B1100 Cell Biology Past Paper PDF
Document Details
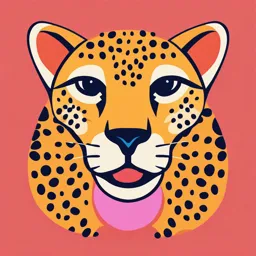
Uploaded by AstonishedJasper9501
Lebanese University
Pr Ziad ABDEL-RAZZAK
Tags
Summary
This document looks like part of a cell biology textbook, covering plasma membranes and other cell membranes. It discusses the chemical composition and structure of cell membranes, focusing on lipids, proteins, and carbohydrates. The document likely contains diagrams and figures to illustrate concepts.
Full Transcript
53 CH 4. PLASMA MEMBRANE AND OTHER CELL MEMBRANES The term "cell membranes" or biomembranes refers to all membranes that are found in a cell, including the plasma membrane, the nuclear membranes, the ER membrane, the Glogi apparatus membrane, vacuole membrane, plastids and...
53 CH 4. PLASMA MEMBRANE AND OTHER CELL MEMBRANES The term "cell membranes" or biomembranes refers to all membranes that are found in a cell, including the plasma membrane, the nuclear membranes, the ER membrane, the Glogi apparatus membrane, vacuole membrane, plastids and mitochondrial membranes,... In fact, inside the cell there are membranes (named cytomembranes) which delimit several specific compartments and separate them from their surrounding environments. All cell membranes are visible by EM but not by LM. They are less developed (less complex) in prokaryotes than in eukaryotes which are Membrane I characterized by complex cytoplasm (a) E compartmentalization. Cell membranes play, however, crucial functions in both eukaryotes and prokaryotes. The plasma membrane (or plasmalemma, cytoplasmic membrane) is responsible for cell integrity since it separates the cytoplasm from the surrounding medium. If plasma membrane is seriously damaged the cell will die. The other membranes in a cell also Hydrophobi tails delimit organelles such as ER, Golgi body, lysosomes and mitochondria, and separate them from their surrounding medium, the cytosol. It is important to Polar head remember that these membranes are not watertight groups and therefore do not completely isolate the compartments from their surrounding media. Cell membranes play not only physical functions, but also many biochemical ones. The plasma membrane is the site of many biochemical reactions and physiological processes such as signal transduction, phosphorylation and cell respiration in prokaryotes. Plasma membrane is also involved in communication and interaction between a cell and its neighboring cells. d All cell membranes have selective and controlled permeability since they regulate passage of solutes (soluble compounds such as ions and molecules) between the two compartments that are separated by a membrane. In other words, cell membranes do not Figure 4.1: Red blood cell membrane allow passage of any particle at any time. Certain seen by TEM (a), the corresponding particles can pass across membranes if the cell allows model scheme of Gorter and Grindel them, while others can pass regardless of membrane (b), the model of Danielli and Davson (c) and focus on the trilaminar permission. Passage of solutes across membrane appearance of plasma membrane (d). may be active or passive (details at the end of this chapter). The selective and controlled permeability of plasma membrane is responsible for their electrical polarity or electric potential. In general, due to the properties of lipids that form the membranes, (explained in Ch 2. VI. 3. ), most hydrophobic molecules can easily pass across the lipid bilayer whereas hydrophilic molecules require channels or transporters to cross the membrane. Channels and transporters are proteins integrated in the membrane. Transportation of large macromolecules can not be achieved through channels, it rather Lebanese University-FS1. B1100 - Cell Biology by Pr Ziad ABDEL-RAZZAK (2022) 54 occurs by vesicles of endocytosis, phagocytosis and exocytosis (details at the end of this chapter). Regarding composition of cell membranes, the main chemical components that form their basic structure are phospholipids (sphingosine-derived and glycerol-derived). Glycolipids also occur in the membrane, precisely in the outer leaflet and form a part of a cell antigens (especially gangliosides). In addition, there may be steroids (e.g. cholesterol) in the cell membranes of many eukaryotes. Thus, lipids form the basic structure of cell membranes. In addition to lipids, cell membranes comprise proteins (responsible for most membrane functions) and carbohydrates linked to proteins or to lipids. It is common to have abundant carbohydrates at the outer layer of the plasma membrane of many cell types, forming a coat to the cell named "glycocalyx". The glycocalyx (10 to 15 nm in thickness 12 ) protects the cell against proteolytics enzymes. It also plays several important roles in endocytosis, intercellular adhesion and cell-cell recognition. It provides a negative charge to the surface of many cell types. All components that form cell membranes (lipids, proteins and carbohydrates) show significant quantitative and qualitative differences between cell membranes of different cell types (see Table 2.2). I. THE LIPID BILAYER The components of cell membranes (lipids, proteins, and carbohydrates) were discovered by chemical and biochemical analyses. However, the structure of the membrane and the way its components are arranged together remained ambiguous for a long time. In 1925, a model of lipid bilayer was proposed by Gorter and Grindel who were studying the organization of red blood cell membrane. Their model was based on the principle of hydrophobic interaction between amphipathic lipids. They suggested that the polar heads of lipid molecules are directed inwardly to the cytosol and outwardly, whereas nonpolar tails of fatty acids are directed to one another (Figure 4.1). As previously explained, lipids are arranged in bilayers in order to avoid contact between hydrophobic tails and water in the surrounding aqueous solution (Figure 2.22); hydrophobic interaction is therefore the main force that stabilizes lipid bilayer structure of cell membranes. However, the model of Gorter and Grindel is silent regarding the organization of proteins in the membrane. In 1935, Danielli and Davson proposed a model (Figure 4.1) in which proteins are adsorbed at the two polar faces of the membrane and may span the membrane forming a Chanel. The lipid bilayer model was confirmed by other studies using TEM such as the freeze-fracturing technique (not explained here). Confirmation of the lipid bilayer model came from investigations by Robertson who examined slices of tissue stained with osmium tetroxide (not explained P riph ral m mbran prot in here) by TEM and remarked a Figure 4.2: The fluid mosaic model represents membrane structure and properties. 12 Thicker in front of microvilli. Lebanese University-FS1. B1100 - Cell Biology by Pr Ziad ABDEL-RAZZAK (2022) 55 trilaminar appearance of membranes. In fact, any cell membrane stained with osmium tetroxide (a polar molecule) and observed by TEM consists of two outer dark lamina referred to as osmophilic lamina (because they have affinity to osmium tetroxide) and a central clear one named osmophobic lamina (part "a" and "d", Figure 4.1 ). The trilaminar appearance corresponds to different affinities of the hydrophobic and hydrophilic regions of the bilayer to osmium tetroxide and does not mean the presence of three lipid layers. The hydrophilic heads of lipids bind osmium tetroxide while the hydrophobic tails do not. All cell membranes are lipid bilayers, but their thickness is variable depending on fatty acids and protein composition. The thickness of the three described lamina is therefore variable according to the observed membrane. The average thickness of an osmophilic lamina is about 20 to 25 A, whereas thickness of the osmophobic lamina is about 25 to 35 A. The total thickness of the set of the three lamina ranges between 65 and 85 A. II. FLUID-MOSAIC MODEL 1. Definition and protein types The diverse models that had been proposed by many scientists to schematize a cell membrane structure failed in giving complete and logical description of their organization. Singer and Nicolson came with a new model describing both membrane structure and properties. It is the "fluid-mosaic model" that can be summarized in one scheme depicted in Figure 4.2. According to the "fluid-mosaic model", a cell membrane consists of a bimolecular lipid layer (outer layer named "E" for exoplasmic, inner layer named "P" for protoplasmic) which is composed of phospholipids with little amounts of neutral fats and steroids and whose surface is interrupted by proteins that occur in a mosaic manner. The irregular distribution of proteins in the lipid bilayer is the mosaic property. The membrane proteins are organized in the three following types (Figure 4.3): - peripheral or extrinsic proteins that are weakly (not covalently) attached at the inner and outer polar surfaces of the lipid bilayer. Protein adhesion to lipids or other membrane proteins is mediated by weak bonds. Peripheral Protein Linked by (Binds integral proteins) 'sticky fingers' or 'greasy feet' Periphera protein Transmembrane (B inds lipids) (Integral) Protein Figure 4.3: Covalent anchoring of peripheral membrane proteins to the lipid bilayer (left) and diverse possibilities of membrane protein topography (right). - peripheral or extrinsic proteins that are tightly (covalently) attached at the inner and outer polar surfaces of the lipid bilayer via lipid molecules. Such proteins are covalently bound to a lipid (phospholipids or simple fatty acid) which is anchored in the membrane. Trimeric G proteins are examples of extrinsic proteins anchored by a fatty acid in the lipid bilayer at the cytosolic side. Lebanese University-FS1. B1100 - Cell Biology by Pr Ziad ABDEL-RAZZAK (2022) 56 - integral or intrinsic proteins that penetrate the membrane partially through one layer (monotopic) or entirely through the two layers (polytopic) by means of one or more helix segments. Integral proteins are the most abundant in the membrane. In fact, single-pass polytopic (also named bitopic) membrane proteins cross the whole membrane only once, while multi-pass membrane proteins cross it several times as depicted in Figure 4.2. All intrinsic protein must have at least one stretch (about 15 to 20 "aa" in length) rich in hydrophobic "aa" in order to be stable inside the hydrophobic region of the lipid bilayer. Such stretches usually adopt a-helix structure which interacts with the hydrophobic tail of membrane lipids. Certain membrane proteins have more than one hydrophobic segment and the polypeptide spans the membrane several times (Figure 4.2, Figure 6.5). Many of the proteins in a membrane are connected to, or interact with cytoskeleton components as well as with other cytosolic proteins or extracellular matrix components. Such connection may be constitutive (permanent) or induced by certain signals such as binding of a ligand (e.g. hormone) to its receptor which is an integral membrane protein. There are hundreds of integral membrane protein types that occur in eukaryotes. Integral membrane proteins are grouped into families depending on their functional properties and their "aa" sequences. The main families are: i- lmmunoglobulins superfamily (lgSF) which is involved in immune functions as well as in calcium- independent cell-cell adhesion. For instance, immunoglobulins mediate interaction between o antigen lymphocytes and macrophages during immune response. Antibodies and T-cell receptors belong also to this family. ii- Cadherins form a family of integral membrane glycoproteins that mediate calcium-dependent cell A antigen adhesion as well as communication with the surrounding medium which is either a cell or an extracellular matrix). They are found on the surface of many animal cell types. iii- Selectins form a family of integral membrane glycoproteins whose activity is also calcium-dependent. B antigen Figure 4.4: Glycolipids that They are characterized by their ability to recognize and determine the three blood bind specific sugar motifs (arrangements) protruding from groups. cell surface. For instance, selectins mediate temporary interaction between circulating leukocytes and endothelial cells of blood vessel walls at the site of inflammation thereby allowing leukocyte infiltration to the damaged tissue. lmmunoglobulins, selectins and cadherins belong to the broad family of CAM (cell adhesion molecules). iv- lntegrins belong to SAM family (substrate adhesion molecules) that bind to the basement membrane components. Also, more heterogenous proteins (extrinsic, present in the basement membrane) such as laminins and fibronectin belong to SAM family and contribute to epithelium attachment to the basement membrane. In addition to lipids and proteins, carbohydrates are found in the plasma membrane of many eukaryotic cells and may represent up to 10% of its weight. For instance, red blood cell membrane contains 52% proteins, 40% lipids and 8% carbohydrates. The fluid-mosaic model emphasizes their presence. In fact, most carbohydrates are covalently bound to certain membrane proteins (as glycoproteins) and lipids (as glycolipids) and are exposed at the outer surface of the plasma membrane. One or more sugar motifs occur in glycolipids and glycoproteins. In the other cell membranes, when carbohydrates are present, they are exposed away from the cytosol (the lipid layer located away from the cytosol). Lebanese University-FS1. B1100 - Cell Biology by Pr Ziad ABDEL-RAZZAK (2022) 57 Glycolipids, glycoproteins and proteins are crucial to cell physiology and metabolism as well as to cell identity at the molecular level. For instance, the various blood groups are determined by different glycolipids present in the red blood cell membrane (Figure 4.4). The difference between blood group antigen (A, B and 0) is a difference in few sugar motifs (Figure 4.4). Membrane proteins are responsible for many catalytic and structural functions. For instance, many proteins are transporters or channels, some proteins are connected to cytoskeleton molecules, and others are receptors to extracellular ligands (e.g. hormones, extracellular matrix). In summary, all cell membranes and prokaryotic membrane have the same structure of lipid bilayer with proteins and obey the fluid-mosaic model principle although they differ in terms of fine composition and functional properties. 2. Membrane asymmetry of proteins and lipids Proteins in a membrane are not uniformly distributed between the two lipid layers. There are some protein types that occur in the outer leaflet (lipid layer), absent in the inner one and vice versa (Figure 4.2). Moreover, certain protein types are present in both layers but in different quantities. This fact is referred to as asymmetry of protein distribution and asymmetry of the membrane as a whole. Asymmetry is logical since the location of a particular protein type is associated to its function. Simply, a protein must be present where it must perform its role. For instance, a protein that mediates attachement to the extracellular matrix must be located at the outer lipid layer. Those that interact with the cytoskeleton are directed inwardly. Most proteins that have disulfide bonds are found at the extracellular side of the membrane. Similarly to proteins, lipids are distributed asymmetrically between the outer and the inner leaflets (Figure 4.5). Also, carbohydrate motifs are always directed outwardly in the plasma membrane. Therefore, all cell membranes are asymmetrical since proteins, lipids and carbohydrates (when present) of the outer layer differ quantitatively and qualitatively from those of the inner one. For example, the outer half of the enterocyte membrane contains far less protein than does the inner half. Another example of asymmetry is with the human red blood cell membrane. In this cell type, the majority of phosphatidylcholine occurs in the outer leaflet, whereas the majority of phosphatidylethanolamine is present in the inner leaflet. Phosphatidylserine, which is negatively charged on its polar head, is more abundant in the inner half (Figure 4.5). Consequently, the inner leaflet of the red blood cell membrane has more negative charges than the outer one. Out ideof ell Completely Unsaturated Unsaturated PhosphatidyI serine PhosphatidyIinositoI Phosphatidyet I hanoIamine saturated fatty acids fatty acids fatty acid with one with two Cytosol tails double bond double bonds Figure 4.5: Lipid asymmetry in a membrane (left) and effect of unsaturation of fatty acids on membrane fluidity (right). Membrane asymmetry concerns the nature of the polar heads of lipids but also the fatty acids themselves. The fatty acids are not evenly distributed in terms of quantity and Lebanese University-FS1. B1100 - Cell Biology by Pr Ziad ABDEL-RAZZAK (2022) 58 quality in the two layers. Degree of asymmetry varies considerably with cell types and even in the same cell from one cell membrane to another. Since phospholipids and glycolipids can not easily make flip-flop movement (from one layer to the other), their asymmetry must have been achieved by specific enzymes named flipases that intervene to displace them, after their synthesis, from a membrane lipid layer to the other. 3. Fluidity of membranes - mobility of lipids and proteins a. Factors affecting membrane fluidity When observed in electron microscopy images, membranes appear as static structures. In reality, they are highly dynamic since there is a continuous flux movement of addition and removal of components. Moreover, the components (proteins and lipids) that are in the membrane are in perment motion because there are no covalent bonds among them, rather hydrophobic interaction holds them together. So, both membrane proteins and lipids are in continuous movement within the membrane since it is fluid 13. Membrane fluidity is due to the fact that lipids and proteins are maintained together by hydrophobic interactions not by covalent bonds. In addition, fluidity is influenced by length of fatty acids, their unsaturation degree, the presence of cholesterol and temperature. Actually, the presence of double bonds in the hydrocarbon chain of fatty acids (of phospholipids) increases fluidity of the lipid bilayer. This is because when fatty acids are completely saturated their assembled structure tends to behave as a rigid crystalline array (Figure 4.5). Cholesterol acts as a bidirectional regulator of membrane fluidity. At high temperatures, it decreases its fluidity, whereas at low temperatures it intercalates between the phospholipids promoting fluidity by preventing phospholipids clustering and hardening. Fatty acids with longer hydrocarbon chain cause a Uuman decrease in membrane fluidity. Temperature is another parameter on which fluidity depends since lipids fluidity increases as temperature increases and vice versa (fats and waxes melt by heating). Adequate fluidity is an important property of cell membranes because if a membrane were too fluid, its components would dissociate from one another and therefore membrane structure would be lost. If a membrane were too viscous, membrane functions that rely on interactions among its components (lipids and proteins) would be blocked since viscosity prevents free interaction and movement. In conclusion, all cells must keep their membranes at the suitable fluidity rate by adjusting fatty acid unsaturation degree and cholesterol amount. For instance, cells grown at temperature below 37 ° C will promote activity of unsaturating enzymes in order to compensate loss of fluidity caused by low temperature and maintain the suitable fluidity. Plants in general are rich in unsaturated fatty acids in order to keep adequate fluidity when temperature drops at night and during winter. Figure 4.6: Demonstration of Plants that thrive in winter have a high percentage of lateral diffusion of membrane unsaturated fatty acids in their membranes. proteins in a hybrid cell. 13 Fluidity is the opposite of viscosity. Lebanese University-FS1. B1100 - Cell Biology by Pr Ziad ABDEL-RAZZAK (2022) 59 b. Mobility of membrane components Mobility of proteins was demonstrated by Frye and Edidin, who used Sendai Virus to facilitate formation of heterokaryon in cell culture. A heterokaryon is a large hybrid cell resulting from fusion between two diploid cells derived from distinct species, e.g. human and mouse. Their experiment consists of labeling human and mouse cell surface antigen with two different fluorochromes (different flurescence colors) (Figure 4.6). Such labelling helps distinguish human and mouse membrane proteins using a fluorescence microscope. After tagging of the antigens with their respective dies, fusion of cells is induced and the heterokaryon is examined under a Parench mal fluorescence microscope. The authors noticed ce ly l that, immediately after fusion, human and mouse membrane proteins (antigens) are located at Bile canalicu us opposite poles, but less than an hour after l fusion, they both become uniformly distributed throughout the entire heterokaryon membrane. This is a clear evidence of the occurrence of lateral movement of proteins in the lipid bilayer. a ll y ______::,...i; :.:.:,._· ··_-__,. ··_,~·..;__:: :l:,;;... -.,;.._- C pi ar So, proteins are not fixed at precise positions within the membrane, rather they are able to Api al d main move laterally in the same plane. Labeling of membrane phospholipids followed by photobleaching 14 can demonstrate I movement of lipids which exhibit higher degree......----+-Tight of mobility than do proteins. For instance, a jun tion phospholipid molecule can move laterally about 2µm per second. Finally, spontaneous movement of membrane proteins and lipids 0 through the bilayer is exclusively lateral (within the same layer) since the hydrophilic part of both proteins and lipids cannot easily pass across the hydrophobic zone of the membrane. The "flip flop" movement between the two layers is almost impossible without intervention of flipases. From the previous experiment it seems that ti u protein movement is always random. Actually, Figure 4.7: Three poles (faces) of the movement of certain proteins may be controlled hepatocyte (top) and of the enterocyte by the cytoskeleton components. The (bottom). See text for details. connection between integral proteins and the cytoskeleton (already explained in Figure 4.2) may be responsible either for immobility of a protein or for its movement in a directed path in the membrane. Moreover, the interactions between extracellular matrix or basal lamina (basement membrane) and membrane proteins, and among membrane proteins themselves in the same area of a membrane, also contribute to control of their movement. Finally, movement of proteins is restricted to one single pole of the plasma membrane in the case of polarized plasma membrane (e.g. epithelial cell). In such a case, the presence of tight junctions (explained next in this chapter) limits protein movement. 14 It consists of staining the entire surface of the plasma membrane of an alive cell, bleaching of a small spot with a beam of light and then analyzing of recovery of labelling in the bleached area by movement of lipids from non bleached areas. Lebanese University-FS1. B1100 - Cell Biology by Pr Ziad ABDEL-RAZZAK (2022) 60 4. Polarization and functional domains The plasma membrane does not show the same detailed properties at all cell sides (d) r in n:ellular space 0.6µm (b) sealing trands of tight junc1ion l Microvilli proteins cytoplasmic half of lipid bilayer (e) cell 2 junction cell 1 space Linkage of protein - particles in adjacent cells Ro of protein claudin occludin particles 60nm L_J tight-junction proteins J./ l - ---'\ / Ba olateral domain (f} Figure 4.8: Diverse representation modes of the tight junction. (a) Side view of tight junction of enterocyte by freeze fracturing-TEM; (b) model showing cross section of tight junction; (c) TEM image of tight junction belt in a cross section; (d) model showing rows of interacting membrane proteins; (e) topology of the three involved proteins; (f) localization of the tight junction belt between the apical pole and the lateral one. even though it obeys the fluid mosaic model. In fact, the fine composition and function of the plasma membrane is usually not uniform throughout the entire cell surface. Most cell types show distinct plasma membrane poles characterized by distinct properties and functions. The cells have distinct plasma membrane poles characterized by the presence Lebanese University-FS1. B1100 - Cell Biology by Pr Ziad ABDEL-RAZZAK (2022) 61 of certain types of proteins and lipids that are associated with precise functions. For instance, all epithelia are composed of polarized 15 cells. The epithelial intestine cell has three poles (Figure 4.7), the apical, the lateral and the basal poles. These three poles differ by their functions and their molecular composition although they share many structural and functional properties. The apical pole is provided with the necessary transporters and channels for nutrients absorption from the intestine lumen. By contrast, the basal pole performs adhesion of the epithelium to the basement membrane and mediates the transport of nutrients to the underlying blood vessels. The lateral pole is involved in an adhesion role with the neighboring cells. Another example illustrating plasma membrane polarity is that of the parenchymal liver cell, the hepatocyte which has three different poles. Each hepatocyte is in contact with two different neighboring cell types and with a bile canaliculus (Figure 4.7). One pole of the hepatocyte membrane faces membranes of the capillary sinusoids (endothelial cells). Through this pole, various substances (such as sugars, "aa", insulin, gases...) are exchanged between bloodstream and hepatocytes. Therefore, this face of the membrane must contain specific molecules, enzymes and receptors involved in transportation of these substances. The second pole is the portion of the plasma membrane adjacent to the neighboring hepatocyte membrane. Across this pole two neighboring hepatocytes exchange many substances and perform junctions named desmosomes. The third membrane pole of hepatocyte is in contact with the bile canaliculi into which bile pigments and salts are continuously discharged by the hepatocyte and conducted to the gall bladder. Therefore, at this pole, the hepatocyte must have the necessary molecular equipment to secrete bile salts. Clearly, each of the three faces is distinguishable by its enzyme content and molecular composition. 5. Microdomains (lipid rafts) Microdomains of the plasma membrane (lipid rafts) consist of local differentiation of the membrane (size 70-350 nm). Specific phospholipids and raft proteins such as sphingomyelin, glycosphingolipids, kinase-like signaling proteins are gathered in form of stable rafts that are involved in signal transduction. Caveolae are a special type of lipid raft (50-100 nm) containing caveolin protein. It is a site where invaginations of the plasma membrane occur and play a role in endocytosis. Ill. SPECIALIZATION OF THE PLASMA MEMBRANE The plasma membrane frequently exhibits specialized junctional regions that play a role in cell-cell adhesion, cell-matrix adhesion and intercellular transport and communication. Without the diverse junction types, cells of a tissue would dissociate, and cell-cell/ cell-extracellular matrix communication would be impossible. Junctions involve membrane proteins (CAM and SAM families) and cytoskeleton components. They form during embryonic development and remain stable. Wound healing involves formation of junctions at any life stage. The junctional complex located near the apical pole of epithelia consists of three types of junctions (tight junction, intermediate junction and spot desmosome). Membrane proteins that are involved in physical adhesion among cells and between cells and the basement membrane, are often involved in communication and signaling at the same time. 15 It is a property other than electric polarity (plasma membranes are negatively charged inside, positively charged outside) Lebanese University-FS1. B1100 - Cell Biology by Pr Ziad ABDEL-RAZZAK (2022) 62 1. Tight junctions Tight junctions or zonula occludens is a actin filaments nside microvil/us kind of cell-cell adhesion characterized by microvilli extending from apical surface narrowed (even plugged) intercellular space. These junctions occur in form of belt at the boundary between the apical pole and the lateral one. The plasma membranes of neighboring cells fuse with one another at tight junction many points due to tight interaction between intrinsic membrane proteins (e.g. occludin, bundle of actin filaments Figure 4.8e) belonging to both membranes. In other words, tight junctions are formed by the lateral plasma attachment of rows of occludin belonging to membranes of adjacent the neighbouring membranes so that they epithelial cells function as two halves of a zipper (Figure 4.8f). Moreover, actin filaments of the cytoskeleton indirectly attach to occludin via other protein types (not shown in the figure) (A) basal surface that belong to the tight junction belt. Since tight junctions are associated with actin filament, they contribute to cell shape determination. The belt of the tight junction consists of several zipper-like structures that intersect one another and form a band at the cell actin cadherin circumference between a cell and its filament dimers anchor proteins (Bl surrounding neighbors. The belt is therefore Figure 4.9: Intermediate junctions forming a belt formed around each cell. The belt plugs the at the cell circumference. intercellular space and acts as a barrier to the flow of material between cells (Figure 4.8c). Zonula occludens occurs in all epithelia such as intestine (enterocytes) and in seminiferous tubules (among Sertoli cells). They also filaments occur between endothelial cells of brain blood capillaries. desmosome In addition to their adhesion role, they contribute to selective absorption of nutrients by intestine epithelium since they prevent passage of nutrients through the intermembrane space and obligate them to pass across the apical membrane surface whose permeability is selective. Finally, tight junctions are under complex control. Although they are very strong their dissociation may be triggered by certain immune cells that have to pass across epithelia or endothelia during the immune response. 2. Intermediate junctions The main function of intermediate junctions is adhesion of cells in many types of tissues. It is also named belt desmosomes or zonula adherens and occurs in many tissues, especially in epithelia forming a belt that Basal lamina hemidesmosome encircles the cell. This belt is located just below the tight Figure 4.10: Interconnected spot desmosomes and junction belt (Figure 4.9). Unlike the tight junction belt, the hemidesmosomes occur at the intermediate junction belt does not plug the lateral and basal poles, respectively. Lebanese University-FS1. B1100 - Cell Biology by Pr Ziad ABDEL-RAZZAK (2022) 63 intermembrane space. The two adjacent plasma membranes joined by belt desmosomes are separated by an intercellular space of 20 to 35 nm. However, the two plasma membranes of neighboring cells are linked together via their respective cadherins which are intrinsic membrane proteins that interact with each other through their extracellular domains. That is, the belt is the result of adhesion among cadherins which are integral proteins belonging to the plasma membranes of neighbouring cells. Cadherin interaction is calcium-dependent as previously described (Ch 4. II. 1. ). At the cytoplasmic face of the belt, each cadherin molecule is linked to a catenin molecule (named anchor proteins in the figure) that binds to actin filaments. Therefore, there are girdles of actin filaments in the cytosol placed against plasma membrane and anchored to catenins in this junction. The zone where actin filaments are appressed to the plasma membrane is dense and thick because it contains a complex network of protein filaments. The belt of actin is thus adhered to cadherins in the membrane via catenin. 3. Spot desmosomes Spot desmosomes or macula adherens may occur everywhere between two neighboring cells and, as their name indicates, they are punctual junctions (1 µm in diameter) forming button-like attachment points (Figure 4.10) that tighten cells in nearly all types of tissues. Spot desmosome which is thought to be the strongest junction occurs abundantly in tissues that are submitted to mechanical pressure such as the skin epithelium, the uterus and the cardiac muscle. At the level of a spot desmosome, width of the intermembrane space is about 20 to 35 nm and it contains interacting cadherin molecules (e.g. desmoglein and desmocollin) whose interaction is calcium-dependent (Figure 4.11). Similarly to the previous junction type, cadherins of a spot desmosome are intrinsic membrane proteins that belong to the plasma membranes of neighbouring cells. Internally, in each of the two neighboring cells, there is a discoid dense region lying against the plasma membrane, it is named cytoplasmic plaque. This plaque is composed of proteins that serve as anchorage point for looping intermediate filaments. Intermediate filaments are linked to cytosolic end of (a) : ane cadherins via two protein types: desmoplakin space and plakooglobin. Intermediate filaments radiate through the cytoplasm and connect to other desmosomes thereby making a 3D network of intermediate filaments that provide internal support to the cell. The 3D network in one cell connects with that in the neighbor cell by the spot desmosome. Therefore, a layer of epithelial cells is crossed by continuous 3-D network of intermediate filaments (keratine), which confers strength to Desmoglein ano Cytoplasmic plaque the entire tissue and resistance to pressure. desmocollin (cadherins) (plakoglobin, desmoplakins) 4. Hemidesmosomes (b) Intermediate filaments Cytoplasmic plaques Hemidesmosomes simply consist of half desmosomes and occur at the basal pole of epithelia (Figure 4.10) that lies at the basal lamina which is tightly linked to underlying connective tissue. Therefore, a hemidesmosome mediates attachement of epithelia to the underlying basement membrane. Therefore, hemidesmosomes are responsible for adhesion between epithelia Figure 4.11: (a) Scheme of spot desmosomes. (b) spot desmosome section view seen by TEM. Lebanese University-FS1. B1100 - Cell Biology by Pr Ziad ABDEL-RAZZAK (2022) 64 and connective tissue (e.g. adhesion of the epidermis to the basement membrane and the underlying dermis). A hemidesmosome consists of a dense plaque made up of plectin and lying against the inner surface of the basal plasma membrane. The intrinsic proteins that mediate attachment are integrins (rather than cadherins). A bundle of intermediate filaments (e.g. keratin) is attached to the plectin plaque and interacts with integrins and other transmembrane proteins which interact with laminins of the basal lamina. 5. Synapticjunctions They consist of contact points between axons of neurons and many cell types (e.g. muscle fibers, gland cells, nerve cells). Each axon hooks up its tip at a specific cell membrane making specific contact that requires highly faithful recognition between postsynaptic and presynaptic plasma membranes. More than 50 different members of cadherin family are involved in formation of synaptic connections. Although presynaptic and postsynaptic plasma membranes do not make direct contact and are separated by the synaptic cleft (20 to 50 nm in width), action potentials of nerve impulses are transmitted through them by release of chemicals (neurotransmitters). Actually, the change of the rest potential of the presynaptic cell membrane leads to change of permeability and release of neurotransmitters (e.g. acetylcholine) in the synaptic cleft. Neurotransmitters then bind to receptors (integral membrane proteins) at the postsynaptic cell membrane and change its permeability causing modification of its rest potential and generation of postsynaptic message. 6.Gapjunctions Gap junctions consist of pipeline structures that occur in clusters between neighboring cells. They are made up of integral proteins named connexins. Gap junctions play a key role in transportation and exchange of molecules and solutes between neighboring cells. Open Closed gapjunctio:n Connexoo (cell A) Cytoplasm Plasma membrane extracellular Plasma membrane Cytoplasm Connexoo (cell B) N·term Connexln {NIH2) Connexln structure C·term (COOH) Figure 4.12: Gap junction model. Six connexin subunits form a connexon (a channel). A connexion is a polytopic integral protein spanning the membrane 4 times. Rotation of connexons opens and closes them. PM: plasma membrane. Gap junctions occur in nearly all tissues except skeletal muscle cells and nerve cells. They are present however in the cardiac muscle and mediate the rapid propagation of nerve impulses through the whole heart muscle so that the whole muscle is synchronized and behaves as a single cell. In smooth muscles (e.g. esophagus) currents of ions through gap junctions mediate the consecutive contractions of the successive parts of the muscle Lebanese University-FS1. B1100 - Cell Biology by Pr Ziad ABDEL-RAZZAK (2022) 65 which results in a peristaltic contractile movement of the organ. This movement ensures swallowing process. Gap junctions are aqueous channels, which occur in clusters. They are made up of a group of six subunits of connexins 16, named connexon. These are pipeline-like structures that extend from the cytosol of one cell to that of the other. Connexons of adjacent cells are tightly linked by interaction through their extracellular domains in the intercellular space. At the level of connexon clusters, the space between two adjacent plasma membranes is therefore narrowed to about 3 nm (Figure 4.12). Each connexin is an integral polytopic protein having four a-helix transmembrane domains. The a-helix is hydrophobic except at one longitudinal side. Because all assembled connexin's hydrophilic sides are directed to the channel center, a hydrophilic channel of 1,5 to 2 nm of diameter is formed. Thus, polar molecules can easily pass through gap junctions provided Prtmary that their size is less Cell than 2 nm. For the Wall small molecules such as "aa" and monosaccharides, passage across opened gap junction is not selective. Communication across gap junctions Particles is controlled even for embedded in ER and the small plasma compounds. In fact, membrane the channels are not 1--'-il"'--- Desmotubule permanently opened -Cell wall since rotation of connexins (of a -Plasma connexon) in one membrane direction opens the channel and rotation Figure 4.13: Primary cell wall diagram (top) and plasmodesmata image by TEM in the opposite and its corresponding diagram (bottom). direction closes it (Figure 4.12). The decrease of pH and the increase of calcium concentration in the cytosol cause opening of the gap junction. The process is reversible upon pH and calcium concentration change. 7. Plant cell wall and plasmodesmata Cell wall of plants surrounds and envelopes the plasma membrane. Primary cell wall is made up of cellulose and a matrix which consists of hemicellulose, pectin and small quantity of glycoproteins. Proportions of these constituents are variable in a species-, cell type- and maturation-dependent manner. Cellulose is synthesized outside the cell by 16 Approximately 20 different connexins are known. Their expression is tissue-specific in order to avoid gap junctions between inapropriate cell types. Lebanese University-FS1. B1100 - Cell Biology by Pr Ziad ABDEL-RAZZAK (2022) 66 enzymes of the plasma membrane whereas matrix components are produced inside the cell by Golgi body (details in Ch 7. II. 2. ). The secondary cell wall is produced around mature cells and is mainly composed of lignin in addition to the previously named components. The previously described junctions are not present in plant tissues whose adhesion is mediated by the cell wall itself, particularly by pectin. However, there are cytoplasmic bridges between adjacent cells in order to allow communication and exchange of nutrients. Such bridges are named plasmodesmata and are important in the exchange of water, soluble salts and gases as well as soluble carbohydrates Figure 4.14: Microvilli of enterocytes. between neighboring cells. The cell wall is interrupted at the level of plasmodesmata where the plasma membrane of the first cell is continuous with that of the second one (Figure 4.13). The diameter of a plasmodesma is about 30 to 60 nm. In the center of the channel there is a membranous structure named desmotubule which is derived from the. tratifi d c lumnar pith lium SER. The presence of desmotubule iii narrows the opening through which molecules can pass (the annulus). Therefore, only molecules less than 1 kDa can easily pass. Nevertheless, it is possible that transportation of much larger molecules be done after dilation of plasmodesmatal pore. Plant cell wall shows specializations depending on tissues. That is the cell wall acquires new properties in some cell types. For instance, lignification is the impregnation of the cell wall by lignin in woody plant tissues to give them hardness. Lignin is mainly made up of phenol derivatives. Mineralization, which also confers hardness, is the impregnation of the cell wall by minerals such as calcium carbonate and silicium. Other specializations confer impermeability such as cutinization (cutin, the shiny cover of leaves) and suberification (cork) of epidermis cells. These substances which are Figure 4.15: EM image of basal infold of an epithelial cell of the proximal tubule cells of the nephron in the kidney hydrophobic (complex lipid mixture of (bottom). Light microscopy image of the epithelium of cerides) may be added as layers or the epididymis showing stereocilia (top). Lebanese University-FS1. B1100 - Cell Biology by Pr Ziad ABDEL-RAZZAK (2022) 67 they impregnate the cell wall so that it becomes watertight. The main role for cutinization is protective. Gelification is another kind of specialization that results from solubilization of the middle lamella so that the cells round up and lose their adhesion. Gelification occurs in most ripe fruits which get soft because the cells are dissociated and float in a gel of pectin. 8. Microvilli Plasma membrane surface is not smooth; it actually shows temporary invaginations and protrusion. Furthermore, certain cell types show permanent and stable structures named microvilli. They are cytoplasmic finger-like extensions of about 1 to 2µm of length, 0.1 µm of diameter, generally found at the apical surface of epithelial cells. Microvilli are involved in absorption (e.g. enterocytes) or secretion (Figure 4.14). They are so abundant that they appear as a brush at the apical pole of enterocytes. In other cell types such as leukocytes, microvilli occur at low abundance (distant from one another). Therefore, they increase the surface area of the apical plasma membrane thereby making absorption more efficient. Each microvillus is internally supported by a bundle of about 40 actin filaments which are extended in the cytosol connected to other networks of cytoskeleton filaments the base and are periodically connected to the plasma membrane covering the microvillus by myosin-I (Figure 12.7). Adjacent actin filaments are transversely linked together by other proteins (e.g. fimbrin, villin). In addition to their supportive role, actin filaments are responsible for microvilli motility, which causes a flow of material in front of the cell. Microvilli are usually covered by a viscous coat of carbohydrates and glycoproteins, named "glycocalyx", which facilitates their movement. 9. Stereocilia Located at the apical pole of some cells, stereocilia are long extensions of the cytoplasm, that contain actin filaments. They are irregular in size and shape and are flexuous (not straight) and sometimes confluent with each other. They are not capable of active movement. The stereocilia would be involved in phenomena of secretion and reabsorption at the level of certain sensory epithelial cells. They are also seen in the male reproductive system (epididymis epithelium Figure 4.15). 10. Basal infolds A medium, in which cells are placed, is said to be Basal infolds are deep intracellular isotonic (isosmotic) to the cytosol if it contains the invaginations at the basal pole. They are same concentration of solutes. If the surrounding medium is diluted with water, its solutes several µm and abundant in some concentration will decrease and, thus, becomes epithelial cells specialized in hypotonic. When solutes concentration is higher in hydromineral exchanges (proximal tubule the surrounding medium, this latter is called hypertonic. Actually, tonicity is an important of the nephron in the kidney). This physiological parameter and cells should be placed system of folds delimits privileged in an isotonic medium in order to function properly. cytoplasmic compartments for such Blood for instance is isotonic with cells. Therefore, if isotonicity is disturbed, cells will seek to exchanges and rich in elongated reestablish it by allowing passive transport mitochondria providing the energy (diffusion). necessary for the exchanges (Figure Box 4.1: Definition of tonicity. 4.15). IV. SELECTIVE PERMEABILITY OF CELL MEMBRANES# 1. Transport of smal I entities Plasma membrane must prevent leaking out of cellular organic and inorganic components. In addition, it must allow communication with the surrounding medium and the neighboring cells. Plasma membrane and nearly all cell membranes have selective Lebanese University-FS1. B1100 - Cell Biology by Pr Ziad ABDEL-RAZZAK (2022) 68 and controlled permeability. This characteristic mediates many physiological processes that are performed by membrane such as generation and propagation of nerve impulses and glucose intake by the cell. Permeability of membranes to molecules is influenced by several parameters such as the electrical charge of the transported molecule, its molecular weight or size, and the distribution coefficient or partition coefficient. The distribution coefficient relates the solubility of a molecule in oil to its solubility in water. Nonpolar compounds (e.g. benzene, glycerol, steroids) can easily pass across membranes since they can cross the hydrophobic region of the lipid bilayer. Very small inorganic molecules such as CO2, 02 and H20 can easily move between membrane phospholipids even though they are polar (Figure 4.16). By contrast, larger polar (e.g. monosaccharides, "aa") and charged entities (e.g. Na+) show poor membrane penetrability. So, they cannot pass across the membrane unless channels, pumps or transporters 17 are present in mall uncharged molecule large polar molecule and ion the lipid bilayer (Figure 4.16). When channels and transporters are involved, Pore the transportation process could be passive or active. Passive transport or diffusion occurs easily due to Figure 4.16: Selective permeability of lipid bilayers (left). Membrane proteins form channels and transporters that control membrane permeability (right). the concentration gradient (from the side where solute concentration is high to that of low concentration) through channels, transporters or among lipids of the membrane. During passive transport, solute molecules move across membrane due to the energy of the gradient and the membrane is passive, not making any chemical nor metabolic activity and thus it is not spending energy. So, passive transport never leads to accumulation of solutes 18. Instead, it establishes equilibrium between the two compartments (two sides of the membrane) so that solute concentration at both membrane sides are equal and the media become isotonic. After the equilibrium is reached, movement of solute through the membrane continues at equal rates in both directions so that the net flux is null. Active transport requires pumps that are polytopic integral proteins in the membrane. These proteins consume ATP to transport a solute against its gradient; that is, from a medium where solute concentration is low to the other one of higher concentration. For example, the proton pumps in the lysosome membrane transports protons from the cytosol into its lumen to make it acidic, and Na+/K+ pump in the plasma membrane of nearly all cells creates its electrical polarity (the resting membrane potential) by transporting sodium and potassium ions against their respective gradients. 17 Channels differ from transporters and pumps by the mode of transportation. A channel opens by conformational change and allows continuous flow of solute or ions. In contrast, pumps and transporter must undergo conformational change at each cycle of transportation and are usually more specific. 18 Unless molecules pass to the nonsoluble state since unsoluble molecules do not contribute to the gradient. Lebanese University-FS1. B1100 - Cell Biology by Pr Ziad ABDEL-RAZZAK (2022) 69 Water diffuses across the plasma membrane from a hypotonic (Box 4.1) medium to a hypertonic one. Such HYPOTONIC: Normal turgor diffusion causes turgor pressure in plant cells (they pressure become turgid 19) when the medium surrounding the cells is hypotonic (Figure 4.17). It also causes swelling of the red blood cells when they are incubated in a hypotonic medium and their shrinking when they are put in a hypertonic one (Figure 4.18). 2. Transport of macromolecules Carriers and channels located in cell membranes are not the only actors involved in transportation across membranes. Macromolecules and large substrates can V) ·.;; :z:. not pass through membranes in the same mode as ions, V) 0 monosaccharides and "aa". In fact, the transport of large entities (protein, polysaccharide, bacterium, virus,..) across membrane is mediated by vesicles and is known as bulk transport. This mode of transport enables the cell to take in, and to expel very large material such as proteins, complex carbohydrates, complex lipids (e.g. LDL), viral particles, bacteria,... Inward transportation is known as endocytosis and HYPERTONIC: phagocytosis and it is restricted to animal cells since No turgor plant cells have a rigid cell wall and turgor pressure that pressure prevent such processes. However, exocytosis is common to all eukaryotic cells. Figure 4.17: Turgid and plasmolyzed states in a plant cell (see text). Depending on the direction of transport and the captured material, bulk transport is divided into three categories: endocytosis, phagocytosis20 and exocytosis (Figure 4.19). Phagocytosis is the internalization of very large particles and complexes (usually about 1 µm or more, e.g. immune complexes, bacteria) while endocytosis refers to internalization of molecules such as proteins, complex lipids and polysaccharides. Some viruses enter their host by endocytosis. All these modes of crenated normal swollen lysed transportation, especially phagocytosis, involve RED sLooo CELL large movements of the plasma membrane (pseudopod extension) -on concentration which requires ,n extracellular space HYPERTONIC ISOTONIC HYPOTQNIC VERY _ intervention of the \ HYPOTONIC cytoskeleton. In effect, Figure 4.18: Response of erythrocytes to change of medium tonicity. endocytosis continuously removes small portions of the plasma membrane, whereas exocytosis continuously adds fragments to the plasma membrane (Figure 4.19). The vesicles that detach or fuse with the plasma membrane belong to a complex system known as the endomembrane system (details in Ch 6. I. ). From a quantitative standpoint, the resulting membrane turnover is quite impressive. In cultured fibroblasts for instance, a 19 In turgid cells, the vacuole occupies a very large proportion of the cell volume and the thin cytoplasm is pushed to the periphery. 20 Phagocytosis has been once considered as a subclass of endocytosis. Lebanese University-FS1. B1100 - Cell Biology by Pr Ziad ABDEL-RAZZAK (2022) 70 membrane area equal to the total plasma membrane surface area is turned over every few hours due to addition and removal of vesicles. * Phagocytosis Endocytosis Exocytosis * (different types) ♦ Large particle OU1slde of cell (or lumen of organelle] Extracellul r * Plasma cytoplasm t t Outside of cell (or lumen of organelle) Phagosome Figure 4.1: General presentation of phagocytosis, endocytosis and exocytosis and membrane movement and modification during these processes. Lebanese University-FS1. B1100 - Cell Biology by Pr Ziad ABDEL-RAZZAK (2022)