AVIA-1052 Week 1 Day 3 PDF
Document Details
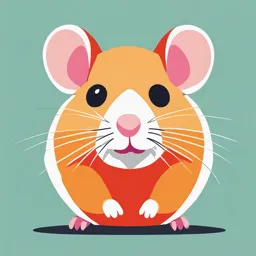
Uploaded by SnazzyDystopia5099
Fanshawe College
Tags
Summary
This document from Fanshawe College covers various aspects of aircraft propeller systems, including their types, location, and maintenance. It includes detailed explanations and diagrams.
Full Transcript
AVIA-1052 1 Previously On AVIA-1052 Well, we talked about… Propeller Location Types of Propellers Fixed-Pitch Propeller Test Club Propeller Ground-Adjustable Propeller Controllable-Pitch Propeller Constant-Speed Propellers Feathering Propel...
AVIA-1052 1 Previously On AVIA-1052 Well, we talked about… Propeller Location Types of Propellers Fixed-Pitch Propeller Test Club Propeller Ground-Adjustable Propeller Controllable-Pitch Propeller Constant-Speed Propellers Feathering Propellers Reverse-Pitch Propellers Propeller Governor Propellers Used on General Aviation Aircraft 2 Today On AVIA-1052 We are going to talk about… Propellers Used on General Aviation Aircraft The never-ending story Metal Fixed-Pitch Propellers Hartzell Constant-Speed, Non-feathering Constant-Speed Feathering Propeller Unfeathering Ice Control Systems Anti-Ice Deice Propeller Synchronization and Synchrophasing Autofeathering System 3 Where are we? Powerplant 7-12 to 7-20 Start at Metal Fixed-Pitch Propellers Stop at Propeller Inspection and Maintenance FAA-H-8083-30A, Aviation Maintenance Technician Handbook-General FAA-H-8083-31A, Aviation Maintenance Technician Handbook-Airframe Volume 1 FAA-H-8083-31A, Aviation Maintenance Technician Handbook-Airframe Volume 2 FAA-H-8083-32A, Aviation Maintenance Technician Handbook-Powerplant Volume 1 FAA-H-8083-32A, Aviation Maintenance Technician Handbook-Powerplant Volume 2 4 AVIA-1052 5 AVIA-1052 6 Powerplant 7-14 Metal Fixed-Pitch Propellers similar in general appearance to a wooden propeller usually, thinner sections widely used on many models of light aircraft LSA (Light Sport Aircraft) many of the earliest metal propellers were manufactured in one piece of forged Duralumin 7 Powerplant 7-14 Metal Fixed-Pitch Propellers compared to wooden propellers lighter elimination of blade-clamping devices lower maintenance cost made in one piece more efficient cooling effective pitch nearer the hub the propeller pitch could be changed within limits by twisting the blade slightly by a propeller repair station because there was no joint between the blades and the hub 8 Powerplant 7-14 Metal Fixed-Pitch Propellers now manufactured as one-piece anodized aluminum alloy identified by stamping the propeller hub with the serial number complete model number combination of basic model number and suffix numbers indicate the propeller diameter the propeller pitch FAA type certificate number production certificate number number of times the propeller has been reconditioned 9 Powerplant 7-14 Metal Fixed-Pitch Propellers 10 AVIA-1052 11 Powerplant 7-14 Hartzell Constant-Speed, Non-feathering Hartzell propellers can be divided by Aluminum hub (compact) and steel hub Hartzell compact aluminum propellers represent new concepts in basic design They combine low weight and simplicity in design and rugged construction In order to achieve these ends, the hub is made as compact as possible, utilizing aluminum alloy forgings for most of the parts The hub shell is made in two halves, bolted together along the plane of rotation This hub shell carries the pitch change mechanism and blade roots internally 12 Powerplant 7-14 Hartzell Constant-Speed, Non-feathering The hydraulic cylinder, which provides power for changing the pitch, is mounted at the front of the hub The propeller can be installed only on engines with flanged mounting provisions One model of non-feathering aluminum hub constant-speed propeller utilizes oil pressure from a governor to move the blades into high pitch (reduced rpm) The centrifugal twisting moment of the blades tends to move them into low pitch (high rpm) in the absence of governor oil pressure This is an exception to most of the aluminum hub models and feathering models https://youtu.be/tIAfmY42siI ConstantSpeedPropPart2 (9:42) 13 Powerplant 7-15 Hartzell Constant-Speed, Non-feathering Most of the Hartzell propeller aluminum and steel hub models use centrifugal force acting on blade counterweights to increase blade pitch and governor oil pressure for low pitch Many types of light aircraft use governor-regulated, constant-speed propellers in two-bladed and up to six- bladed versions These propellers may be the non- feathering type, or they may be capable of feathering and reversing 14 Powerplant 7-15 Hartzell Constant-Speed, Non-feathering The steel hub contains a central “spider,” that supports aluminum blades with a tube extending inside the blade roots Blade clamps connect the blade shanks with blade retention bearings 15 Powerplant 7-15 Hartzell Constant-Speed, Non-feathering A hydraulic cylinder is mounted on the rotational axis connected to the blade clamps for pitch actuation The basic hub and blade retention is common to all models described The blades are mounted on the hub spider for angular adjustment The centrifugal force of the blades, amounting to as much as 25 tons, is transmitted to the hub spider through blade clamps and then through ball bearings The propeller thrust and engine torque is transmitted from the blades to the hub spider through a bushing inside the blade shank 16 Powerplant 7-15 Hartzell Constant-Speed, Non-feathering 17 Powerplant 7-15 Hartzell Constant-Speed, Non-feathering In order to control the pitch of the blades, a hydraulic piston-cylinder element is mounted on the front of the hub spider The piston is attached to the blade clamps by means of a sliding rod and fork system for non- feathering models and a link system for the feathering models 18 Powerplant 7-15 Hartzell Constant-Speed, Non-feathering The piston is actuated in the forward direction by means of oil pressure supplied by a governor, which overcomes the opposing force created by the counterweights Hartzell and McCauley propellers for light aircraft are similar in operation The manufacturer’s specifications and instructions must be consulted for information on specific models 19 AVIA-1052 20 Powerplant 7-15 Constant-Speed Feathering Propeller The feathering propeller utilizes a single oil supply from a governing device to hydraulically actuate a change in blade angle [Figure 7-25] This propeller has five blades and is used primarily on Pratt & Whitney turbine engines A two-piece aluminum hub retains each propeller blade on a thrust bearing A cylinder is attached to the hub and contains a feathering spring and piston The hydraulically actuated piston transmits linear motion through a pitch change rod and fork to each blade to result in blade angle change 21 Powerplant 7-16 Constant-Speed Feathering Propeller 22 Powerplant 7-16 Constant-Speed Feathering Propeller 23 Powerplant 7-15 Constant-Speed Feathering Propeller While the propeller is operating, the following forces are constantly present: spring force, counterweight force centrifugal twisting moment of each blade, and blade aerodynamic twisting forces The spring and counterweight forces attempt to rotate the blades to higher blade angle, while the centrifugal twisting moment of each blade is generally toward lower blade angle Blade aerodynamic twisting force is usually very small in relation to the other forces and can attempt to increase or decrease blade angle 24 Powerplant 7-16 Constant-Speed Feathering Propeller the propeller forces move the blades toward higher pitch (low rpm) These forces are opposed by a variable force toward lower pitch (high rpm) The variable force is oil under pressure from a governor with an internal pump that is mounted on and driven by the engine The oil from the governor is supplied to the propeller and hydraulic piston through a hollow engine shaft Increasing the volume of oil within the piston and cylinder decreases the blade angle and increases propeller rpm If governor-supplied oil is lost during operation, the propeller increases pitch and feathers https://youtu.be/DvWKfu4ubik How it's Made, Airplane Propellers (4:58) 25 Powerplant 7-16 Constant-Speed Feathering Propeller Feathering occurs because the internal propeller forces push the oil out of the propeller until the feather stop position is reached Normal in-flight feathering is accomplished when the pilot retards the propeller condition lever past the feather detent This permits control oil to drain from the propeller and return to the engine sump Engine shutdown is normally accomplished during the feathering process Normal in-flight unfeathering is accomplished when the pilot positions the propeller condition lever into the normal flight (governing) range and restarts the engine 26 Powerplant 7-16 Constant-Speed Feathering Propeller As engine speed increases, the governor supplies oil to the propeller and the blade angle decreases Decreasing the volume of oil increases blade angle and decrease propeller rpm By changing blade angle, the governor can vary the load on the engine and maintain constant engine rpm (within limits), independent of where the power lever is set The governor uses engine speed sensing mechanisms that permit it to supply or drain oil as necessary to maintain constant engine speed (rpm) Most of the steel hub Hartzell propellers and many of the aluminum hub are full feathering 27 Powerplant 7-16 Constant-Speed Feathering Propeller These feathering propellers operate similarly to the non- feathering ones except the feathering spring assists the counterweights to increase the pitch Feathering is accomplished by releasing the governor oil pressure, allowing the counterweights and feathering spring to feather the blades. This is done by pulling the condition lever (pitch control) back to the limit of its travel, which opens a port in the governor allowing the oil from the propeller to drain back into the engine Feathering occurs because the summation of internal propeller forces causes the oil to drain out of the propeller until the feather stop position is reached 28 Powerplant 7-16 Constant-Speed Feathering Propeller The time necessary to feather depends upon the size of the oil passage from the propeller to the engine, and the force exerted by the spring and counterweights The larger the passage is through the governor and the heavier the spring, the quicker the feathering action is An elapsed time for feathering of between 3 and 10 seconds is usual with this system Engine shutdown is normally accomplished during the feathering process In order to prevent the feathering spring and counterweights from feathering the propeller when the engine is shut down and the engine stopped, automatically removable high-pitch stops were incorporated in the design 29 Powerplant 7-16 Constant-Speed Feathering Propeller These consist of spring-loaded latches fastened to the stationary hub that engage high-pitch stop plates bolted to the movable blade clamps. When the propeller is in rotation at speeds over 600–800 rpm, centrifugal force acts to disengage the latches from the high pitch stop plates so that the propeller pitch may be increased to the feathering position. At lower rpm, or when the engine is stopped, the latch springs engage the latches with the high pitch stops, preventing the pitch from increasing further due to the action of the feathering spring. 30 Powerplant 7-17 Constant-Speed Feathering Propeller As mentioned earlier, the engine load would be excessive, especially on fixed-turbine turboprop engines. One safety feature inherent in this method of feathering is that the propeller feathers if the governor oil pressure drops to zero for any reason. As the governor obtains its supply of oil from the engine lubricating system, it follows that if the engine runs out of oil or if oil pressure fails due to breakage of a part of the engine, the propeller feathers automatically. This action may save the engine from further damage in case the pilot is not aware of trouble. 31 AVIA-1052 32 Powerplant 7-17 Unfeathering Unfeathering methods: 1. Start the engine the governor can pump oil back into the propeller to reduce pitch most light twins this procedure is considered adequate since feathering of the propeller rarely happens May vibrate as it come out of feather 33 Powerplant 7-17 Unfeathering Unfeathering methods (continued) 2. An accumulator connected to the governor a valve traps air-oil pressure when the propeller is feathered Releases the pressure to the propeller when the rpm control is returned to the normal position unfeathers the propeller in a very short time and the engine starts to windmill 3. An unfeathering pump provides pressure to move the propeller back to low pitch quickly using engine oil 34 Powerplant 7-18 Unfeathering 35 Powerplant 7-17 Unfeathering Normal in-flight unfeathering accomplished when the propeller condition lever is moved into the normal flight (governing) range causing the governor to disconnect the propeller oil supply from drain reconnects it to the governed oil supply line from the governor. At that point The engine is not running so there is no oil to the governor So, no governed oil is available from the governor for controlling the propeller blade angle and rpm 36 Powerplant 7-17 Unfeathering Normal in-flight unfeathering (continued) As the engine is started the governor supplies oil to the propeller the blade angle decreases As soon as the engine is operating the governor starts to unfeather the blades windmilling takes place speeds up the process of unfeathering 37 Powerplant 7-17 Unfeathering When reciprocating unfeathering is used engine takes a little longer to start turning enough to provide oil pressure to the governor then to the propeller. This delay can cause vibration as the propeller unfeathers 38 Powerplant 7-17 Unfeathering Many aircraft use an accumulator to provide stored pressure to unfeather the propeller much quicker Used when restarting the engine is difficult or for training purposes The system consists of an oil accumulator connected to the governor through a valve air or nitrogen pressure on one side of the accumulator pushes a piston to force oil from the accumulator Through the governor to the propeller piston moving the propeller blades from feather to a lower blade angle 39 Powerplant 7-17 Unfeathering Many aircraft use an accumulator (continued) The propeller begins to windmill the engine to started When the unfeathering pump is used an additional pump that provides the oil pressure propeller control is in the correct position full increase rpm pump is turned on oil pressure from the pump Through the governor unfeathers the propeller 40 Powerplant 7-17 Unfeathering On the ground the blades can be unfeathered using blade paddles. 41 AVIA-1052 42 AVIA-1052 43 Powerplant 7-17 Ice Control Systems Ice formation on a propeller blade produces a distorted blade airfoil section causes a loss in propeller efficiency collects asymmetrically produces propeller unbalance destructive vibration increases the weight of the blades https://youtu.be/ez0ta_GRnnE Aircraft Propeller Ice Tests - Conventional electric de-ice system at 2100 RPM (1:11) 44 AVIA-1052 45 Powerplant 7-17 Anti-Icing Systems Fluid Anti-Ice system typically includes: a tank holds a supply of anti-icing fluid a pump forces fluid to each propeller control system permits variation in the pumping rate quantity of fluid delivered to a propeller can be varied depending on the severity of icing 46 Powerplant 7-18 Anti-Icing Systems Fluid Anti-Ice system [Figure 7-27] 47 Powerplant 7-18 Anti-Icing Systems Fluid Anti-Ice system (continued) Fluid types: isopropyl alcohol high availability low cost phosphate compounds reduced flammability more expensive not widely used 48 Powerplant 7-18 Anti-Icing Systems Fluid Anti-Ice system (continued) feed shoes a narrow strip of rubber on the blades extending from the blade shank to a blade station that is approximately 75% of the propeller radius installed on the blade leading edge because airflow can disperse the fluid to where ice doesn’t collect in large quantities 49 Powerplant 7-18 Anti-Icing Systems Fluid Anti-Ice system (continued) feed shoes molded with several parallel open channels where fluid flows from the blade shank toward the blade tip by centrifugal force 50 Powerplant 7-18 Anti-Icing Systems Fluid Anti-Ice system (continued) Slinger ring transfers fluid from a stationary nozzle on the engine nose case into a circular U-shaped channel (slinger ring) mounted on the rear of the propeller assembly the fluid, under pressure of centrifugal force, is transferred through nozzles to each blade shank 51 Powerplant 7-18 Anti-Icing Systems Fluid Anti-Ice system (continued) some disadvantages: adds weight to the aircraft limited fluid available on board isn’t used on modern aircraft electric deicing systems are used 52 AVIA-1052 53 Powerplant 7-18 Deicing Systems Electric propeller-icing control system consists of an electrical energy source a resistance heating element mounted on the propeller spinner and blades internally externally system controls necessary wiring 54 Powerplant 7-19 Deicing Systems [Figure 7-28] 55 Powerplant 7-19 Deicing Systems electric propeller-icing control system a master switch [optional] a toggle switch for each propeller [optional] a selector switch Adjusts for icing conditions light heavy a timer determines the sequence of which blades are being deiced for what length of time the blades are being deiced 56 Powerplant 7-19 Deicing Systems electric propeller-icing control system a cycling unit applies power to each deice boot (boot segment) 1. in a sequence 2. all in order a brush block normally mounted on the engine behind the propeller transfers electricity to the slip ring 57 Powerplant 7-19 Deicing Systems 58 Powerplant 7-19 Deicing Systems Figure 7-29 59 Powerplant 7-19 Deicing Systems electric propeller-icing control system a slip ring rotates with the propeller provides a current path to the blade deice boots a slip ring wire harness is used on some hub installations electrically connects the slip ring terminal strip connection screw 60 Powerplant 7-19 Deicing Systems electric propeller-icing control system deice boot contains either internal heating elements dual elements securely attached to the leading edge of each blade with adhesive a deice wire harness used to electrically connect the deice boot the slip ring assembly 61 Powerplant 7-19 Deicing Systems Figure 7-30 62 Powerplant 7-19 Deicing Systems electric propeller-icing control system electrical power from the aircraft system transferred to the propeller hub through electrical leads terminate in slip rings brushes from the hub to blade elements through flexible connectors 63 Powerplant 7-19 Deicing Systems electric propeller-icing control system icing control accomplished by converting electrical energy to heat energy in the heating element balanced ice removal from all blades must be obtained quickly before excessive vibration occurs obtained by controlling the heating current in the blade elements similar heating effects are obtained in opposite blades 64 Powerplant 7-19 Deicing Systems electric propeller-icing control system designed to intermittently apply power to the heating elements removes ice after formation before excessive accumulation 65 Powerplant 7-20 Deicing Systems electric propeller-icing control system Proper control of heating intervals aids in preventing runback since heat is applied just long enough to melt the ice face in contact with the blade If heat is supplied more than that required for melting just the inner ice face, insufficient to evaporate all the water formed water will run back over the unheated surface and freeze runback of this nature causes ice formation on uncontrolled icing areas of the blade or surface 66 Powerplant 7-20 Deicing Systems electric propeller-icing control system cycling timers are used to energize the heating element circuits for periods of 15 to 30 seconds a complete cycle time of 2 minutes are electric motor driven contactors that control power contactors in separate sections of the circuit 67 Powerplant 7-20 Deicing Systems electric propeller-icing control system controls for propeller electrical deicing systems include on-off switches ammeters or load meters indicate current in the circuits permit monitoring of individual circuit currents reflect operation of the timer protective devices current limiters circuit breakers 68 Powerplant 7-20 Deicing Systems electric propeller-icing control system To prevent element overheating the propeller deicing system is used only when the propellers are rotating for short tests the takeoff check list system inspection 69 Powerplant 7-20 Deicing Systems Sequence of propeller deice on the Navajo PA31 Right hand prop boots inboard Right hand prop boots outboard Left hand prop boots inboard Left hand prop boots outboard System cycles in this order until deice is selected off 70 AVIA-1052 71 Powerplant 7-20 Propeller Synchronization and Synchrophasing propeller synchronization systems Common on most multi-engine aircraft provide a means of controlling and synchronizing engine rpm reduces vibration and eliminates the unpleasant beat produced by unsynchronized propeller operation an electronic system matches the rpm of both engines can also match a blade phase relationship between the left and right propellers to reduce cabin noise 72 Powerplant 7-20 Propeller Synchronization and Synchrophasing Figure 7-31 73 Powerplant 7-20 Propeller Synchronization and Synchrophasing The system is controlled by switch (on/off) located forward of the throttle quadrant supplies DC power to the electronic control box Input signals providing propeller rpm received from magnetic pickup on each propeller computed input signals corrected to a command signal sent to an rpm trimming coil or motor located on the propeller governor of the slow or slave engine To match rpm of the other propeller 74 Powerplant 7-20 Propeller Synchronization and Synchrophasing 75 Powerplant 7-20 Propeller Synchronization and Synchrophasing 76 AVIA-1052 77 Powerplant 7-20 Auto-feathering System An auto-feather system used normally only during Takeoff Approach and landing feathers the propeller automatically if engine power is lost 78 Powerplant 7-20 Auto-feathering System uses a solenoid valve that dumps oil pressure quickly from the propeller cylinder allowing the prop to feather if two torque switches sense low torque from the engine This system has a test-off-arm switch that is used to arm the system 79