of the cell, keeps [Na+] lower in the cell than in fluid bathing the apical surface The Na+ gradient drives uphill transport of glucose into the cell at the apical end, via glucose-Na+ symport. [Glucose] within the cell is thus higher than outside 110 Inhibitors of the Na+/K+ Pump: Digitalis Digitalis is a mixture of cardiotonic steroids cardiotonic steroids – strong effect on heart Increases the force of contraction of the heart Inhibit dephosphorylation of the phosphorylated form of ATPase on the extracellular face of the membrane Leads to higher Na+ in the cytosol Diminished Na+ gradient leads to slower exclusion of Ca 2+ by Na-Ca exchanger (antiporter) Increase in intracellular levels of Ca 2+ enhances the ability of the cardiac muscle to contract 111 Na+-Linked Antiporter Exports Ca2+ from Cardiac Muscle Cells In cardiac muscle cells a three-Na+/one-Ca2+antiporter, rather than the plasma membrane Ca2+ ATPase. plays the principal role in maintaining a low concentration of Ca2+ in the cytosol By lowering cytosolic Ca2+ operation of the Na+/Ca2+ antiporter reduces the strength of heart muscle contraction 112 Na+-Linked Antiporter Exports Ca2+ from Cardiac Muscle Cells Because of their ability to increase the force of heart muscle contractions, inhibitors of the Na+/K+ ATPase are widely used in the treatment of congestive heart failure. 113 Inhibitors of the Na+/K+ Pump: Ouabain Ouabain a Somali toxin used for ‘arrow poison’ Binds to the form of the Na+/K+ ATPase that is open to the extracellular side Locks in Na+ and prevents the change in conformation necessary for transport of ions increase in intracellular sodium reduces the activity of the sodium-calcium exchanger, which pumps one calcium ion out of the cell and three sodium ions into the cell down their concentration gradient 114 H+ ATPases Transporter V-class proton pumps 115 V-Class H+ ATPases Pump Protons Across Lysosomal and Vacuolar Membranes All V-class ATPases transport only H+ ions. These proton pumps, present in the membranes of lysosomes, endosomes, and plant vacuoles, function to acidify the lumen of these organelles. 116 Inhibitors of H+ ATPases Pump Proton pump inhibitors act by irreversibly blocking the H+/K+ ATPase system eg. gastric proton pump. Proton pump inhibitors promote healing of ulcers. Gastric acid secretion is a useful therapeutic principle for the treatment of ulcer of the upper GI tract. They are also useful in treating conditions that cause excessive stomach acid secretion. 117 ABC- superfamily Transporters ABC (ATP-Binding-Cassette) transporters 118 ABC Transporters The very large and diverse ABC superfamily of transport proteins contain: two transmembrane (T) domains two cytosolic ATP-binding (A) domains. 119 ABC Transporters The T domains, each built of six membrane-spanning - helices, form the pathway through which the transported substance (substrate) crosses the membrane and determine the substrate specificity of each ABC protein. 120 ABC Transporters Bacteria grow in soil or pond water where the concentration of nutrients is low, ABC transport proteins enable the cells to import nutrients against substantial concentration gradients eg E. coli histidine permease use the energy released by hydrolysis of ATP to transport specific amino acids, sugars, vitamins, or even peptides into the cell. 121 ABC Transporters The human genome contains 48 genes for ABC transporters. CFTR- the cystic fibrosis transmembrane conductance regulator TAP-the transporter associated with antigen processing ABC transporters that pump chemotherapeutic drugs out of cancer cells 122 ABC Transporters: CFTR Cystic fibrosis is a genetic disease: Faulty Cl- channels keep Cl- and Na+ in the cells that line the airways. Normally chloride ions(Cl-) move out of the cells lining the airways through the chloride channels. Na + follow the Cl- out of the cells due to the electrochemical gradient created. A hypoosmotic condition is created compared to the lumen of the airways. H2O moves out of the cells and blood stream by osmosis and into the airways, thinning the mucus. In cystic fibrosis the Cl- channel does not function properly so Cl- and Na+ remains in the cell. The cells are hyperosmotic compared to the lumen of the airways thus water remains in the cells and bloodstream. Without water passing into the airways, the mucus becomes thick and clogs the airways 123 ABC Transporters- MDR proteins Specialised for pumping small compounds [drugs and toxins] out of eukaryote cell. P-glycoprotein (P-gp): pump drugs from cytoplasm. P-glycoprotein is overexpressed in human cancer cells makes the cells resistant to a variety of drugs used in cancer chemotherapy a phenomenon known as multi-drug resistance. Currently testing drugs that inhibit P-gp for treatment of certain types of highly drug-resistant cancers 124 ABC Transporters 125 ABC Transporters- Blood-brain Barrier many drugs are not efficiently delivered to the brain, ABC transporters present in the plasma membranes of brain capillary endothelial cells pump a wide range of drugs back out into the blood. carriers bind to molecules in a specific manner. It is still unclear how P-gp specifically binds to drugs that are structurally unrelated Need to alter the permeability of the blood-brain barrier to drugs 126 Causes of defects in ABC Transporters Genetic diseases such as: Cystic fibrosis Tangier disease Retinal degeneration Anemia Liver failure 127 Additional Resources Membranes http://themedicalbiochemistrypage.org/membranes.php https://youtu.be/xHIzfkbj82U 128