Powder Metallurgy PDF
Document Details
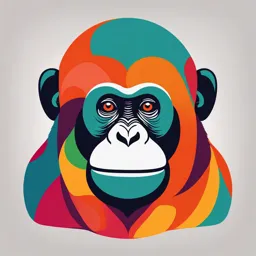
Uploaded by ImaginativePeachTree
STC/JHS
Tags
Summary
This document describes powder metallurgy, focusing on the sintering process and its role in imparting strength to metal components. It also discusses secondary operations such as cold restriking and repressing, which improve density and dimensional tolerances.
Full Transcript
Powder Metallurgy 615 Sintering Purging Preheating Cooling...
Powder Metallurgy 615 Sintering Purging Preheating Cooling Conveyor Fig. 10.6. Sintering Furnace. 1. Loading, purging and preheating portion (drying or burn-off section). 2. Sintering portion (high heat zone). 3. Cooling portion. The strength during sintering is imparted by diffusion bonding of the atoms of the particles. Most metals remain solid during sintering. The solid-state diffusion bonding originates at the old weld zones (created during compacting) and continues until all of the powder particles are welded into a coherent mass. However, sintering process is accelerated (density increase) when one of the constituents melts and envelops the higher melting constituent (Liquid-phase sintering) or Vitreous sintering. A liquid that wets the solid particles exerts capillary pressure which physically moves and presses particles together for better densification. Best bonding is achieved, if there is mutual solubility. In the case of cemented carbides, for example, sintering increases the density of the part due to the action of cobalt (which is added as a binder), which dissolves small fragments of the carbide and promotes fusion and bonding. Particles of iron, copper base or iron base parts do not fuse, however, and are strengthened solely by the action of the atoms as they diffuse and bind to one another.Generally, the sintered part contains from 4 to 10% porosity. The other characteristics accomplished by sintering are : 1. Residual stresses within the particles, caused by the compacting operation, are relaxed, allowing the particles to deform plastically. 2. Voids tend to become closed and this causes some shrinkage during sintering. Therefore, the green compact should be oversized. 3. Individual crystals may be recrystallised within the compact if the sintering temperature is high enough to have an effect on the crystalline structure. 4. The impurities (if any) that are a part of the metal particles are forced out of each individual interstice into small impurity voids within the sintered compact. 10.6.1. Pre - Sintering. If a part made by P/M needs some machining (say drilling), it will be rather very difficult if the material is very hard and strong. These machining operations are made easier by the pre-sintering operation which is done before sintering operation. The compact is heated to a temperature well below the final sintering temperature. The compact will gain enough strength to be handled and machined without any difficulty. After this, the part undergoes the final sintering operation. 10.7. SECONDARY OPERATIONS The relative density of a fully sintered part (that is relative to the fully dense material) is in the range of 65 to 85% depending upon powder characteristics, compacting pressure, and sintering temperature and time. Factors limiting cold densification of P/M compacts include press capacity and compressibility of powder blends. Often a higher porosity is intentionally kept to subsequently 616 A Textbook of Production Technology manufacture porous bearings, filters etc. So, secondary operations are needed to obtain desired dimensional tolerances, physical properties and produce porous bearings etc. Secondary operations may also include: heat treatment and electroplating. 1. Cold Restriking or Repressing. The operations included under this are: sizing and coining. These operations increase the density and improve dimensional tolerances of a part so as to get a more precise size and with a better surface finish (often a burnished surface) than was obtained after sintering. Punch ‘Sizing’ operation consists of holding the part in a simple fixture so that an accurate mandrel or tool can be forced through a hole, slot or some hollow feature of the part. Another method P/M Bushing is to ‘extrude’ or force the part through die openings in a special die, resembling that used originally for compacting the part. The part can also be squeezed between two flat surfaces. Fig. 10.7 shows the sizing operation for the outer and inner surfaces Die of a bush. ‘Coining’ is a variant of closed-die forging in which there is no flash. It consists of repressing P/M parts by use of high pressures in dies especially for this purpose. Increased densities of the order of 95% may be obtained by mechanically reducing the voids that remain between adjoining particles. Densification also improves the surface quality and precision of the part. Further densification and strength improvement can be Die Closed obtained by resintering the repressed compact obtained by sizing Fig. 10.7. Sizing Operation. and coining.“ Coining is not practical on carbide materials due to their low compressibility. 2. Hot Densification. Instead of the traditional pressing, sintering, repressing and resintering sequence, a preform (green compact) that closely resembles the finished part is made by P/M. It is then heated to an elevated temperature and compacted in a finishing die with a single stroke of press. The relative density attained can be as high as 99.5% or even more. The method is also called Powder Forging or P/M forging and has been discussed in chapter 4. Such hot-forged powder preform parts as well as hot-rolled bars possess the wrought properties and complex parts can be made otherwise very difficult to obtain by conventional forging. As discussed, powder metallurgy forged parts have mechanical properties, close to wrought metals, unlike conventional pressed and sintered powder metallurgy parts, that have limited dynamic properties of impact and fatigue. These limitations can be overcome by eliminating residual density with the forging process. In addition to the advantages of the powder forged parts, mentioned on Pages 288, other advantages of the process are given below: 1. High production rates. 2. Reduction or elimination of flash. 3. Lower forging load. 4. Less die wear. 5. Lower forging temperature. 6. Ability to form quite complex components in one forging operation. 7. Lesser forging blows, than in conventional forging. 8. Forging costs are lower than in conventional forging. Powder Metallurgy 617 9. Reduction of lead time and inventory. However, the cost of the powder metallurgy forging process exceeds conventional powder metallurgy products, due to extra processing steps, reduced tool life of forging dies, perform design and development, and the process controls, necessary to achieve full density. Three different approaches have been developed for powder metal forging : (i) Hot repressing. In this method, the perform is very similar in shape to the final part, except its length in the forging direction. That is, the lateral dimensions of the perform are the same as the inner dimensions of the repressing die. Therefore, no lateral flow of material takes place during the repressing stroke. The final product needs minimum machining. This technique is generally used in applications where densities from 95 to 98% of theoretical are satisfactory. Recent modifications to this technique indicate that it may be possible to produce densities approaching 100% theoretical, with properties equivalent to wrought products. (ii) Precision forging process without flash. In this variation, the shape of the perform is simpler than the final part shape. The final shape is produced to close dimensional control during the forging step. In this case, the perform is upset or extruded during the hot forging step, that is, there is lateral flow also of the material. This results in a more rapid densification and greater shear stresses at the pore surfaces, than in hot repressing. This method can produce densities approaching 100% theoretical and develops properties nearly equal to the equivalent wrought metal of the same composition. The method combines the advantages of P/M and conventional forging. The product may need slight machining. (iii) Powder metal forging with formation of flash. In this method, the P/M performs are substituted for bar stock, as the raw material in conventional die forging. That is, the method is an extension of the present forging practice. The product needs trimming and finishing. It appears doubtful, however, whether the method can offer economic advanteges over conventional die forging from bar stock. 3. Infiltration :— In this operation, molten metal is used to fill the porosity in the sintered compact. It is carried out by immersing the part in the molten metal or by placing the infiltrant metal in the form of a sheet or slug either above or below the compact in a furnace. Capillary action fills the pores. Vacuum aids the process. The melting point of liquid metal used should be as low as possible and below that of any of the powders in the compact. Best results are obtained if both wetting and solubility exists between the molten metal and the powders. The operation is principally used for iron-base compacts which increases the density, strength and hardness of a part. Low melting point metals such as copper, copper alloys and brass are used as liquid metal. The operation seals the surface porosity, so that operations like electroplating can be carried out if required. Silver infiltrating tungsten is another typical application. 4. Impregnation :— If the pores in a sintered compact are filled with an oil, the operation is called as impregnation. The lubricants are added to the porous bearings, gears, and pump rotors etc. The parts are immersed in a tank of heated oil for a period of time to fill the pores. Application of vacuum aids the process. Porous components impregnated with lubricants in this manner don't need external lubrication during operation. As the part heats, because of friction during operation, the contained oil will expand and emerge on the bearing surface providing a film of lubricant. Subsequent cooling (after operation) results in re-absorption of oil into the pores of the part. Such bearings are called as “Self Lubricated bearings”. Porosities for such parts range from 25% to 35%. Higher values will reduce the strength of the component. For the production of self lubricated bearings, the iron or bronze compositions are used.Typically, the powders used include: copper, tin, graphite and a small amount of stearing acid. Graphite is added to improve the lubricating qualities of the finished bearing and stearic acid is an effective pore-generating agent. During sintering, the stearic acid, which is volatile, aids in producing interconnecting channels or pores, throughout the material. After the powder composition is 618 A Textbook of Production Technology compacted and sintered, the lubricating oil is impregnated in an impregnation chamber. In this chamber, vacuum is first applied to remove air from the pores. Then, lubricating oil is admitted into the chamber, where it readily penetrates and fills the empty pores due to capillary action. A typical P/M mix can be : Copper = 2%, Graphite = 1%, Zinc stearate = 0.5 to 1.0%. Balance Iron. Impregnation may be done for other reasons also : For example, parts may be impregnated with wax for lubrication or moisture resistance (particularly for electrical parts) ; resin impregnation for strength, bonding or corrosion resistance and plastic impregnation to improve the outer surface for plating. P/M parts can be plated to provide a decorative, corrosion- resistant, or wear-resistant surface. Parts can also be steam treated to increase corrosion-resistance and seal-porosity. Features, that cannot be formed by compaction, can be achieved by machining. During heat treatment, protective atmospheres must be maintained. Lubricant/ Powders Additives Mixer/ Blender Compacting Green Compact Pre- sintering Machining Heat Sintering Treatment Finished Product Heat Sizing/ Finished Treatment Coining Product Finished Coining Sintering Product Sinter Coin- Finished Coining Product ing ing Infiltration Electro- Finished Plating Product Impreg- Electro- Finished nation Plating Product Hot Finished Densification/ Product Forging Fig. 10.8. Flow Chart of P/M Process. Powder Metallurgy 619 Fig. 10.8 shows the flow chart of producing a part by P/M technique, by combining basic and secondary operations. 10.8. RECENT TRENDS IN POWDER METALLURGY 1. Hot Pressing. The chief trend in P/M is to produce full density, high strength parts with fewer processing steps. In the conventional P/M discussed so far, sintering is done after compacting the product. Then secondary operations, such as cold restriking, resintering etc. and hot densification are carried out to increase the density of the product. However, if both compacting and sintering are carried out simultaneously, an improved product is obtained. This is what is done in the process known as ‘Hot Pressing’. Sufficient pressure is applied at the sintering temperature to bring the powder particles together and thus accelerate sintering. Under such conditions porosity can be completely eliminated. However, the chief problem in hot pressing is to find a suitable die material which can withstand the high pressures at the elevated temperatures. Hot pressing in heated graphite or ceramic dies is feasible. However, it is difficult to transmit pressure uniformly to all parts of the compact. This difficulty is overcome in `Hot Isostatic Pressing (HIP)’. In this process the powder is encased in a deformable metal or ceramic can or container that has the shape of the desired part. The can is evacuated and then placed inside a furnace, which in turn is enclosed in a high pressure chamber. The chamber is pressurized with an inert gas (argon) upto 300 MPa or more. Furnace temperatures may range from 480°C to 2000°C. It is clear that the pressure applied is omnidirectional (hydrostatic). Therefore the compact will be uniformly pressed throughout its mass. The powder is compacted, densified and sintered in one step. When the container is removed, a finished product close to the final shape is obtained. This process is particularly suited to producing parts from more exotic metals (high-temperature alloys) that are difficult to forge and machine, for example, Uranium, Zirconium and high strength titanium alloys where high purity is needed. Advantages of HIP 1. The process has the potential for the fabrication of highly refractory ceramics which are difficult to density using more conventional processing routes such as sintering. 2. Low temperatures can be used, thus allowing a better control of microstructure (fine grain size, for instance). 3. Use of proper cans and inserts also allows the fabrication of complex geometries to “near net shape” which are especially interesting in the case of hard to machine materials. Limitations The HIP process is not very attractive for high volume production due to the following factors : 1. High cost of canning. 2. Relatively longer HIP process cycle. 3. Difficulty in producing and maintaining uniform temperature throughout the chamber. Hot rolling, extrusion and forging of metal powders is also feasible. However, provisions must be made to prevent undesirable reactions with the surrounding atmosphere. For this, the metal powder is placed in a thin steel can, that then is welded, evacuated and sealed. After the operation, the can is removed. Or the process can be carried out in a protective atmosphere or carbon is added to produce a reducing environment. 2. Spark Discharge Sintering. This process is a variant of HIP, in which a high energy electric discharge spark is produced during compaction. Due to the high energy of the spark, the diffusion bonding is instantaneous. It thus combines compacting and sintering (as in HIP) the metal powders to a dense metal part in 12 to 15s. Its greatest advantage is its ability to maintain dimensional accuracy of the parts. Also, no separate sintering furnace is needed for this process. 620 A Textbook of Production Technology 3. Gravity Sintering. This method is used to produce thin compacts of low density and little strength. A mould (ceramic trays) may be filled under gravity upto a uniform thickness, perhaps assisted by vibration. The trays are sintered upto about 48 hours in proper furnace environment as discussed earlier. Careful handling is needed to produce a porous sintered product. The process is particularly suited to producing corrosion resistant Stainless-steel porous sheets. These sheets can then be fabricated into suitable shapes and their application is as filters for oil, petrol and chemicals. 4. Induction Sintering. Induction sintering provides extremely rapid heating rates and temperature equilibrium. Also, the process lends itself to short soaking time at temperature. At the higher sintering temperatures short-soaking times at temperatures are sufficient to reduce the oxygen content of the perform to the level achieved during conventional furnace sintering. However at more short times, the diffusion of carbon is incomplete and a post forming heat treatment is necessary to obtain a uniform distribution of carbon. 5. Some newly developed techniques for obtaining full density products are discussed below : (i) The Sinter-HIP Process. This technique is an improvement on the conventional HIP process, in that the expenses on canning and de-canning of the powder are eliminated. The technique consists of the following steps : 1. The components are conventionally compacted. 2. The compacted parts are sintered in a HIP chamber under vacuum. The sintering time should be sufficient, so that all the remaining porosities are closed and isolated. 3. The vacuum is next broken and high pressure is applied to complete the process. The sealed surfaces produced during step. 4. Act as isolating can, during the present step. (ii) Ceracon Process. This technique was developed to achieve full density in the conventionally compacted and sintered parts. However, the process can also be used for porous performs. The process consists of the following steps : 1. The hot perform is completely surrounded by a granular material capable of trans- mitting pressure in the pseudo uniform manner. 2. The complete assembly is then pressed and compacted in a conventional hydraulic press. The technique has the following advantages : (a) No need of canning and de-canning the parts. (b) The compacted part and the pressurizing medium (granular material) can be sepa- rated conveniently. (c) The granular material can be reheated and reused. (iii) Osprey Process. The process is also known as “In situ consolidation”. The process consists of the following steps : 1. The molten metal is atomized into powder. 2. The powder is sprayed by streams of inert gas into a shaped collector mould. 3. Cooling of the droplets is controlled is such a way that they strike the surface of the mould in a semi-solid state and quickly cool. This process can produce 98 to 99% dense parts. The perform, so obtained, is then immediately hot-forged or otherwise hot-worked, to get the final shape. The process has the following advantages : (a) No sintering step is required. (b) The performs have better workability, compared to conventional P/M performs. (c) The collector mould costs are low. Powder Metallurgy 621 (d) The product has uniform fine grain size and uniform chemistry. This process has been used in England and other countries, to produce parts weighing about 0.9 to 1.8 kg from medium-alloy steel, tool steel and super-alloys. 6. Metal Injection Moulding. Metal Injection Moulding (MIM) which is also known as Powder Injection Moulding (PIM) is the newest of the power metallurgy processes. The metal powder is mixed with a suitable plasticizer. This allows the material to be forced into a closed mould, at relatively low pressure and temperature, using a conventional plastic injection moulding machine. The typical temperatures and pressures are : 120 to 250°C and 70 to 140 kPa respectively. After moulding, the organic binder is removed from the part and this is the critical step of the process. After that, the part is sintered in atmospheric or vacuum furnaces. Powders used in this process are much finer than those used for conventional P/M. Due to this, the shrinkage during sintering is very high, reaching as high as 30%. However, the shrinkage is isotropic due to uniform density of the as-moulded part. Common materials used are : pure iron, low alloy steels, stainless steels and certain speciality alloys. The advantages of the process are : 1. Highly complex components, with densities of 96% of the theoretical and above, can be produced. 2. Close tolerance can be maintained, typically 0.003 per mm. 3. Ductility of as-sintered parts is usually high, with common elongation of 25% to 30%. However, the process has the following limitations : (i) Parts are usually limited in overall size and less than about 75 gms. However, larger parts are under development. (ii) Due to the process to remove binder, the cross- sectional thickness of the part is limited, which is usually 6.35 mm or less. 10.9. PROPERTIES OF POWDER METALLURGY PARTS The mechanical properties of powder metallurgy products depend on many variables, for example, shape and size of powder particles used, type of metals used, pressing pressure, sintering temperature and time and finishing treatments and so on. Due to this, it is rather difficult to give generalised information regarding them. However, in general, the sintered products have relatively low mechanical properties compared to similar parts produced by other processes. Mechanical properties are closely related to relative density because pores in the sintered compact act as internal notches resulting in stress concentration. The ductility is markedly less because of lower density. In general, strength properties of parts made from pure metals (unalloyed) are better than the sintered parts made from powders containing alloying elements. Increasing the density of a compact invariably increase the tensile strength, hardness and usually the elongation. Tensile strengths of 275 to 345 MPa are common and strengths above about 690 MPa can be attained. As larger presses and coining and forging combined with P/M preforms are used, to provide greater density, the strength properties of P/M products more nearly equal to those of the wrought products. HIP and spark sintering are also the steps in this direction. 10.10. APPLICATIONS OF P/M PARTS The major product applications of P/M have already been listed while discussing the advantages of the process. Almost all metals lend themselves to P/M. However, largest quantities of products are made from iron powders often alloyed with 4% to 6% copper and 1% graphite for greater strength, of porous iron infiltrated with copper and increasingly of atomized steel. Smaller but increasing quantities are being made of copper and especially aluminium alloys which are preferred in business machines because of their light weight. 622 A Textbook of Production Technology P/M processing has found greatest acceptance for small parts ( < 0.5 kg) in automotive and appliance applications where the ability to produce a nearly final shape requiring a minimum of machining, provides a strong economic advantage. Similarly, the near-net-shape or net-shape components for air craft, and rocket motor applications are of great importance. Such components include: superalloy turbine disks, Ti-alloy bulk heads and fuselage components and rocket nozzles made of W and Mo infiltrated with Cu. In super alloys, the P/M approach avoids the problems of alloy segregation, carbide clustering and residual cost structures. Surgical implants is a small but important application of P/M. Filling of teeth with dental amalgams is a long established application. The amalgams represent room-temperature transient liquid-phase sintering in which an Ag-Cu alloy is amalgamated with Hg, the mercury is used up in the reaction. Beryllium like Tungsten can be processed only by the P/M route. It is hot vacuum pressed. Production of incandescent-lamp filaments :— As already discussed, these lamp filaments are made from Tungsten powder which is doped with small quantities of alloying elements (for example 0.5% Ni) to accelerate sintering. The Tungsten powder is first compacted and then sintered into a bar shaped body. The bar is swaged at one end to a small diameter. While hot, at a temperature of about 930°C, the entire bar is pulled through WC dies to a diameter measuring only 0.25 mm. The finished diameter of the filament is obtained by a final drawing of the material through diamond dies. Tungsten wires drawn in this manner have tensile strengths of the order of 5950 MPa. 10.11. DESIGNING THE P/M PARTS FOR PRODUCTION 1. The design of the component must be such that it can be ejected from the die. Parts with holes whose axes are perpendicular to the direction of pressing cannot be made. Similarly, multistepped diameters, reentrant holes, grooves and undercuts should be eliminated. However, no such constraints are there in the case of isostatic pressing. 2. The main quality attribute of a P/M product is uniform density of the material. Non- uniform density causes stress in the material and consequently, warping and cracking of parts. For this :— (a) an almost uniform thickness of walls must exist throughout the length of the component. Also parts with straight walls are preferred. (b) Since pressure is not transmitted uniformly through a deep bed of powder, the ratio of the unpressed length to pressed length should be kept below 2, if possible, and never exceed 3. (c) The part should be relatively short in length in comparison to its diameters. The length of a die-pressed component should not exceed about 2.5 times its diameter. 1 (d) The height of step (Fig. 10.3) should not be more than height of part, in a single 4 punch design. However, much larger steps are allowable in a multiple punch design. 3. Even under pressure, the powder can not fill very thin sections. Therefore, do not design for thin walls, narrow splines and sharp corners. Minimum wall thickness for cylindrical parts is 1 mm and for parts of other types 1.5 mm. Sharp corners on the components and hence on the punch and die reduce the strength of the tooling. The rounding radii for internal and external corners should not be less than 0.3 mm and 2.5 mm respectively. 4. Avoid abrupt changes in section thickness. Provide ample fillets. 5. Narrow deep flutes should be avoided 6. Straight serrations (lengthwise) can be readily moulded, but threads and cross knurling and similar impressions can not be pressed on vertical sides. Powder Metallurgy 623 7. Holes should have a draft of 0.08 mm per cm and minimum hole diameter for lengths upto 12.7 mm is3.2 mm. 8. The meeting plane between moulding punches should be on a flat or cylinderical surface and never on a spherical surface. 9. Draft should be provided on the part walls perpendicular to the mould parting plane for easy ejection of the compact from the die. The draft angle should range from 5° to 10°. However, no draft is needed for ejection from a lubricated die. 10.12. COMPARISON OF POWDER METALLURGY WITH OTHER PROCESSES Powder metallurgy process can be compared with other processes on the basis of : Tooling cost, production cost, dimensional accuracy and surface quality needed, quantity of production and other features, as discussed below : 1. Die-Casting. Both P/M and die-casting are very competitive as far as dimensional accuracy and surface finish of the components are concerned. However, tooling costs and machine costs usually favour P/M. But, die-casting can be made in sizes beyond P/M capabilities. Again, die- casting is mainly used for non-ferrous metals. Hence, P/M is the choice, when requirements for strength, wear resistance or high operating temperatures exceed the capabilities of die-casting alloys, or if the corrosion resistance of copper alloys or stainless steel is required. 2. Stamping. When a component can be made as one stamping from one die, stamping is usually more feasible and economical than P/M. However, if components need the use of multiple dies or progressive dies, the tooling costs and machine costs are significantly increased and P/M becomes competitive. 3. Fine Blanking. P/M is highly competitive with fine blanking, which runs at slower cycle than conventional stamping and has higher equipment costs. However, fine blanking has less latitude to produce shapes that can be easily designed with P/M. 4. Foundary Castings. P/M offers greater precision, eliminating most or all of the finish machining operations required for castings. P/M avoids casting defects, such as blow holes, slag and shrinkage etc. But foundary castings can be made in sizes which exceed the capabilities of conventional P/M. Where both the processes are viable, foundary castings usually incur lower tooling and material costs, but higher production costs. P/M is usually the choice when production quantities are high. 5. Investment Casting. Both investment casting and P/M are competitive, as far as precision and component materials are concerned. However, shapes which cannot be made by P/M or which require extensive machining, can often be investment cast. Tooling cost is substantially lower, and production costs are higher. Investment casting cannot compete with P/M for very large production volumes. 6. Automatic Screw Machines. Even though automatic screw machines incur the lowest tooling cost of any production methods, are highly automated, not labour intensive, but the material utilization (from bar stock) is very poor. As the production volumes increase, P/M scores over automatic screw machines. Also, P/M can produce irregular shapes, whereas screw machining operations are more advantageous, when the component shape is symmetric with a central axis. PROBLEMS 1. Define “Powder metallurgy” process. 2. Write in brief the basic steps of P/M process 3. Define the following terms: mixing and blending, compacting or briquetting, sintering, Green compact. 624 A Textbook of Production Technology 4. Name the two major advantages in favour of P/M process. 5. List the limitations of P/M process. 6. Discuss the various methods of powder manufacture. 7. Define the following terms related to metal powders :— Surface area, density, Apparent density, Tap density, compressibility, flow rate, Particle shape, Particle size, Particle size distribution. How these powder characteristics influence the properties of a P/M product? Discuss. 8. What are Pre-alloyed and Pre-coated powders? How they are beneficial ? 9. For what types of products the following are added to the powders and why: Graphite, Slearic acid, Cobalt ? 10. What are the sources of the strength of a green compact ? 11. What are the limitations of a single punch design ? 12. What is a ‘multiple punch design’ and ‘rotary table design’ of a P/M press ? 13. Compare the collapsible and non-collapsible dies. 14. Write shortly on :— (a) Impact compacting (b) Rolling and extrusion methods of powder compacting. (c) Centrifugal compacting. (d) Slip Casting. (e) Cold Isostatic Pressing 15. How the final strength is imparted to the green compact during the sintering operation ? 16. Why special precautions are needed about the furnace atmosphere during sintering ? 17. What type of furnace atmosphere is needed during the sintering operation and how it is achieved ? 18. Name the three zones of a sintering furnace and explain the function of each zone. 19. List the properties accomplished by sintering operation. 20. What is pre-sintering ? 21. What are the functions of the following secondary operations done on a sintered product :— Sizing, Coining, Hot densification, Infiltration, Impregnation. How these operations are carried out. 22. How the self lubricated bearings are made ? 23. How the incandescent lamp filaments are made ? 24. What is the advantage of irregular-shaped particles in powders ? 25. What are the principal advantages of fine powders over coarse powders? 26. What is ‘Hot pressing’ ? 27. What is HIP ? Explain the operation and its advantages. 28. Write on: Spark sintering and Gravity sintering. 29. Write on: Properties of P/M products. 30. List the major product application of P/M process. 31. Discuss the design factors of a P/M part. 32. How would the following P/M parts be made :— Cemented carbides, Porous sheet metal, long uniformly shaped parts and Alnico magnets. Powder Metallurgy 625 33. What is Viterous sintering ? 34. Write briefly on the following processes ? (a) Induction Sintering (b) The Sinter-HIP process (c) Ceracon process (d) Osprey process. 35. Write briefly on Metal injection moulding. What are the advantages and limitations of the process. 36. Compare the Powder metallurgy process with the following manufacturing proceses : (a) Die casting (b) Stamping (c) Fine blanking (d) Foundry castings (e) Investment casting (f) Automatis screw machines 37. What is the difference between mixing and blending of powders ? 38. Explain why metal powders are blended ? 39. Explain the difference between infiltration and impregnation. Give some examples for each. 40. Why are protective atmospheres necessary in sintering ? What would be the effects on the properties of powder metallurgy parts if such atmospheres are not used? 41. What are the thermal difficulties experienced during sintering process for some metals. Name those metals. 42. Write a typical P/M mix for producing self lubricated bearings. 43. What are the limitations of HIP process ? 44. What is green strength ? 45. Under what conditions, powder metallurgy technique is superior to conventional manufacturing processes ? 46. Name the important variables in the sintering operation. 47. What is the particle size normally used in P/M method ? 48. Name the common powders employed in P/M process. 49. Explain the important of powder metallurgy. 50. List the advantages of powder metallurgy 51. List the prrducts of Powder metallurgy. 52. Explain why making alloys by melting is unsintable ? 53. Define green density. 54. What are some of the factors which affect the magnitude of the apparent density of powders of the same composition ? 55. Define Compression ratio. 56. What is the effect of the green density of a compact on the hardness it has after sintering ? 57. What is the relationship between % theoretical density and % porosity ? 58. What is meant by “Green” ? Is green strength important ? Explain. 59. Why MIM of metal powders is becoming an important process ? Explain. 60. Describe what happens during sintering operation. 61. Write a short note on sintering furnaces. 62. Discuss the effects of different shapes and sizes of powder particles in PM processing. 63. List the advantages and limitations of MIM as compared to other methods of compaction. Chapter 11 Processing of Plastics 11.1. GENERAL Plastics belong to the family of organic materials. Organic materials are those materials which are derived directly from carbon. They consist of carbon chemically combined with hydrogen, oxygen and other non- metallic substances, and their structures, in most cases, are fairly complex. The large and diverse organic group includes the natural materials : wood, coal, petroleum, natural rubber, animal fibers and food, which have biological origins. Synthetics include the large group of solvents, adhesives, synthetic fibers, rubbers, plastics, explosives, lubricants, dyes, soaps and cutting oils etc. which have no biological origins. Of them, plastics and synthetic rubbers are termed as “polymers”. 11.2. POLYMERS The term “polymer” is derived from the two Greek words : poly, meaning “many”, and meros meaning “parts” or “units”. Thus polymers are composed of a large number of repeating units (small molecules) called monomers. The monomers are joined together end-to-end in a polymerization reaction. A polymer is, therefore, made up of thousands of monomers joined together to form a large molecule of colloidal dimension, called macromolecule. The unique characteristic of a polymer is that each molecule is either a long chain or a network of repeating units all covalently bonded together. Polymers are molecular materials and are generally noncrystalline solids at ordinary temperature, but pass through a viscous stage in course of their formation when, shaping is readily carried out. The most common polymers are those made from compounds of carbon, but polymers can also be made form inorganic chemicals such as silicates and silicones. The naturally occuring polymers include : protein, cellulose, resins, starch, shellac and lignin. They are commonly found in leather, fur, wool, cotton, silk, rubber, rope, wood and many others. There are also synthetic polymers such as polyethylene, polystyrene, nylon, terylene, dacron etc., termed under plastics, fibers and elastomers. Their properties are superior to those of the naturally occuring counterparts. Our concern, here, is therefore, with synthetic polymers, also called plastics (again from Greek plastikos, derived from plassein : to form, to mould) or resins. 11.3. POLYMERIZATION The process of linking together of monomers, that is, of obtaining macromolecules is called “polymerization”. It can be achieved by one of the two processing techniques : (a) Addition Polymerization. In addition or chain polymerization under suitable conditions of temperature and pressure and in the presence of a catalyst called an initiator, the polymer is produced by adding a second monomer to the first, then a third monomer to this dimer, and a fourth to the trimer, and so on until the long polymer chain is terminated. Polyethylene is produced 626 Processing of Plastics 627 by the addition polymerization of ethylene monomers. This linear polymer can also be converted to a branched polymer by removing a side group and replacing it with a chain. If many such branches are formed, a network structure results. “Co-polymerization” is the addition polymerization of two or more different monomers. Many monomers will not polymerize with themselves, but will copolymerize with other compounds. (b) Condensation Polymerization. In this process, two or more reacting compounds may be involved and there is a repetitive elimination of smaller molecules, to form a by-product. For example, in the case of phenol formaldehyde (bakelite), the compounds are : formaldehyde and phenol. Metacresol acts as a catalyst and the by-product is water. The structure of the `mer' is more complex. Also, there is the growth perpendicular to the direction of chain. This is called ‘cross- linking’. Size of a Polymer. The polymer chemist can control the average length of the molecules by terminating the reaction. Thus, the molecular weight (the average weight, in grams, of 6.02 × 1023 molecules) or degree of polymerization, D.P., (the number of mers in the average molecule) can be controlled. For example, the length of molecules may range from some 700 repeat units in low- density polyethylene to 1,70,000 repeat units in ultrahigh-molecular-weight polyethylene. 11.4. ADDITIONS TO POLYMERS The properties of polymers can be further modified by the addition of agents which are basically of two types. Those that enter the molecular structure are usually called “additives”, whereas those that form a clearly defined second phase are called “fillers”. 1. Plasticizers. Plasticizers are liquids of high boiling point and low molecular weight, which are added to improve the plastic behaviour of the polymer. The broad role of a plasticizer is to separate the macro- molecules, thus decreasing the inter-molecular forces and facilitating relative movement between molecules of the polymer, that is, making deformation easier. They are essentially oily in nature. Organic solvents, resins and even water are used as plasticizers. 2. Fillers. A filler is used to economize on the quantity of polymer required and/or to vary the properties to some extent, for example, mechanical strength, electrical resistance etc. A filler, whose function is to increase mechanical strength, is termed a “reinforcing filler”. A filler is commonly fibrous in nature and is chemically inert with respect to the polymer with which it is to be used. Common fillers are wood flour, cellulose, cotton flock, and paper (for improving mechanical strength); mica and asbestos (for heat resistance); talc (for acid resistance).Other filler materials are : fabric, chipped-wood moulding compound, wood veneer, textile or glass fibres. Wood flour is a general purpose filler. It improves mouldability, lowers the cost with fairly improved strength of the plastics. Mica also imparts excellent electrical properties to plastics and results in low moisture absorption. The commonly used “reinforcing filler agents” with plastics are : fibres/filaments of glass, aramid, graphite or boron. Reinforcing by metal and glass fibres make plastics strong, flexible and light materials such as used in bullet proof vests. Cotton fibres improve toughness. Carbon fibres are used for high performance installations such as aircrafts etc. requiring high strength and stiffness. 3. Catalysts. These are usually added to promote faster and more complete polymerization and as such they are also called ‘accelerators’ and ‘hardeners’ e.g., ester is used as a catalyst for Urea Formaldehyde. 4. Initiators. As the name indicates, the initiators are used to initiate the reaction, that is, to allow polymerization to begin. They stabilize the ends of the reaction sites of the molecular chains. H2O2 is a common initiator. 5. Dyes and Pigments. These are added, in many cases, to impart a desired colour to the material. 628 A Textbook of Production Technology For example, titanium dioxide is an excellent white pigment ; iron oxides give yellow, brown or red colour ; carbon black is not only a pigment but also a UV light absorbent. Finely divided calcium carbonate dilutes (extends) the colour and is used in large quantities as low-cost filler. 6. Lubricants. Lubricants are added to the polymers for the following purposes : to reduce friction during processing, to prevent parts from sticking to mould walls, to prevent polymer films from sticking to each other and to impart an elegant finish to the final product. Commonly used lubricants include : oils, soaps and waxes. 7. Flame retardants. Most plastics will ignite at sufficiently high temperatures. The non- inflammability of the plastics can be enhanced either by producing them from less inflammable raw materials or by adding “flame retardants”. The common flame retardants are : compounds of chlorine, bromine and phosphorous. 8. Solvents. Solvents are useful for dissolving certain fillers or plasticizers and help in manufacturing by allowing processing in the fluid state. For example, alcohol is added in cellulose nitrate plastics to dissolve Camphor. However, subsequently, the solvents must be removed by evaporation. 9. Stabilisers and anti-oxidants are added to retard the degradation of polymers due to heat, light and oxidation. 10. Elastomers are added to plastics to enhance their elastic properties. Note. Above, excepting fillers, all other materials used, fall under the category of “Additives”. 11.5. PLASTICS Polymers can be divided into three broad divisions : plastics, fibers and elastomers (polymers of high elasticity, for example, rubber). Synthetic resins are usually referred to as plastics. Plastics derive their name from the fact that in a certain phase of their manufacture, they are present in a plastic stage (that is, acquire plasticity), which makes it possible to impart any desired shape to the product. Plastics fall into a category known chemically as high polymers. Thus, “Plastics” is a term applied to compositions consisting of a mixture of high-molecular compounds (synthetic polymers) and fillers, plasticizers, stains and pigments, lubricating and other substances. Some of the plastics can contain nothing but resin (for instance, polyethylene, polystyrene). 11.5.1.Types of Plastics. Plastics are classified on the broad basis of whether heat causes them to set (thermosetting) or causes them to soften and melt (thermoplastic). 1. Thermosetting Plastics. These plastics undergo a number of chemical changes on heating and cure to infusible and practically insoluble articles. The chemical change is not reversible Thermosetting plastics do not soften on reheating and can not be reworked. They rather become harder due to completion of any left-over polymerization reaction. Eventually, at high temperatures, the useful properties of the plastics get destroyed. This is called degradation. The commonest thermosetting plastics are : alkyds, epoxides, melamines, polyesters, phenolics and ureas. 2. Thermoplastic Plastics. These plastics soften under heat, harden on cooling, and can be resoftened under heat. Thus, they retain their fusibility, solubility and capability of being repeatedly shaped. The mechanical properties of these plastics are rather sensitive to temperature and to sunlight and exposure to temperature may cause thermal degradation. Common thermoplastic plastics are : acrylics, poly tetra fluoro ethylene (PTFE), polyvinyl chlorides (PVC), nylons, polyethylene, polypropylene, polystryrene, etc. Thermosetting plastics are cross-linked polymers and the thermo- plastics are linear and branched- linear polymers. The method of process- ing a plastic is determined largely by whether a plastic is thermosetting or thermoplastic. Processing of Plastics 629 Thermosetting plastics are usually harder, stronger, and more brittle than thermoplastics. Since they do not soften when heated and roasted or charged at high temperatures, thermosetting plastics have applications only in moderate service temperatures, for example, saucepan handles. Thermoplastics are reclaimable while thermosetting plastics are not. They have less load carrying capacity than thermosetting plastics. Moreover, reinforcement fibres can be used to increase the load carrying capacity of the later. 11.5.2. Properties of Plastics. Their great variety of physico- chemical and mechanical properties, and the ease with which they can be made into various articles have found plastics their wide application in the engineering and other industries. 1. Their comparatively low density (1 to 2 g/cm3 ), substantial mechanical strength, higher strength – to – weight ratio and high anti friction properties have enabled plastics to be efficiently used as substitute for metals, for example, non-ferrous metals and alloys-bronze, lead, tin, babbit etc., for making bearings. 2. With certain special properties (silent operation, corrosion resistance etc.), plastics can sometimes replace ferrous metals. 3. From the production point of view, their main advantage is their relatively low melting points and their ability to flow into a mould. 4. Simple processing to obtain machine parts. Generally there is only one production operation required to convert the chemically manufactured plastic into a finished article. 5. In mass production, plastics substituted for ferrous metals allow the production costs to be reduced by a factor of 1.5 to 3.5 and for non-ferrous metals by a factor of 5 to 20. 6. Good damping capacity and good surface finish of the product. 7. The high heat and electric insulation of plastics permits them to be applied in the radio and electrical engineering industries as dielectrics and as substitutes for porcelain, ebonite, shellac, mica, natural rubber, etc. 8. Their good chemical stability, when subjected to the action of solvents and certain oxidizing agents, water resistance, gas - and steam- proof properties, enable plastics to be used as valuable engineering materials in the automobile and tractor, ship building and other industries. With the development of high - performance engineering plastics, they are successfully competing with other engineering materials. They are replacing sheet metal parts, zinc and Al alloy castings and C.I. They are being increasingly used in automobile industry, where weight reduction is one of the means of fuel economy. The initial use of plastics in this direction was for fascia panels, interior fittings, and other non-load bearing components. Now structural parts such as bumpers, brake fluid tanks and some body parts are also being made of plastics. Products from thermoplastic plastics cannot be used at high temperature. Typical examples are : refill and body of Pen, carry bags etc. Switch boards and Chairs are made from thermosetting plastics. Smaller sizes of dust bins and water tanks are made from thermoplastic plastics, while the bigger sizes from thermosetting plastics. Disadvantages 1. Comparatively higher costs of materials. 2. Inability of most plastics to withstand even moderately high temperatures. The properties and uses of common thermoplastics and thermosetting plastics have been given in Chapter 2 (Tables 2.16 and 2.17). The factors which have determined the rapid growth of polymer materials in the recent past, are : 1. Ready availability of the basic raw chemical materials in large quantities and, in general, at a low cost. 630 A Textbook of Production Technology 2. The large number of available starting materials for their production provide us with an almost continuous spectrum of composition and structure, and hence of mechanical, optical, electrical and thermal properties of the resulting polymers. 3. The engineer now has at his disposal many well developed processes and machines to convert these materials (as they come from the factory and that he can choose according to the specification of the ultimate product) into useful goods. 11.6. PROCESSING OF THERMOPLASTIC PLASTICS The common forms of raw materials for processing plastics into products are :– pellets, granules, powders, sheet, plate, rod and tubing. Liquid plastics are used especially in the fabrication of reinforced – plastic parts. Plastic Material Parting Line Heating Section Die Ram Mould-Water Cooled Mould Cavity Fig. 11.1. Injection Moulding Process. Thermoplastics can be processed to their final shape by moulding and extrusion processes. However, extruding is often used as an intermediate process to be followed by other processes, for example, vacuum forming or machining. 11.6.1. Injection Moulding. An important industrial method of producing articles of thermoplastics is injection moulding, (Fig. 11.1). The process is essentially as follows : The moulding material is loaded into a hopper from which it is transferred to a heating section by a feeding device, where the temperature is raised to 150°C –370°C and pressure is built up. The material melts and is forced by an injection ram at high pressure through a nozzle and sprue into a closed mould which forms the part. The mould is in at least two sections, so that it may be split in order to eject the finished component. For the process to be competitive, the mould must be fairly cool (between ambient temperature and the softening point of the plastic) and consequently the mould must be cooled by circulating water. The improvement to the ram type injection moulding machine lies in the separation of the plasticizing and filling actions. The single-screw pre-plasticizer is probably the most successful design for injection moulding machines, (Fig. 11.2). The rotation of the screw provides the plasticizing action by shearing and frictional effects and the axial motion of the screw provides the filling action. Injection moulding machines have a high production capacity : some can produce from 12 to 16 thousand parts per shift. This method is suitable for making parts with complex threads and intricate shapes, thin-walled parts etc. Typical parts include : Cups, containers, housings, tool handles, toys, knobs, plumbing fittings, electrical and communication components such as telephone receivers etc. Processing of Plastics 631 The limitations of the process are :- Equipment of cylinder and die should be non-corrosive. Also, reliable temperature controls are essential. Plastic Material Screw Mould Fig. 11.2. Screw Injection Moulding Machine. Injection moulding machines range in size from an injection capacity of 12,000 mm3 to 2.2 × 10 mm3. The locking forces are applied to the mould usually by hydraulic means, and may vary 6 from 0.1 MN to 8.0 MN or even more. The injection pressure may range from 100 MPa to 150 MPa. 11.6.2. Extrusion Process. The extrusion process, in many cases, produces material in an intermediate form for subsequent reprocessing to its final component form. The process is the same as for metals, that is, the expulsion of material through a die of the required cross-section. The earliest extrusion machines were of the ram type. The cylinder of the machine (container) is filled with prepared plastic and extruded through a die under the pressure of the ram. The advantages of this machine are : simplicity in operation and a controlled pressure which can be virtually as high as required. If the polymer can be plasticized by pressure, then the ram extruder is advantageous in view of its simplicity. But for plastics which require heat, the separate pre-processing may be regarded as a draw back. Another major drawback of this type of machine is the reciprocating action of the ram which is time wasting since the ram must be withdrawn after its power stroke and a new dolly of material inserted in the container. Also, with many materials, the die orifice must be cleaned between each working stroke. Nowadays, the ram machine is mainly used for “wet extrusion” that is for extruding plastics which have been softened by the addition of solvents. Although useful in homogenizing materials which contain hard inclusions, wet extrusion has the disadvantage of producing a component from which the solvent has to be removed. For the extrusion of plastics, single-screw machine has completely replaced the ram type machine. There are two basic types of screw extruders : the melt extruder and the plasticizing extruder. In the former, the material is delivered to the extruder already melted and thus the function of the extruder is merely to push the material to the die and through the orifice. In the plasticizing extruder the material is in the form of granules or particles and so the extrude has to compress and work it until it melts before delivering it, under pressure, to the die orifice. Fig. 11.3. illustrates a screw type extrusion machine. It consists of : a water cooled screw having a special thread form to suit the material being extruded ; a barrel in which the screw rotates (including a form of heating in the case of a plasticizing extruder) ; and an extrusion die. The material is fed from a hopper through a port in the cylinder where the rotation of the screw imparts both axial and rotary motion to the particles. The restricting effect of the die at the far 632 A Textbook of Production Technology end builds up a pressure in the particulate mass which is then worked by shearing and heated by frictional effects until it is in a plastic state and can be extruded. Plastic Feed Chamber Die Screw Fig. 11.3. Screw - Extrusion Machine. Complex shapes with constant cross-sections can be extruded with relatively inexpensive tooling. The extruded product can be coiled or cut into desired lengths. 11.6.3. Sheet-forming Process. Many plastic articles are formed from sheet. The processes resemble those for metals, but require very low forces. Even atmospheric pressure may be sufficient. In “Drape forming”, the sheet is heated to a moderate temperature. It is then clamped at the edges and stretch-formed over a die (See Fig. 4.99). One of the problems encountered is that the portions of the sheet first touching the die will be chilled and remain thicker than the rest. This is overcome or minimised by blowing hot air between the sheet and the die. Vacuum forming is a process, in which a heated plastic sheet is changed to a desired shape by causing it to flow against the mould surface by reducing the air pressure between one side of the sheet and the mould surface. The process consists of clamping the heated plastic sheet over a mould in such a way that the air between the sheet and mould can be evacuated, (Fig. 11.4). This vacuum, of increasing intensity, draws the sheet against the surface of the mould, where it cools and Heated Plastic solidifies. The solidification will take Normal Air Pressure Sheet Clamp place earliest in those regions which Clamp touch the mould first, (Fig. 11.5 a). This will cause differential cooling Vaccum and, as a result of non uniform temperature distribution, there will be a marked change in thickness Fig. 11.4. Principle of Vacuum Forming. along any given section of the component. Thinning is clearly worst where the sheet contacts the mould first (high spots on the mould) and near the peripheral clamping ring. This effect is reduced in drapeforming by replacing the clamping ring by a movable ring or drape, so that part of the profile is achieved before evacuation occurs, (Fig. 11.5 b). There is still a definite depth limitation, however, and additional steps must be taken to eliminate thinning in very deep components. Where one of the difficulties is the restriction of movement at a right-angle corner, (Fig. 11.5 c), the same circuit which is used to extract air can be used earlier in the cycle, to blow hot air between the sheet and the mould. The air pressure acts as a cushion and the air temperature delays cooling of the sheet. Processing of Plastics 633 (a) (b) (c) air Fig. 11.5. Vacuum Forming Processes. This method is also known as “Thermo-forming”. Since high strength of the moulds is not a prerequisite, these are usually made of aluminium. So, tooling cost is quite less. Product applications are : Refrigerator liners, Packaging, Appliance housings, Panels for shower stalls and advertising signs. 11.7. PROCESSING OF THERMOSETTING PLASTICS Compression moulding and Transfer moulding are the most common methods of processing thermosetting plastics. Although, suitable for thermoplastics also, the main application of these methods is to thermosets. 11.7.1. Compression Moulding. Punch (Male Die) Compression moulding, (Fig. 11.6), is the Plastic equivalent of closed-die forging. In this Material process, a premeasured quantity of plastic in the form of particles or briquettes, is Part placed in a heated mould and compressed Female at suitable pressure and temperature. Die I II III Hydraulic presses are usually employed Ejector to provide the pressure (which may range from 20 to 30 MPa or even higher upto 80 MPa in some cases) for compressing Fig. 11.6. Compression Moulding. the plastic compound. Other equipment, such as friction and screw presses, can also be used. The object of compression moulding is to bring the plastic to virtually molten state. Thus the process is, effectively, forming from the liquid state, the material being held in the mould until the curing stage is over when polymerization is complete. The process is rather slow with the phenolics and urea resins, but some of the newer resins have shorter curing times and this has improved the production rates appreciably. When the plastic is completely trapped between the male and female die, it is called as “positive mould”. Closer tolerances can be held if a small flash is allowed to extrude, usually along the male die perimeter in “Semipositive moulds’’. More plastic is lost in “flash mouldsª, similar to those used in impression-die forging. Typical product applications are : Disches, handles, container caps, fittings, electrical and electronic components, washing machine agitators and housings etc. 11.7.2. Transfer Moulding. Transfer moulding, (Fig. 11.7), is a modification of compression moulding in which the material is first placed in a separate chamber (transfer pot), from which it is pushed through an orifice (sprue) into the mould cavity (by the action of a punch) as the mould 634 A Textbook of Production Technology closes. The material to be moulded is often pre-heated by radio-frequency methods and, where it is desired to improve toughness and strength, reinforcing fillers may be used. The process has got the following advantages : 1. There is little pressure inside the mould cavity until it is completely filled, at Punch which stage the full liquid pressure is transmitted. 2. The plastic acquires uniform temperature Loading Chamber and properties in the transfer pot prior Space (Transfer Pot) to transfer. The plastic is further heated Channels Upper Plate by shearing through the orifice, viscosity is reduced, and the plastic fills the Part Die intricate mould cavities. 3. It scores over normal compression Ejector moulding in that cold presses can be used, since, heating of the plastic is affected, not by press itself, but by a Fig. 11.7. Transfer Moulding. simple heating jacket round the transfer chamber. Field of Application :- It is used less for mass production. Its chief application is in short runs where the shape of the moulding would make readjustment of the injection moulding machine profitable. 11.8. CASTING OF PLASTICS Casting of plastics in moulds finds application when making parts of a plastic material with a binder but no filler. It is also used to obtain various kinds of cast thermosetting plastics, for example, cast car- bolite, as well as certain cast thermoplastic materials, such as organic glass, polystyrene and others. The method is simple and cheap since no expensive tooling or equipment is required, and no pressure needs to be applied to fabricate the part. There are many variations of the casting method for plastics : 1. By using flexible moulds, very intricate shapes can be fabricated. The mould is peeled off afterwards. 2. Thick plastic sheets are produced by using plate glass moulds. 3. Thinner plastic sheets are produced by using moving stainless steel belts which contain and cool the resin. 4. Hollow shapes can be obtained by centrifugal casting of the molten plastic material. 5. Potting. In this method, the plastic material is cast around an electrical component which gets embedded in the plastic material. This is achieved by pouring the molten plastic material in a housing or case which is an integral part of the component and in which the component is prepositioned before pouring the plastic. 6. Encapsulation. Here, the component is covered with a layer of cooled and solidified plastic. Both potting and encapsulation are very important to the electrical and electronics industry. The plastic material serves as a dielectric. 7. Foam moulding/casting. In this method a foaming agent is mixed with the plastic resin. The mixture is placed in a mould and heated. The foaming agent makes the material to Processing of Plastics 635 expand (even upto 50 times the original size) to take up the shape of the mould. The amount of expansion can be controlled through temperature and time. Both rigid and flexible foamed plastics can be obtained from thermo-plastics and thermo-setting plastics. Rigid construction is used for structural purposes and flexible for cushioning. Product applications include : Shaped packaging materials for cameras, appliances and electronics etc., insulating blocks, food containers and styrofoam cups. 11.9. MACHINING OF PLASTICS Plastics can be machined, but in most cases, machining of plastics is not required. Acceptable surface quality and dimensional accuracy can be obtained by moulding and forming methods. However, there are certain plastics like PTFE (Polytetra fluoroethylene) which are sintered products and are not mouldable by usual techniques, as they do not melt. For such “thermo stable plastics” machining is a viable alternative to moulding. The machining of plastics (by operations such as turning, drilling and milling) has special features due primarily to the structure of the material. It also depends upon the binder and the filler and the method of moulding the component. For example, the machining of thermosetting plastics allows optimum cutting variables and tool geometry to be employed because these do not soften on heating, whereas thermoplastic resins soften under heat. The permissible maximum temperature in the cutting zone is 160°C for thermo-setting resins and only 60°C to 100°C for thermoplastics. Special features of the machining of plastics are : 1. The tendency of certain plastics to splitting. 2. High elasticity (40 times as much as that of steels). Therefore, they must be carefully supported, to avoid their deflection during machining. 3. Non-homogeneous structure of the material, with components of different hardness. This results in poor surface finish after machining. 4. Plastics have a strong abrading action on cutting tools. 5. Their low thermal conductivity results in poor heat dissipation from the cutting zone and in over-heating of the cutting edges. 6. The intense dust formation, especially for thermosetting plastics, makes it necessary to use special dust-removing devices. 7. The hygroscopicity of plastics excludes the use of liquid cutting fluids. Compressed air is commonly used for cooling. 8. Reinforced plastics are very difficult to machine. Plastics can be machined with H.S.S. and cemented-carbide tools. In machining a plastic material with a filler of glass, quartz or mica type, a satisfactory tool life can be obtained only with carbide-tipped tools. Only diamond tools are suitable for turning high-strength plastics of this type. The strength of cast parts of laminate plastics is 40 to 50 per cent less than that of the parts made by compression moulding. Therefore, higher cutting speeds and feeds can be used in their machining than for strong thermo-setting plastics. The main trouble in turning laminated plastics is the peeling of the surface layer. The cutting variables are also influenced by the life of the cutting tool which is subject to abrasive wear in machining most engineering plastics. Dulling of the cutting tool leads to a poor surface finish and to breaking out of the material at the points the cutting tool enters and leaves the cut. This makes it necessary to use more keenly sharpened cutting tools for plastics. The need for sharp cutting edges also follows from the high elasticity of plastics. The selection of cutting variables is also influenced by the low heat conductivity of the plastics, since, in machining the tool may be within a closed volume (as in drilling) with no cooling facilities. This may lead to charring of the machined surface. 636 A Textbook of Production Technology The cutting tool angles for machining plastics are made somewhat different than those of tools for ferrous and non-ferrous metals. The rake angles are positive and relatively larger. Because of the viscoelastic behaviour of thermoplastics, some of the local elastic deformation is regained when the load is off. Therefore tools must be made with large relief angles (20° to 30°). Abrasive machining of plastics has many advantages over machining with metal cutting tools. These include the absence of splitting and crack formation, and the better surface finish that can be obtained. In grinding, the contact between the wheel and the surface being ground, should be as short as possible, to avoid burns. Organic glass is commonly ground with coated abrasive, applying an ample amount of water as a coolant. If possible, however, grinding should be replaced by polishing with a felt, broadcloth or flannel wheel charged with lapping paste, the process is known as “Buffing”. The buffing wheels are of diameter 250 mm, 40 to 60 mm wide and of speed 2000 rev/min. Meduim and fine lapping pastes are used as the buffing compound for plastics. Laminate fabric base, asbestos- fibre and glass-fibre laminate can be cut with abrasive wheels (SiC) of grain size 24 to 46 and with a 5% emulsion as the cooling fluid. 11.10.OTHER PROCESSING METHODS FOR PLASTICS 1. Calendering. It is an intermediate process where the extruded plastic sections are reduced to sheet which may or may not, then be formed to final shape by vacuum forming. It is clear that the calendering process can be used only for thermoplastics and not for thermosetting plastics. Calendering is in some ways similar to rolling process in that the material is compressed between rolls and emerges as sheet, (Fig. 11.8 a). However, there are differences. There is appreciable thickening after the material has reached minimum thickness at the roll gap and the pre-calendered material is not in sheet form, but of indefinite shape. The method of producing wide sheet and foil is illustrated in Fig. 11.8 b. The thermoplastic melt is fed to a multiroll calendar. The first roll gap serves as a feeder, the second as a metering device, and the third roll gap sets the gauge of the gradually cooling plastic which is then wound, with about 25% stretching onto a drum. Calendering is a high-production rate (typically 100 m/min) process, mostly for flexible PVC, for example, upholstery, rainwear, shower curtains, tapes, etc. and rigid PVC, for example, trays, credit cards, laminations. PVC is also calendered into the well known transparent film widely used for packaging. Roll Plastic Roll (a) (b) Fig. 11.8. Calendering Process. Processing of Plastics 637 2. Rotational Moulding. In this process, also called “roto- moulding”, large relatively thin- walled hollow (open or closed) parts are made. A measured quantity of polymer powder is placed in a thin-walled metal mould. The mould is closed and is rotated about two mutually perpendicular axes as it is heated. This causes the powder to sinter against the mould walls, building up the wall thickness of the component. At the end of the heating and sintering operations, the mould is cooled while it is still rotating. Cooling is done by applying cold water and air to the outside of the rotating mould. The rotation is then stopped and the component is removed. To increase production rates, three moulds at the end of three arms joined together to the central spindle (just like centrifuge casting) are used, with one mould for each stage of the process, that is, load-unload, heat and cool positions. The process is simple as no pressure is employed and the part is free of moulded in stresses. The technique is extensively used for the production of toys in P.V.C. such as boats, horses etc. Large containers of polyethylene (or upto 20,000 litre capacity) and large components like laminated petrol tanks for motor cars are made from polythene (outer shell) and nylon (inner shell). Other products include : Trash cans, boat hulls, buckets, housings, and carrying cases etc. 3. Blow Moulding. In this process, a hot extruded tube of plastic, called a parison, is placed between the two part open mould, (Fig. 11.9 a). The two halves of the mould move towards each other so that the mould closes over the tube. The tube gets pinched off and welded at the bottom by the closing moulds, (Fig. 11.9 b). The tube is then expended by internal pressure, usually by hot air, which forces the tube against the walls of the mould, (Fig. 11.9 c). The component is cooled and the mould opens to release the component, (Fig. 11.9 d). Typical product applications are : Plastic beverage bottles and hollow containers. Extruding Die Parison (a) (b) Air Nozzle Part (c) (d ) Fig. 11.9. Blow Moulding. 4. Reaction Injection Moulding (RIM). This method differs from the conventional injection moulding process in the sense that it is not the molten polymer which is injected into a mould, but a mixture of two or more monomers (reactants) are forced into a mould cavity. Chemical reaction takes place between the constituents of the mixture giving off heat to form a plastic polymer, which 638 A Textbook of Production Technology solidifies producing a thermoset component. The major product applications include : Automotive bumpers, and fenders, thermal insulation for refrigerators and freezers and stiffners for structural components. 5. Solid State Forming. The term is a misnomer because the temperature of the polymer is just (10° to 20°C) below the melting point of the polymer. The main operations involved are : Sheet metal techniques such as stretching, bending and deep drawing. Many food-packaging tubs and containers are fabricated from Polypropylene. Forging is also used mainly for producing gears. 6. Cold Forming. All the cold working methods used for metals can be used for polymers. Filaments and fibres are produced by “Cold drawing”, that is, continuous stretch drawing. Conventional rolling can also be used for producing fibres. In “Cold pressing” or “Cold moulding”, the raw thermosetting material (or mixed plastic compounds) are put in the mould and pressed to shape at room temperature. The formed part is then removed from the mould and cured in an oven. Pressures applied by the press range from 14 MPa to 84 MPa. The moulds are made of abrasion resistant tool steel. The process is quite economical and the process cycle is relatively short. However, the surface quality and dimensional accuracy of the part is not very good. 7. Thermoplastic Stamping. Thermoplastic stamping or matched-die forming is a method in which filled thermoplastic polymer sheet at melt temperature is worked between mating dies. The cycle time is greatly reduced and the springback is minimum. 8. Spinning. The extrusion process can be modified to produce filaments, fibres and yarns. The molten thermoplastic polymer is extruded through a die containing many holes. For obtaining strands, the dies can be rotated to produce twists and wraps. 11.10.1. Comparison of Various Methods for Plastic Processing Process Characteristics of parts Tooling cost Production Surface produced rates Quality Injection Moulding Complex shapes of various sizes High High Good Extrusion Long, uniform hollow or solid Low ,, sections tolerances Thermoforming Shallow or relatively deep ,, Medium Good cavities Compression Moulding Similar to closed-die forgoing Medium ,, Good Transfer Moulding More complex than compression ,, High Good moulding Casting Intricate with flexible moulds Low Low Good Calendering Sheets Medium High Good Rotational Moulding Large hollow parts of relatively Low Low Good simple shapes Blow Moulding Hollow thin walled parts of Low High Good various sizes. Structural Foam Moulding Large parts with high stiffness to Medium Low Good weight ratio. 11.11. WELDING OF PLASTICS Most plastics are available in the form of mould powders or granules, which are then fabricated into finished articles. The principal processes for the manufacture of articles from plastic materials have been discussed earlier. Plastics differ drastically from metals in their behaviour, when