Histology Textbook PDF
Document Details
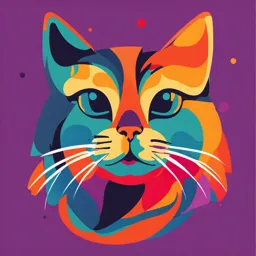
Uploaded by yvesss
Ayura 2027
Don W. Fawcett
Tags
Summary
This document is a section from a textbook on histology, specifically focusing on muscular tissue. It details the different types of muscle tissue found in vertebrates and the various characteristics of smooth muscle fibers, including their structure, organization, and functions. The section also covers the ultrastructure of smooth muscle and the diverse forms of organization seen.
Full Transcript
10 MUSCULAR TISSUE r Contractility is a fundamental property of power for mixing the ingested food wit...
10 MUSCULAR TISSUE r Contractility is a fundamental property of power for mixing the ingested food with di¬ protoplasm exhibited, in varying degree, by gestive juices and for its propulsion through nearly all cells. In cells of muscular tissue, the the tract. Smooth muscle is also found in the ability to convert chemical energy into me¬ walls of the ducts in the glands that are associ¬ chanical work has become highly developed. ated with the alimentary tract and in the walls Locomotion of multicellular animals, the beat¬ of the respiratory passages from the trachea ing of their hearts, and the movements of to the alveolar ducts. Blood vessels also have their internal organs depend on muscle of smooth muscle in their wall that controls their different types, each specialized for the kind caliber. of force or motion required. Three categories of muscle are distin¬ guished in vertebrates: smooth muscle, striated SMOOTH MUSCLE FIBERS muscle, and cardiac muscle. Smooth muscle is composed of fusiform, uninucleate cells that In the histological literature, the organiza¬ are not subject to voluntary control but re¬ tional subunits of all types of muscle have tra¬ spond to stimulation by the autonomic ner¬ ditionally been called fibers. Those of smooth vous system. Striated muscle, associated with muscle are fusiform or spindle-shaped with the skeleton, is innervated by the cerebrospi¬ their elongated nucleus situated in their wider nal nervous system and is responsible for loco¬ central portion (Fig. 10—1). They vary greatly motion and other voluntary movements. Its in their length in different organs. In the long cylindrical subunits, called muscle fibers, gravid human uterus, smooth muscle fibers are multinucleate syncytia containing closely may reach a length of 0.5 mm. In the wall of packed myofibrils, exhibiting along their length the intestine, their average length is about 0.2 alternating dark and light bands that are in mm, and in the walls of blood vessels, the register across the width of the fiber. This smallest smooth muscle fibers may be only 20 highly ordered arrangement results in a dis¬ /xm long. Where smooth muscle is organized tinctive pattern of repeating cross-striations in bundles, or extensive layers, the individual that are the basis for the term striated muscle. fibers are offset such that the wide portion of This type of muscle is also frequently called the cells are adjacent to the thin tapering ends skeletal muscle to distinguish it from cardiac mus¬ of neighboring cells. Therefore, in transverse cle which is also cross-striated but differs in sections, smooth muscle appears as a mosaic that its fibers are not syncytial but are made of rounded or polygonal profiles, varying up of uninucleate cellular units joined end- from one to several microns in diameter, with to-end. Cardiac muscle is not under voluntary nuclei found only in the larger profiles (Fig. control. It is endowed with an intrinsic system 10-2). The nucleus contains one or two for generating rhythmic contractions but re¬ nucleoli and its chromatin is distributed in ceives input from the central nervous system small clumps along the inner aspect of the influencing its rate. sunential ringle call, nuclear envelope. The major cell organelles multiple are confined to conical regions of cytoplasm nuclei extending in either direction from the poles SMOOTH MUSCLE of the elongate nucleus. The peripheral cyto¬ plasm, containing the contractile components, Smooth muscle forms the contractile por¬ generally appears rather homogeneous under tion of the wall of the digestive tract from the light microscope, but in favorable prepa¬ the middle of the esophagus to the internal rations studied at high magnification, longitu¬ sphincter of the anus. It provides the motive dinally oriented bundles of filaments can be 260 MUSCULAR TISSUE 261 Figure 10-1. Photomicrograph of a longitudinal section of Figure 10-2. Photomicrograph of a transverse section of smooth muscle from the tunica muscularis of the intestine, smooth muscle from the tunica muscularis of the human stomach. The section was stained with the periodic-acid- Schiff reaction which stains the glycoprotein of the lamina externa of the muscle cells, accentuating their outline. resolved. These can be revealed more clearly sue, or gathered together in slender fascicles, by their distinct birefringence, when exam¬ as in the tiny arrector pili muscle associated ined with the polarizing microscope. with each hair bulb, or in an annular band, In sections stained with the periodic-acid— as in the constrictor pupillae muscle of the Schiff reaction, the cytoplasm of the smooth iris. In precapillary arterioles, single circum¬ muscle cells in some organs may contain pink- ferentially oriented fibers are spaced at inter¬ staining deposits of glycogen. In preparations vals along the vessel. Their contraction nar¬ stained by this method, the individual smooth rows the lumen, decreasing blood flow muscle fibers are also outlined by a carbohy¬ through the capillary bed. In the wall of larger drate-rich external lamina, comparable to the arteries, flow is controlled by a continuous basal lamina of epithelia (Fig. 10—2). Silver layer of circular smooth muscle. In the wall impregnation methods reveal a delicate net¬ of the intestine, smooth muscle fibers form work of reticular fibers closely associated with two concentric layers with the fibers of the the external lamina enveloping each muscle inner layer oriented circumferentially and cell. those of the outer layer longitudinally. Con¬ traction of the inner layer constricts, and the outer layer tends to shorten the intestine. ORGANIZATIONAL DIVERSITY Their coordinated action produces peristaltic waves of contraction that are propagated Smooth muscle fibers are organized in dif¬ along the intestine to propel its contents. In ferent patterns to meet local requirements. other hollow organs, such as the urinary blad¬ They may occur singly in loose connective tis¬ der, smooth muscle is not organized in distinct 262 MUSCULAR TISSUE layers but in interwoven coarse bundles ori¬ ented in various directions. The force gener¬ ated by contraction of the fibers is transmitted through their investment of reticular fibers to the interstitial connective tissue, and thus spreads throughout the muscle mass, dimin¬ ~ ishing the size of the organ to void its contents. VARIATIONS IN FORM hitherto-up this to particular ti me Although smooth muscle is usually made up of fusiform cells of varying length, scanning microscopy, combined with methods for di¬ gesting the surrounding connective tissue, has revealed examples of morphological diversity that were hitherto unsuspected. The fibers in the urinary bladder and ductus deferens are often branched. In other organs, the fibers may have short lateral processes that contact neighboring cells. Where muscle fibers attach to the central tendon in the gizzard of birds, they have multiple finger-like processes at their ends resembling those seen on skeletal muscle fibers at myotendinous junctions. There are other cell types, of limited distri¬ bution, that differ from smooth muscle fibers in form but share some of their characteristics. In the mammary gland, myoepithelial cells of putative ectodermal origin have several long radiating processes that contain myofibril-like bundles of actin and myosin filaments. The cell processes embrace the acini of the gland and their hormonally induced contraction serves to expel the milk. Similar myoepithelial cells are associated with the secretory portions of the tubular sweat glands in the skin. Cells, called pericytes, are found at intervals on the surface of certain capillaries and postcapillary venules. They have a rounded cell body and several tapering processes that extend around the vessel. They have been little studied but are assumed to be capable of contracting to influence blood flow. ULTRASTRUCTURE OF SMOOTH MUSCLE In electron micrographs of low magnifica¬ tion, the juxtanuclear cytoplasm of smooth muscle fibers contains a small Golgi complex, a cluster of elongate mitochondria, a few short profiles of endoplasmic reticulum and many free ribosomes (Fig. 10-3). The bulk of the Figure 10-3. Micrograph of a smooth muscle cell in longitu¬ contractile cytoplasm presents a homoge¬ dinal section. The uniform gray region at the periphery is neous appearance, save for widely spaced, occupied by myofilaments that are not resolved at this magnification. A conical region of cytoplasm extending downward from the pole of the elongate nucleus contains numerous mitochondria and a few profiles of endoplasmic reticulum. MUSCULAR TISSUE 263 rather ill-defined fusiform densities termed The contractile units of smooth muscle are cytoplasmic dense bodies (Figs. 10-3 and 10-4). identified with difficulty. Short myosin fila¬ Similar densities, uniformly spaced along the ments (1.5-2.2 fim) seem to be intercalated inner aspect of the cell membrane, are called within bundles of relatively long actin fila¬ subplasmalemmal dense plaques. The cytoplasmic ments (—4.5 /xm) that probably correspond to dense bodies can be selectively stained with the myofibrils described with the light micro¬ fluorescent antibodies to the actin-binding scope. The ratio of actin filaments to myosin protein a-actinin (Fig. 10-5) and the dense ⑤ filaments is 12 to 1 to 14 to 1 in smooth muscle plaques, at the cell surface are reactive with (Fig. 10-6, inset) compared to 2 to 1 or 4 to antibodies to vinculin and talin. 1 in skeletal muscle. The orientation of the In micrographs of high magnification, bundles of filaments is longitudinal or oblique three kinds of filaments can be resolved: thin and there is no hint of lateral register of the actin filaments 4-8 nm in diameter; thicker myosin filaments such as that responsible for myosin filaments, about 15 nm in diameter; the cross-banding of skeletal muscle. The my¬ and 10-nm intermediate filaments. The latter ofibrils terminate in the cytoplasmic dense generally consist of desmin, a cytoskeletal pro¬ bodies and the actin filaments on the two sides tein characteristic of muscle, but they may be of a dense body are of opposite polarity. This vimentin in vascular smooth muscle. These fil¬ suggests that the dense bodies of smooth mus¬ aments occur in longitudinal or oblique bun¬ cle are the functional equivalent of the Z-lines dles that converge on, and attach to, the cyto¬ of skeletal muscle. There is a different molec¬ plasmic dense bodies and subplasmalemmal ular organization of the thick filaments in dense plaques to form a robust cytoskeletal smooth muscle. In isolated thick filaments, the network. Microtubules are rarely found in projecting heads of the myosin molecules, smooth muscle. which form cross-bridges to the surrounding Figure 10-4. Electron micrograph of smooth muscle in cross section. The cells are separated by wide intercellular spaces occupied by glycoprotein cell coats and small bundles of collagen fibers. Scattered through the cytoplasm are densities (at arrows) that are sites of lateral bonding of filaments of the cytoskeleton and of actin filaments. Those at the periphery of the cells are sites of attachments of filaments to the cell membrane. Fusiform densities, indicated by arrows, are sites of lateral bonding of bundles of myofilaments. 264 MUSCULAR TISSUE along tubular invaginations of the plasma membrane (T-tubules) to the reticulum in the interior of the muscle fiber. No comparable tubular invaginations of the membrane are found in smooth muscle fibers. However, a close relationship exists between the caveolae and elements of smooth endoplasmic reticu¬ lum that course among them, parallel to the surface. This has led to the suggestion that they may function in electromechanical cou¬ pling in a manner comparable to the interac¬ tion of T-tubules with the sarcoplasmic reticu¬ lum in skeletal muscle. This speculation is in need of further validation, because other in¬ vestigators contend that in such small fibers inward diffusion of extracellular calcium, ac¬ companying depolarization of the membrane, would be adequate to trigger contraction of smooth muscle. The argyrophilic reticulum observed around smooth muscle fibers, with the light microscope, is represented in electron micro¬ graphs by small bundles of slender collagen fibrils in the intercellular clefts. The cells are thus held apart over most of their surface by their external laminae and the intervening collagen fibers. However, in visceral smooth muscle these extracellular components are lacking at certain sites, permitting the mem¬ branes of adjacent cells to come into contact Figure 10-5. Confocal images of contracted, isolated smooth muscle cells labeled with fluorescent antibody to and form gap junctions. In certain other a actinin, showing the number and distribution of the cyto¬ smooth muscles, such low-resistance junctions plasmic dense bodies. (A) X1600, (B) X4400. (Micro¬ appear to be absent. graphs courtesy of Draeger, A. et al., 1990. J. Cell Biol. 11:2463.) PHYSIOLOGY OF SMOOTH MUSCLE actin filaments are found along their entire Smooth muscle differs considerably from length, whereas thick filaments of skeletal skeletal muscle in its physiology. Its contrac¬ muscle have a bare central segment that is tion is slow but it can be sustained for long devoid of cross-bridges. periods. It can shorten to one-quarter of its A distinctive feature of the ultrastructure resting length and can generate a force, per of smooth muscle fibers is the presence of a cross-section area, comparable to that of stri¬ great number of vesicular invaginations of the ated muscle, while consuming far less energy. plasmalemma (caveolae), associated with all How this economy of energy is achieved is not areas of the membrane between the subsur¬ entirely clear. The mechanism of contraction face dense plaques. These resemble vesicles is less well understood than that of skeletal involved in pinocytosis in other cell types, but muscle due, in part, to the difficulty of defin¬ in smooth muscle, there is no evidence that ing a contractile unit comparable to the sar¬ they detach and move into the cytoplasm. comere. It is generally assumed, however, that Their functional significance is puzzling. In shortening is produced by a similar sliding skeletal muscle, the coupling of excitation to of actin filaments with respect to the myosin contraction is dependent on release of free filaments. Its relatively slow speed and broad calcium from stores in a network of endoplas¬ range of shortening may be attributable to the mic reticulum (sarcoplasmic reticulum) that fact that the actin filaments are considerably surrounds the individual myofibrils. Calcium longer (4.6 /xm) than those of skeletal muscle release is triggered by impulses conducted (1.6 fxm). The force generated is believed to MUSCULAR TISSUE 265 Hit® Figure 10-6. Electron micrograph of a vascular smooth muscle cell in cross section, clearly showing thick and thin filaments. The inset shows a portion of cytoplasm at higher magnification. (Micrograph from Somlyo, A.P., E. Devine, and A.V. Somlyo. 1972. In Vascular Smooth Muscle. Heidelberg, Springer-Verlag.) be transmitted to the cell surface via the at¬ type of smooth muscle is responsible for the tachment of the contractile units to the cyto¬ waves of peristalsis that sweep along the intes¬ plasmic dense bodies at nodal points in the tine to advance its contents. Similar spontane¬ cytoskeleton. ous waves of contraction are observed in the The mechanism of triggering contraction is ureters and bile ducts. The mechanism of in¬ also different. The tropomyosin complex that trinsic excitation of the unitary smooth muscle is activated by calcium ions to initiate contrac¬ in the viscera is still poorly understood. How¬ tion of skeletal muscle is absent in smooth ever, its ability to perform slow, synchronized muscle. Instead, calcium diffusing into the cell contractions is attributable to the electrical with depolarization of the membrane, or cal¬ coupling of adjacent fibers by gap junctions cium released from the subplasmalemmal re¬ that permit the spread of excitation from fiber ticulum, binds to a protein calmodulin. The to fiber. Skeletal muscle fibers, on the other complex, so formed, activates ATPase in the hand, are electrically isolated from one an¬ heads of the myosin molecules, and cleavage other, with each requiring stimulation by its of ATP induces a conformational change in own neuromuscular junction. the myosin heads that results in translocation The smooth muscle in the walls of large of the adjacent actin filaments. arteries, in the ciliary body and iris of the eye, Smooth muscles in various tissues and or¬ and in the wall of the ductus deferens of the gans differ in their mode of activation. Vis¬ male reproductive tract is described as ceral smooth muscle, also called unitary smooth multiunit smooth muscle. In this kind of muscle, muscle, exhibits an autorhythmicity. Intrinsi¬ there is little evidence of impulse conduction cally generated stimuli are conducted, via gap from fiber to fiber via gap junctions. Instead, junctions, from cell to cell throughout a large each fiber is innervated and contraction is rel¬ area of muscle that contracts in unison. This atively rapid. Postganglionic autonomic nerve 266 MUSCULAR TISSUE fibers pass over the muscle surface or pene¬ extracellular matrix. An abnormal prolifera¬ trate into it, synapsing with fibers en passant. tion of smooth muscle cells and their produc¬ Smooth muscle in the walls of blood vessels tion of excess collagen contribute to the for¬ normally maintains a state of partial contrac¬ mation of atherosclerotic plaques in the aorta tion called muscle tonus, but this is subject to and coronary arteries of aging humans. modulation by blood-borne hormones. The cells have receptors for norepinephrine, an¬ giotensin, and vasopressin which stimulate STRIATED MUSCLE their contraction, resulting in vasoconstriction, a decrease in the caliber of the vessel. Other The units of organization of skeletal muscle substances, such as brandykinin and, prosta¬ are the muscle fibers, long cylindrical multinu- glandins produced locally in the tissues irri¬ cleated cells. They are much larger than gated, may result in loss of smooth muscle smooth muscle fibers, ranging from 10 to 30 tone and consequent vasodilatation. Response cm in length and from 0.1 to 0.5 mm in diame¬ to hormones is not confined to vascular ter. Parallel fibers are aggregated in bundles smooth muscle. Changes in circulating levels or fascicles large enough to be visible to the of estrogens during the menstrual cycle are naked eye. The individual fibers, the fascicles, reflected in variations in the actin and myosin and the muscle as a whole are invested by content of uterine smooth muscle, and during connective tissue that forms a continuous sup¬ pregnancy, the fibers undergo a remarkably porting framework. However, to facilitate de¬ hypertrophy. scription, its different portions are designated Smooth muscle fibers were formerly by separate terms. The dense connective tis¬ thought to be highly specialized cells incapable sue surrounding the whole muscle is called of proliferation, or of protein synthesis, other the epimysium. Thin septa that extend inward than that required to maintain their contrac¬ from it to surround each of the fascicles consti¬ tile components. It is now known that they tute the perimysium and the delicate reticulum synthesize laminin, collagen, elastin, and investing the individual fibers is the endomys- other components of the external lamina and mm (Figs. 10-7 and 10-8). Although the con- Figure 10-7. Photograph of a cross section of a human sartorius muscle showing the connective tissue of the epimysium around the entire muscle and the perimysium enclosing muscle fiber bundles of varyinq size (From Heidenhain 1911 Plasma und Zella 2 Leif. Jena.) ’ MUSCULAR TISSUE 267 capillaries are sufficiently tortuous to accom¬ modate changes in fiber length by straight¬ Myofibrils (1-2,/u) ening somewhat during relaxation and be¬ coming more contorted during contraction. The arrangement of the fascicles varies Nuclei of muscle fiber* from muscle to muscle. In some relatively short muscles, they are oriented parallel to the direction of pull and may continue without Muscle fiber crushed. Showing interruption throughout its length. In very Sarcolemma ( long muscles, fibers are usually shorter than the muscle as a whole and are connected to one or more transverse bands of connective Connective -tissue cells tissue spaced at intervals along the length of ( perimysi um) the muscle. In other muscles, described as uni- pinnate, the fasciculi are oriented obliquely Blunt end of fiber with respect to a longitudinal band of connec¬ tive tissue along one side of the muscle. Others are bipinnate, having oblique fascicles that ra¬ diate from a connective tissue core in the mus¬ cle, resulting in a pattern resembling that of the barbs extending obliquely from the axial core of a feather. A few muscles are multipin- Cohnheim’s fields nate, with oblique fascicles radiating from sev¬ of myofibrils eral longitudinal connective tissue strands Sarcolemma within the muscle. These strands converge on the tendon. Some loss of efficiency associated with oblique fascicular architecture is offset by the very large number of fibers that can be accommodated in this arrangement. It is generally found in muscles where consider¬ able power and a short range of motion are required. DEVELOPMENT AND REPAIR In the embryo, skeletal muscle develops from uninucleate precursors called myoblasts. These proliferate and fuse to form long multi- Figure 10-8. Drawing of the relationships of skeletal mus¬ nuclear cells that are often referred to as myo- cle fibers and the surrounding connective tissue forming the tubes, an unfortunate term that erroneously endomysium. (From Brodel, M. 1937. Bull. Johns Hopkins Hosp. 61:295.) implies that they have a hollow structure. These elongated syncytia then develop, in their cytoplasm, myofibrils, the contractile ele¬ ments of differentiated muscle fibers. During nective tissue serves to bind together the sub¬ postnatal growth, the muscle fibers increase units of the muscle, it permits a certain degree in length and thickness, reaching a diameter of freedom of motion between them. Some of 10-70 /am, depending on the muscle and longitudinal movement of fascicles with re¬ the species. Fibers in the same muscle may spect to one another is possible and each mus¬ vary considerably in their thickness. In adults, cle fiber can move independently of neigh¬ they may undergo further increase in diame¬ boring fibers. ter in response to prolonged and intense mus¬ The blood vessels supplying skeletal muscle cular activity—a phenomenon called hypertro¬ ramify in the epimysium and penetrate via phy of use. Conversely, the fibers may become the septa of the perimysium to form, in the thinner in muscle immobilized for a long pe¬ endomysium, a rich capillary network around riod by a cast applied in treatment of a frac¬ the individual muscle fibers (Fig. 10—9). The tured bone—a change called atrophy of disuse. 268 MUSCULAR TISSUE Blood corpuscles r —- r r ~t.. v. -1 0 SO 100 u >r-fTTjTrrT+ SC o' tOOO,U 0 SO iOCi. Figure 10-9. Drawing of the blood supply of muscle fiber bundles from the human rectus abdominis muscle (left) and the capillary network around the individual muscle fibers (right). (From Brodel, M. 1937. Bull. Johns Hopkins Hosp. In muscle of adults, occasional small cells THE STRUCTURE OF MUSCLE FIBERS with an elongate nucleus and a relatively coarse chromatin pattern can be found occu¬ Fresh muscle can be teased apart, under a dis¬ pying shallow depressions in the surface of secting microscope, into its individual fibers. At muscle fibers. These so-called satellite cells are higher magnification, these show a closely situated within the external lamina that sur¬ spaced transverse striation that is characteristic rounds the individual muscle fibers. They are of skeletal and cardiac muscle and is the basis for believed to be a residual population of myo- the term striated muscle used to distinguish them blast-like stem cells that persist in fully differ¬ from smooth muscle (Fig. 10-10). A fine longitu¬ entiated muscle and participate in muscle re¬ dinal striation is also detectable. Its structural ba¬ pair after injury. However, skeletal muscle has sis becomes apparent if samples of muscle are only limited capacity for repair in adults. After macerated in dilute nitric acid. This treatment minor injury, satellite cells, within an intact destroys cell membranes, extracts organelles, endomysium may divide and fuse to restore and cytoplasmic matrix. The contractile compo¬ damaged fibers. If the injury is more exten¬ nents left behind separate into thousands of thin sive, disrupting the endomysium, regenera¬ cross-striated myofibrils that appear to be made tion of muscle fibers does not occur. Instead, up of alternating short segments of differing re¬ the damaged area is invaded by fibroblasts fractive index. It is evident that the cross-stria- that deposit collagen, resulting in a fibrous tion of the intact muscle fiber is due to the fact scar. Restoration of muscle function after such that corresponding segments of the closely an injury depends mainly on hypertrophy of packed myofibrils are in register across the entire the remaining undamaged fibers. width of the fiber. MUSCULAR TISSUE 269 In the literature of histology, the plasma thereby protecting it against stresses devel¬ membrane of muscle fibers has traditionally oped during muscular contraction. In the X- been called the sarcolemma and the cytoplasm, linked heritary myopathy Duchenne muscular the sarcoplasm. These terms persist but no fun¬ dystrophy this protein is lacking resulting in damental difference from the corresponding structural weakness of the sarcolemma. Onset structures in other cells is implied by their use. of the disease is apparent by age 5, and there is The sarcolemma of the long cylindrical fibers a progressive replacement of damaged muscle is reinforced by an external lamina compara¬ fibers by connective tissue and adipose tissue. ble to the basal lamina of epithelia. Numerous By age 12 these individuals are usually unable nuclei, spaced along the length of the fiber, to walk. are displaced to the periphery by the column All of the common cell organelles are repre¬ of myofibrils that occupies the bulk of the sar¬ sented in the sarcoplasm. A small Golgi com¬ coplasm (Fig. 10—11). They are flattened plex is associated with one pole of most of the against the sarcolemma and, thus, present a nuclei. Mitochondria (sarcosomes) congregate long profile in section. Their number cannot in the juxtanuclear sarcoplasm and are also be specified because it varies from muscle to deployed in longitudinal rows between the muscle, but in a fiber several centimeters in myofibrils where they provide energy for length, the nuclei would number in the hun¬ muscle contraction. Lipid droplets are found dreds. The peripheral location of the nuclei is in small numbers between the myofibrils or useful in distinguishing skeletal muscle from among the mitochondria at the poles of the cardiac muscle in which the nuclei are central. nuclei. After staining with the periodic-acid- The cytoplasmic surface of the sarcolemma Schiff reaction, glycogen can be demonstrated in skeletal muscle is coated with the 400 kD throughout the sarcoplasm. In addition to protein dystrophin which appears to provide these microscopically demonstrable compo¬ mechanical reinforcement to the membrane, nents, the sarcoplasm contains, in solution, Figure 10-10. Photomicrograph of three muscle fibers in longitudinal section, showing the alternating dark, A-bands, and light l-bands. Preparation stained with iron hematoxylin. 270 MUSCULAR TISSUE accentuated, with heavily stained bands alter¬ nating with relatively pale bands (Fig. 10—12). The bands that appear dark in stained prepa¬ rations are anisotropic (birefringent) when ex¬ amined with the polarizing microscope and are, therefore, designated A-bands, whereas the lighter-staining bands are isotropic and are called the I-bands. The relative lengths of the bands depends on the state of contraction of the muscle. The I-bands are very short during contraction, longer during relaxation, and longest in muscle that has been passively stretched. The length of the A-bands remains constant in all phases of the cycle of contrac¬ tion and relaxation. Each I-band is bisected by a transverse line, the Z-line or Z-disc. The segments of myofibrils between successive Z-lines are called sarcomeres and all of the morphological changes during the contractile cycle are described with refer¬ ence to this structural unit. The sarcomere includes an A-band and half of the two contig¬ uous I-bands. The A-bands, I-bands, and the Z-discs are usually the only cross-striations vis¬ ible with the light microscope, but in excep¬ tional preparations a slightly paler zone, called the H-band, may be seen traversing the center of each A-band. The structural basis of the Figure 10-11. Photomicrograph of skeletal muscle fibers cross-striations of skeletal muscle becomes ap¬ in cross section, illustrating their polygonal outline, due to parent in electron micrographs and will be mutual deformation, and the peripheral location of their discussed below. nuclei. HETEROGENEITY OF SKELETAL myoglobin, an oxygen-binding protein which is MUSCLE FIBERS largely responsible for the slightly brown color of muscle. As required, oxygen dissociates The myofibers that make up a given muscle from myoglobin and becomes available for ox¬ are not all identical. They vary in diameter idations. Myoglobin is present in low concen¬ and in their cytochemical and physiological tration and is possibly of little functional sig¬ properties. The relative proportions of the nificance in the relatively pale muscles of different fiber types vary from muscle to mus¬ humans, but in the deeply colored muscle of cle. Two major categories of muscle fibers are diving birds and mammals, it is abundant and distinguished by physiologists: twitch fibers probably of greater physiological significance. (fast fibers) which propagate an action poten¬ In transverse sections, myofibrils occupy tial and respond with all-or-nqne contraction, most of the interior of the fiber and are re¬ and tonic fibers (slow fibers), which are unable solved as fine dots either uniformly distrib¬ to propagate an action potential and require uted or in polygonal areas formerly referred a series of nerve impulses. Their contraction to as the fields of Cohnheim. Whether the latter is more prolonged than that of twitch fibers. represent the true distribution of myofibrils By these criteria, slow fibers are common in or are a result of shrinkage during specimen amphibian and reptilian muscle but rare in preparation was long debated. The weight of mammals. The discussion that follows will re¬ evidence now favors their interpretation as a fer to heterogeneity among twitch fibers, shrinkage artifact. which are the dominant physiological type in In longitudinal sections, stained with iron mammalian skeletal muscle. haematoxylin, the cross-striations that are Mammalian muscle fibers vary in diameter barely detectable in fresh muscle are greatly over a narrow range, but other differences are Figure 10-12. Electron micrograph of skeletal muscle, extracted with glycerin to improve the contrast of the myofibrils. Observe the uniform diameter of the myofibrils and the location of the nucleus immediately beneath the sarcolemma. Corresponding bands of the myofibrils are usually in register across the muscle fiber. Where they are out of register, as at the upper left, this is thought to be an artifact of specimen preparation. 271 272 MUSCULAR TISSUE Structural and Functional Characteristics of Muscle Fiber Types White Red Intermediate Fast twitch Slow-twitch Fast-twitch Fiber Type glycolytic (FC) Oxidative (SO) oxidative, glycolytic (FOG) Structural Characteristics Color White Red Pink Fiber diameter Large Small Medium to small Mitochondria Few Many Many Capillary density Sparse Rich Rich Metabolic Characteristics Twitch rate Fast Slow Fast Rate of Fatigue Fast Slow Intermediate Primary pathway for ATP synthesis Anaerobic Aerobic Aerobic Myosin ATPase activity Fast Slow Fast Myoglobin content Low High High Histochemistry Glycogen content High Low Intermediate Neutral fat content Low High Intermediate ATPase, pH 9.4 High Low High ATPase, pH 4.3 Low High Low Succinic dehydrogenase Low High Medium to high NADH dehydrogenase Low High Medium to high Figure 10-13. Table presenting the structural, metabolic, and histochemical characteristics of the three types of skeletal muscle fibers. At the top of the columns are the traditional terms (red, white, and intermediate) and the corresponding terms employed by physiologists. (Modified after Burke, R.E. 1981. In Handbook of Physiology, The Nervous System II, pp. 345-422. American Physiology Society, Bethesda and Table 9-2, from Morieb, E.N. 19xx. In B. Cummins, ed. Human Anatomy and Physiology. not apparent in routine histological sections. However, with cytochemical staining meth¬ ods, three fiber types are easily distinguished. These have traditionally been designated red fibers, white fibers, and intermediate fibers. Regret¬ tably, some terminological confusion has re¬ sulted from recognition of four types by physi¬ ologists and by their use of descriptive terms based on different criteria from those used by histologists. The divergences in terminol¬ ogy are set forth in the table in Fig. 10-13. Red fibers (slow twitch, oxidative fibers) are relatively small in diameter and have a darker color, attributable to their greater content of myoglobin and rich supply of capillaries. They have numerous large mitochondria beneath the sarcolemma and between the myofibrils. Lipid droplets are common in the sarcoplasm of these fibers and, owing to the abundance of their mitochondria, they stain intensely with the cytochemical reaction for the enzyme succinic dehydrogenase (Fig. 10-14). The Z- bands tend to be wider than those of other fiber types. They are innervated by small axons with small and relatively simple motor end plates. Motor units consisting of red Figure 10-14. Photomicrograph of several skeletal muscle fibers contract more slowly than other types fibers in cross section, stained for the mitochondrial en¬ and are very resistant to fatigue because of zyme succinic dehydrogenase. Three categories of fibers their ability for oxidative regeneration of are distinguished: small red fibers rich in peripheral mito¬ chondria; large white fibers, with relatively few mitochon¬ ATP, the high-energy compound needed to dria; and fibers with intermediate characteristics. (Photomi¬ recycle the contractile process. These proper- crograph courtesy of G. Gautier.) MUSCULAR TISSUE 273 ties make them particularly effective in pos¬ tural maintenance. The white fibers (fast-twitch fibers) are the largest of the fiber types. Their subplasma- lemmal mitochondria are smaller and less nu¬ merous than those of red fibers and interh- brillar mitochondria are relatively few. Their cytochemical reaction for succinic dehydroge¬ nase is correspondingly weak (Fig. 10-14). Their generation of ATP depends on anaero¬ bic glycolysis of glucose derived from abun¬ dant deposits of glycogen in the sarcoplasm. White fibers are innervated by larger axons that form motor end plates about twice the size of those of red fibers. They contract rap¬ idly and generate a large force but they fatigue rapidly. They are best suited for brief bursts of intense muscle activity. The form and disposition of mitochondria in the three types of muscle fibers has been studied by held emission scanning electron microscopy, after removal of the cytoplasmic matrix (Fig. 10—16). In red fibers, spherical subsarcolemmal mitochondria are large and abundant. Slender, paired mitochondria are transversely oriented on either side of the Z- lines. In the interhbrillar spaces, mitochon¬ Figure 10-15. Succinic dehydrogenase reaction in skeletal dria, one sarcomere in length, form longitudi¬ muscle. (A) Plantaris muscle of a control guinea pig. nal columns. These may be thick, consisting (B) Same muscle of an animal after 8 weeks of running of multiple mitochondria, or thin, consisting on a treadmill. The fiber population of the exercised muscle of rows of single mitochondria (Fig. 10—16A). is more homogeneous—nearly all fibers are small and rich in mitochondria. (Photomicrograph from Faulkner. J.A. In white fibers, subsarcolemmal mitochondria 1944. In Podolsky, R.J., ed. Contractility of Muscle Cells are fewer and longitudinal columns are rare and Related Processes. Englewood Cliffs, NJ, Prentice- and often interrupted (Fig. 10—16B). Mito¬ Hall.) chondrial disposition in intermediate fibers is similar to that of red fibers, but thick mito¬ chondrial columns are rarely found. A subclass of fast-twitch fibers, recognized axon. The number of hbers innervated by a by physiologists, are relatively rich in myoglo¬ single motor neuron varies from 100 to 300, bin and have a capacity for aerobic glycolysis, in the small muscles of the hand, to 600—1700, but also have considerable capacity for anaer¬ in large muscles of the arm and leg. The mus¬ obic metabolism and are relatively resistant cle hbers in any one motor unit are not to fatigue. Such fibers are classified by some grouped together but are scattered over a con¬ authors as fast-twitch fatigue resistant fibers (FR) siderable area which may be shared by 20 or and by others as fast-twitch oxidative glycolytic more other motor units. The intermingling fibers (FOG). A fourth class intermediate be¬ of the hbers of many different motor units tween these and fast fatiguable twitch-hbers explains the differences in staining reactions has also been described. It is not entirely clear of neighboring hbers (Fig. 10-15). how many of these subclasses fall within the When muscles are examined with the naked histologist’s category of intermediate fibers, eye, they vary somewhat in color. The differ¬ which are simply defined as those that are ence between the white and dark meat of a intermediate between red and white hbers in chicken is a striking example. In mammalian diameter, mitochondrial volume, and cyto¬ muscles, slight differences in color are also chemical staining intensity. detectable. These variations are a reflection Muscle hbers do not contract individually, of differences in vascularity and differing pro¬ but in motor units, groups of hbers of the same portions of the hber types. The ratio of hber type innervated by branches of the same nerve types in any given muscle is fairly constant, 274 MUSCULAR TISSUE to a slow postural muscle and a fast locomotor muscle are exchanged, there is gradual change in the structural and physiological characteristics of both muscles to those of the other type. ULTRASTRUCTURE OF STRIATED MUSCLE FIBERS Our understanding of the mechanism of shortening and the generation of force by skeletal muscle has been greatly advanced by examination of the fibers with the electron microscope. The structure of the common or¬ ganelles is not fundamentally different from that in other cells. However, consistant with the high-energy requirement for contraction, the mitochondria have numerous closely spaced cristae and their distribution, in inti¬ mate relation to the myofibrils, brings the source of energy (ATP) close to its site of utili¬ zation. The endoplasmic reticulum is highly organized and has acquired physiological properties not typical of this organelle in other cell types. In the latter part of the nineteenth century, a cytologist examining muscle that had been impregnated with a heavy metal described a lace-like network of blackened strands that appeared to surround each of the myofibrils. This he called the sarcoplasmic reticulum. Ef¬ forts of others to demonstrate it were only partially successful and its validity remained in doubt until it was rediscovered with the electron microscope in 1954. It consists of membrane-bounded tubules that form a con¬ tinuous network occupying the narrow spaces between the myofibrils throughout the muscle fiber. Although it corresponds to the endo¬ plasmic reticulum of other cells, it is largely devoid of associated ribosomes and is special¬ ized for a different function. It is the site of sequestration of calcium during muscle relax¬ ation and for release, into the sarcoplasm, of the free calcium ions that trigger contraction Figure 10-16. The three-dimensional organization of the in response to a nerve impulse. It is, therefore, mitochondria in red muscle fibers (A) and white fibers (B). essential for the termination and initiation of N: nucleus; SM: subplasmalemmal mitochondria; CK: thick mitochondrial column; CN: thin mitochondrial column; IM: muscle contraction. The reticulum around l-band-limited mitochondria. (Drawings from Ogata, T. and each myofibril exhibits a repeating pattern of Y. Yamasaki. 1985. Cell Tissue Res. 241:251.) local differentiations, related to specific re¬ gions of the sarcomeres. The prevailing orien¬ tation of the sarcotubules is longitudinal, but but it is not immutable. It has now been shown anastomosing lateral branches form a denser that fast-switch fibers can be transformed to network encircling the myofibril at the level slow twitch fibers, and vice versa. If the nerves of the H-zone of each A-band. At the junction MUSCULAR TISSUE 275 of the A-band with the I-band, the longitudi¬ ferred to as the triad. Like the sarcomeres, the nal sarcotubules are confluent with a trans¬ triads are in register across the entire width versely oriented tubule of larger caliber, called of the muscle fiber. The above description the terminal cisterna. The longitudinal tubules applies to skeletal muscle of all mammals. In that span successive I-bands and the interven¬ amphibia, the triads are located at the Z-line ing Z-line also terminate in a separate terminal instead of at the A—I junction (Fig. 10-18). cisterna at the A-I junction. Thus, a pair of The sarcoplasmic reticulum is continuous parallel terminal cisternae is associated with throughout the muscle fiber, but two func¬ each A—I junction along the length of the my¬ tionally distinct regions are now distin¬ ofibril, two pairs to each sarcomere. Between guished. The expanded transverse cisternae each pair of terminal cisternae, there is a slen¬ that are associated with the T-tubules to form der transverse tubule (T-tubule) that is not an triads, are designated the junctional reticulum. integral part of the sarcoplasmic reticulum but It has properties that enable it to respond to is a long tubule extending inward from the an action potential, spreading inward along sarcolemma (Fig. 10-17). At the surface of the adjacent T-tubule, by releasing calcium the muscle fiber, its limiting membrane is con¬ into the sarcoplasm. The tubules making up tinuous with the sarcolemma and its lumen the remainder of the reticulum are commonly opens onto the extracellular space. Thus, the referred to as longitudinal sarcotubules, even T-tubules are slender invaginations of the sar¬ though they have lateral branches that form colemma that penetrate deep into the interior a network around the A-bands of the myofi¬ of the muscle fiber crossing many myofibrils. brils. In freeze-fracture preparations, the The two parallel terminal cisternae and the membrane of these tubules is rich in intra¬ intervening T-tubule form a complex re¬ membrane particles that are thought to con- Figure 10-17. (A) Micrograph of a small area from a longitudinal section of skeletal muscle, passing tangenital to a myofibril. Observe the longitudinal tubules of the sarcoplasmic reticulum and two transversely oriented triads at the level of the A-I junctions. Glycogen particles are present among the sarcotubules. (B) Longitudinal section of muscle that has been immersed in peroxidase. The dense reaction product of the peroxidase is present in the lumen of the two T-tubules, verifying the continuity of their lumen with the extracellular space. (Micrograph courtesy of D. Friend.) 276 MUSCULAR TISSUE SKELETAL MUSCLE Figure 10-18. Schematic drawing of the distribution of the sarcoplasmic reticulum around myofibrils of amphibian skeletal muscle. The longitudinal sarcotubules are confluent with transverse terminal cisternae. A slender transverse T-tubule extending inward from the sarcolemma is flanked by two terminal cisternae to form the “triads”'of the reticulum. In amphibian muscle, depicted here, the triads are at the Z-lines. In mammalian muscle, there are two to each sarcomere, located at the A-l junction. (From McNutt, S. and D.W. Fawcett. 1969. J. Cell Biol. 42:46; modified from Peachey, L. 1965. J. Cell Biol. 25:209.) tain the Ca++-Mg++-ATPase responsible for junctional reticulum, on the other hand, con¬ transporting calcium from the sarcoplasm tains an amorphous material of low density back into the lumen of the reticulum during that consists mainly of calciquestrin. This 55 muscle relaxation. The contents of the longi¬ kD protein can bind 300 nM of calcium per tudinal sarcotubules is extracted in specimen milligram and is believed to serve as a seques¬ preparation and their lumen generally ap¬ tering agent for the storage of calcium within pears empty. The lumen of cisternae of the the reticulum. MUSCULAR TISSUE 277 excitation-contraction isolated T-tubules and junctional reticulum. COUPLING Two proteins have been isolated that are be¬ lieved to be involved in signal transduction at The term excitation-contraction coupling the junction. An 1800 kD protein composed refers to the sequence of electrical and chemi¬ of four identical subunits has been extracted cal events leading to the activation of muscle from terminal cisternae. It binds ryanodine, a contraction. Some of these have already been plant alkaloid known to release calcium from alluded to, but a brief overview of the process the sarcoplasmic reticulum. In electron micro¬ may facilitate understanding of additional de¬ graphs, this protein has an appearance identi¬ tail to follow. In brief, it begins at the myoneu¬ cal to that of the junctional feet in situ. A ral junction with the generation of an action second protein of 212 kD, extracted from T- potential that spreads over the sarcolemma tubules, binds 1,4,dihydroxypyridine, a known and along the membrane of the T-tubules into voltage dependent blocker of Ca++ channels. the interior of the muscle fiber. This activates In electron micrographs, this protein matches events at the interface between the T-tubules the tetrads seen in freeze—fracture prepara¬ and the terminal cisternae of the triads that tions of T-tubules. It is believed to sense the result in the rapid release of calcium from the voltage change associated with depolarization sarcoplasmic reticulum. The calcium set free of the T-tubule membrane and to activate in the sarcoplasm binds to the myofibrils and opening of the Ca++ channels in the junctional triggers their shortening. When depolariza¬ feet. tion of the sarcolemma by nerve impulses ceases, calcium is actively transported back into the lumen of the terminal cisternae. The SUBSTRUCTURE OF THE MYOFIBRILS consequent lowering of the calcium concen¬ tration around the myofibrils brings about The smallest units of the contractile mate¬ muscle relaxation. rial of muscle that are visible with the light In recent years, the structural and chemical microscope are the myofibrils (Fig. 10-20D), components of the triad involved in excita¬ but in electron micrographs, these are found tion-contraction coupling have been pursued to be composed of even smaller units, the myo¬ down to the molecular level. In electron mi¬ filaments (Fig. 10-20E). These are of two crographs of high resolution, the T-tubules kinds, thin actin filaments and thicker myosin and adjoining cisternae are seen to be joined filaments. The cross-banded pattern of striated by small regularly-spaced electron-dense muscle is due to a highly ordered arrange¬ structures that bridge the 15-nm gap between ment of interdigitating sets of these filaments. their membranes. These so-called junctional Myosin filaments, 1.5 ,u,m in length and 15 feet (also called spanning proteins or junctional nm in diameter, are oriented longitudinally in channel complexes) are arranged on the surface parallel array and spaced about 45 nm apart. of the cisterna in two or three rows, parallel They are the principal constituent of the A- to the long axis of the T-tubule. In sections bands of the sarcomeres. Each is slightly tangential to the junctional face of the cis¬ thicker in the middle and tapers toward its terna, each foot appears to be made up of four ends. They are held in lateral register by slen¬ subunits about 14 nm in diameter arranged in der cross-links in the middle of the A-band. a quadrifoil configuration. In freeze—fracture The transverse alignment of these links cre¬ preparations, the intramembranous domain ates a thin linear density called the M-line that of these complexes is represented by groups bisects the paler H-band at the middle of each of four rounded profiles projecting from the A-band (Fig. 10-20F). Actin filaments are the inner surface of cleaved junctional mem¬ dominant component of the I-bands, but at brane. Images of cleaved T-tubule membrane their ends, they interdigitate with myosin fil¬ reveal groups of four particles that have the aments in the neighboring A-bands to varying same center-to-center spacing as the subunits degrees depending on the state of muscle con¬ of the junctional feet. These are also arranged traction. It follows that in cross sections at the in longitudinal rows and are believed to be in level of the I-band, only punctate profiles of contact with the subunits of foot proteins that thin actin filaments are found (Fig. 10-20G), span the gap between the junctional mem¬ whereas in cross sections near the middle of branes of the triads.(Fig. 10—19) the A-band, only larger punctate profiles of These ultrastructural observations have myosin filaments are found (Fig. 10-20H). been supplemented by biochemical studies on However, in cross sections through the region 278 MUSCULAR TISSUE Triad T-Tubule Junctional T-Tubule Triad reticulum (freeze-cleaved) Figure 10-19. (A) Diagram showing the location of the triads. (B) Triad at higher magnification showing the so-called feet connecting the cisternae to the T-tubule. (C) Drawing of the freeze-fracture appearance of the junctional surface of a terminal cisterna, showing the groups of four subunits of the feet. The lipophilic intramembrane portions of these subunits surround the calcium-release channel. (D) Appearance of freeze-fracture image of the T-tubule, showing diamond-shaped clusters of particles that correspond in their distribution to that of the protein subunits of the feet. These presumably detect the depolarization of the membrane and transmit a signal to the foot processes that results in opening of the calcium channels. (Figure based on diagrams in Block, B.A. et al. 1988. J. Cell Biol. 107:2587.) of filament interdigation, near the ends of the correspond to the cross-bridges seen between A-band, both kinds of filaments are found, thick and thin filaments in intact myofibrils. with thin filaments in hexagonal array around Further dissociation of a thick filament yields each myosin filament (Fig. 10—201, 20-21). In about 350 myosin molecules, each 300 nm long longitudinal sections, the 10—20-nm interval and 2—3 nm in diameter. These, in turn, con¬ between thick and thin filaments is traversed sist of two a-helical polypeptide chains with a by regularly-spaced cross-bridges radiating globular region at one end. The two chains from each myosin filament toward the neigh¬ are entwined to form the straight, rod-like boring actin filaments (Figs. 10-20F, 10—23 “tail” of the molecule, whereas the globular and 10—24). Little additional detail can be seen regions diverge to form two “heads,” corres¬ in routine electron micrographs of thin sec¬ ponding to one of the cross-bridges of an in¬ tions, but analysis of the myofilaments has tact myosin filament. The junction of the head been carried further by their isolation and and tail of the molecule is flexible, permitting examination at high magnification after shad¬ a change in configuration which, as will be owing with heavy metals and with negative- shown later, results in movement of the adja¬ staining procedures. cent actin filament (Fig. 10-25). Enzymatic Isolated thick filaments have a smooth cen¬ proteolysis of the myosin molecule cleaves it tral segment, but toward either end, they bear into two fragments, a straight portion called short lateral projections 14 nm apart. These light meromyosin (LMM), representing the MUSCULAR TISSUE 279 E Myofilament* Actin Filam«nrt CroM UcUm I Bc*d Cross Sscttoo H Bond Cross Ssctton A Bond Figure 10-20. Diagram of the organization of skeletal muscle from the gross to the molecular level. F, G, H, and I show the arrangement of filaments in cross section at the levels indicated in E. (Drawing by Sylvia Colard Keene.) greater part of the tail, and a shorter segment ments attach to the filaments decorating them called heavy meromyosin (HMM), which in¬ in a distinctive arrow-head pattern. This cludes the heads and a short portion of the clearly demonstrates, in vitro, the acdn-bind- backbone of the molecule. When a heavy ing property of the heads of the myosin mole¬ meromyosin fraction is added to isolated actin cules that is essential for muscle contraction. filaments, large numbers of these HMM frag¬ Under appropriate physicochemical condi- W 'd.:> J*. < ®P T. ** * A , * fK *. « 'H # ^ *f H 9 *. *>* -.I**9*T*i *T*# T*9 f.-A... % * ** /* * 9* * id * m * ® * & * A * »#* * *'* m * ip* ' *9® * * vX‘ — » « ^ * *'. jjfct: * # A * »;#.* * #'is * *;«* * I *: * >' .*.*. * ,9# m *.. *dk’ , *9* * " a * ? * * 7*.-d.« . «fc '5- * * * * * *-*. ^*;*%*? *"* * A * m. * 9 * d* * ‘ * ' 9-~ *% “"*'d T ** * *#'*^,‘*d* 9' i *« * *# *”#’ #7 > V# *. , !, 1 i,' > # W I 9 * * " d * ~ A * # "‘ ts “*“9***9* *:;>..#. _* **;*>: ->**>;»>.; * ; : *:«; %*,.. * -#* * **c * * *0\ f! i *«* i.!#« * 4 , ,**©,* *#»,*** * * * 9* * *4 , ,# * Jm, I #.v O * 9. * 9,, *. # 4? * j®s» * #9 * # ' * ” a ‘ # ' # ' *» * * ' m * * * # * » m » # ; > * r»» * O * ft 41. t » * f * * * „#.. f „ d *> ^ *. *. J, * *..#,.» » d f * ,d * Id * 9 ' * *## * " * * 49* * 7 * * # * 9 ^ #> w* * "* -* * ® ~ ’- -w-v 9 * * * 'mt-v '' / T *. * *4i *.d. #^#. « d, « d_ * ~ d * * d t # * a,®. * L #; »;#; *: *:* * **k‘ _*P ^ # *.^iP im *. * _ p| m p ^. M * 9 „ ,. _ 9 *.9 * 9 * t;f>:*:9; #' >*. 9. >; 9 «*^i;*;#. ld * d * ,** *t *!d * * d 1 * I f , * * * - * 9 *,9 **9' ’ *.9. f , ,'*, 9 ' 9 ‘ Id. * , , *.9, * * 9* * 9- * 9* * ‘ 9 * 9 * *91 * - 9. * #* 9 * 9 * ' % « ,«,' 9* * * * * * ® * *:# *;# **.*„d, ,«.»*.T'a-,*9*. # A. ’ m" * m * *m * M * A- * a # 9 « tt * 9 * d» * * 9 » * ,9 » , m * 1 * * 1 * *. i * , * *** *A'***9 * * 9 * 9 * , d # * 9 ^ * gL » :' * ^ d pi.... f. ^ w *.*. * Id. * ' w #' : 1 ,9, * * *?*«* :*A* * *9 d, % f * t ** ^ 9* , * „ *.9* * * d-* *, »*.»... *. ” * -m. * '«k 1 wt. *: 9 '. i 9 #. ** 9* « -9 * 9 * J9* « »d Figure 10-21. Micrograph of a cross section through the A-band of insect flight muscle at high magnification showing the orderly arrangement of actin filaments around the larger myosin filaments, as in Fig. 10-20, I. The pattern is similar in vertebrate muscle but it usually does not exhibit such a highly ordered “crystalline” lattice, and the myosin filaments do not appear hollow in cross section in mammalian muscle. (Micrograph courtesy of H. Ris.) Figure 10-22. Electron micrograph of several juxtanuclear myofibrils labeled to indicate the various bands in the normal pattern of cross-striations in a relaxed mammalian muscle. 280 Figure 10-23. Micrograph of the major portion of one sarcomere of insect flight muscle. Cross-bridges between the think and thin filaments are just detectable. These are more apparent in the Fig. 10-24, an area comparable to that outlined in the square on this figure. (High-voltage micrograph courtesy of H. Ris.) Figure 10-24. Micrograph of an area of skeletal muscle prepared by rapid freezing in liquid helium, deep etching, and rotary-shadowing. The cross-bridges between the myosin and actin filaments are clearly visible (at arrows). (Micrograph courtesy of N. Hirokawa and J. Heuser.) 281 282 MUSCULAR TISSUE mers (G-actin), 5.6 nm in diameter, into two strands helically entwined, with each gyre about 36 nm in length. Study of polymeriza¬ tion of G-actin, in vitro, has shown that the monomers are consistently oriented, giving the resulting filaments a definite polarity. Within the myofibrils, in vivo, the actin fila¬ ments on either side of the Z-discs are of oppo¬ site polarity. Associated with the actin fila¬ ments are molecules of tropomyosin, a protein about 40 nm in length consisting of two poly¬ peptide chains in a-helical configuration. These long molecules are arranged end-to- end in the grooves between the helically en¬ twined actin chains (Fig. 10-25). Bound to each molecule of tropomyosin, at regular in¬ tervals of about 40 nm, is a complex of three troponin peptides (Tn-T, Tn-I, and Tn-C) that have distinct functions. Tn-T binds the com¬ plex to tropomyosin, Tn-C has a binding site for calcium, and Tn-I inhibits binding of myo¬ sin heads to actin in resting muscle. These submicroscopic components of the thin fila¬ Figure 10-25. (A) Schematic representation of the ar¬ ments have key roles in the sliding filament rangement of myosin molecules in a segment of a thick mechanism of muscle contraction that will be filament, and the double helical configuration of the actin discussed later in this chapter. filament with its periodic associated tropomyosin and tropo¬ nin complexes. (B) In the current interpretation of the mech¬ Muscle exhibits a resistance to stretch that is anism of movement of the actin filaments, calcium binding independent of the interaction of actin and causes a change in the configuration of the tropomyosin- myosin filaments and a third type of filament, troponin complexes, exposing the myosin-binding sites on responsible for this passive elasticity, was pos¬ the actin filament. The myosin heads, energized by ATP, then change their angle to bind and move the actin filament. tulated. This has now been verified by the dis¬ covery of very thin filaments that extend from the A-band to the Z-line. They are seldom resolved in routine electron micrographs, but tions, myosin molecules will self-assemble in when muscle is greatly stretched, they can be vitro to form myosin filaments. In so doing, seen in the gap created between the ends of they assume an antiparallel arrangement with the thick and thin filaments. In addition, if the rod portions toward the center of the na¬ the actin filaments are selectively depolymer- scent filament and the heads toward its ends. ized with gelsolin, delicate strands can be The overlapping molecules are staggered so seen extending the full length of the I-band. that the projecting heads recur at intervals of These extremely thin strands (~ 4 nm) consist 14 nm and each is rotated 120° with respect of titin, an exceptionally large protein having to its neighbors. This results in a spiral disposi¬ a molecular weight of about 3 x 106 D. The tion of the heads along much of the filament molecules are approximately 1 /zm long, span¬ toward either end. A central segment con¬ ning the distance from the M-line of the A- sisting only of the overlapping antiparallel rod band to the Z-disc (Fig. 10-26). The portion of portion of the molecules is devoid of bridges. the molecule that extends into the A-band may In intact myofibrils, the lateral register of be an integral part of the thick filaments. There these smooth segments of the myosin fila¬ are probably six per filament. The I-band re¬ ments results in the paler H-band in the center gion of the molecules forms an elastic connec¬ of the A-bands (Fig. 10-22). tion between the thick filaments and the Z-line. Thin filaments isolated by dissociation of Titin is the main structural basis for myofibril¬ myofibrils are about 1 /xm in length and con¬ lar elasticity. In addition, it is believed to main¬ sist predominantly of filamentous actin (F-ac- tain the central position of the thick filaments tin). At very high magnification, they have a in the sarcomere. This is supported by the ob¬ slightly beaded appearance because they are servation that, after radiation damage to titin, formed by polymerization of globular mono¬ the central position of the A-band is lost. MUSCULAR TISSUE 283 Extent of one titin molecule Figure 10-26. Diagram illustrating the probable arrangement of titin molecules within the sarcomere. The elastic part of the molecule is shown only in the l-band. (From Trinnick, J. 1991. Current Opinion Cell Biol. 6:112.) The myofilaments of successive sarcomeres are linked end-to-end by the Z-disc. The struc¬ tural basis for this linkage is still a matter of debate because, in electron micrographs, the density of the disc and the superimposition of its components in sections of normal thickness makes its substructure difficult to analyze. In very thin sections of muscle from a species with a relatively simple disc, the actin filaments appear to terminate at the edge of the disc. There, each filament is attached to four thin diverging Z-filaments. These course obliquely across the disc to attach to four actin filaments A of the next sarcomere. In transverse sections passing through the disc, the ends of the actin filaments, and the Z-filaments connecting them project a square lattice (Fig. 10—27). The filaments of one sarcomere are slightly offset with respect to those approaching the disc from the other side. Therefore, in longitudi¬ nal sections, the connecting Z-filaments form a zigzag pattern across the myofibril. The chemical nature of the Z-filaments has yet to be established, but other important compo¬ nents of the disc have been identified. Much B of its electron density is attributed to a-actinin, Figure 10-27. Diagram illustrating one interpretation of a 100 kD actin-binding protein which is also the structure of the Z-disc. At (A) the actin filaments termi¬ involved in the end-to-end linkage of actin nate at the edge of the Z-disc, where each is attached to filaments in the cytoplasmic dense bodies of four diverging Z-filaments, coursing across the disc to at¬ smooth muscle. A 200 kD protein, zeugmatin, tach to four actin filaments of the next sarcomere. (B) In transverse sections through the disc, the ends of the actin can be localized in two parallel lines along the filaments and the Z-filaments, project a square lattice. boundaries of the disc. Another actin-binding (After Kelly, D.E. and M.A. Cahill. 1972. Anat. Rec. protein, filamin, originally isolated from 172:623.) 284 MUSCULAR TISSUE smooth muscle, is also found in the Z-discs of the thin filaments are moved relative to the skeletal muscle. The respective roles of these thick filaments, sliding more deeply into the proteins in maintaining the structural integ¬ A-bands, thus shortening the sarcomeres rity of the disc has yet to be worked out. along the entire length of the myofibrils (Fig. A complex cytoskeleton of sarcoplasmic in¬ 10-28). Consistent with this interpretation are termediate filaments supports the myofibrils the changes in length of the H-band in differ¬ and maintains the transverse alignment of sar¬ ent phases of the contractile cycle. Its dimen¬ comeres that results in the characteristic cross- sions are determined by the distance between striated appearance of skeletal and cardiac the ends of the actin filaments that extend into muscle. A ring of interwoven filaments encir¬ the A-band from opposite ends. Therefore, it cles the myofibrils at each Z-line and the rings is widest in resting muscle and becomes nar¬ around the Z-lines of successive sarcomeres rower in contracted muscle, owing to the are connected by longitudinal filaments in the deeper penetration of the actin filaments into intermyofibrillar spaces. Thus, each myofibril the A-band. is enclosed in a delicate sleeve of 10-nm fila¬ The only visible structures that could pro¬ ments and the collars around the Z-lines of duce the observed displacement of the actin neighboring myofibrils are interconnected by filaments are the heads of the myosin mole¬ short transverse filaments. Beneath the sarco- cules that form cross-bridges between the two lemma, there are inconspicuous rib-like sets of filaments. It is assumed that it is these bands, called costameres, seen in longitudinal that develop the force necessary for actin fil¬ sections as small densities that repeat along ament sliding. During contraction, the dis¬ the fiber in register with the Z-lines of the placement of the actin filaments may be as peripheral myofibrils. These appear to be sites much as 300 nm in each half-sarcomere, where the myofibrils are loosely connected to whereas the movement of the distal ends of the sarcolemma by filaments of the cytoskele¬ the bridges could be no more than 10 nm. ton. In contracted or swollen muscle fibers, Therefore, hundreds of bridge-forming and indentations occur along the length of the fi¬ bridge-breaking cycles must take place to pro¬ ber at these subsarcolemmal densities which duce the observed displacement of the actin can be shown to contain the anchoring pro¬ filaments. tein, vinculin. The contribution of this elabo¬ The observation that the distance between rate cytoskeleton to the active tension and pas¬ filaments, in some phases of contraction, ap¬ sive elasticity of muscle has yet to be explored. peared to be greater than the length of the myosin heads, initially cast some doubt on their involvement in translocation of the actin SLIDING FILAMENT MECHANISM filaments. Reluctance to accept this explana¬ OF CONTRACTION tion was dispelled by the subsequent discovery of two flexible regions in the myosin molecule, Histologists using the light microscope ob¬ one at the base of the heads and one at the served the changes in relative length of the junction of the heavy and the light meromysin sarcomeres during contraction but could offer subunits. The existence of these two flexible no satisfactory explanation of the mechanism regions permits the heads to attach over a of contraction. In the past three decades, de¬ range of interfilament distances and still main¬ tailed analysis of muscle by electron micros¬ tain the same orientation of the heads relative copy and X-ray diffraction has revealed the to the actin filaments (Fig. 10-25). What part structural basis for its periodic cross-striations of the myosin molecule develops the force that and has led to a widely accepted explanation results in filament movement is still not en¬ of the mechanism of myofibril shortening. tirely clear, but it is believed that a force-gen¬ Any acceptable theory had to account for erating change occurs in the angle of attach¬ the observation that, during contraction, the ment of the heads to the actin filaments. length of the A-band remains constant while For the conversion of chemical energy to the length of the I-band and H-band both mechanical work, actin filaments are a neces¬ decrease. An explanation became apparent sary cofactor in the release of energy by the when the electron microscope revealed two ATPase in the heads of the myosin molecules. sets of interdigitating filaments. In the sliding If purified actin is added to myosin filaments, filament theory, it is proposed that when muscle in vitro, there is rapid hydrolysis of ATP. contracts, the thick and thin filaments main¬ However, in resting muscle, the binding sites tain the same length as in resting muscle, but for myosin on the thin filaments are blocked MUSCULAR TISSUE 285 RESTING LENGTH STRETCHED Figure 10-28. Diagram of the changing appearance of the cross-striations of skeletal muscle in different phases of contraction (on left) and the corresponding differences in the degree of interdigitation of the thick and thin filaments (on right). by the tropomyosin—troponin complexes, pre¬ branches that penetrate into its interior via venting myosin-actin interaction. Release of the perimysial septa. Individual axons then calcium into the sarcoplasm, in response to a ramify in the endomysium to form endings, nerve impulse, is followed by binding of cal¬ from a few to several hundred, on individual cium to troponin C in each of the subunits muscle fibers. A single motor neuron, its axon, along the length of the actin filaments (Fig. 10— and the muscle fibers it innervates together 25). This is believed to cause a conformational constitute a motor unit. Activity of a motor unit change that moves the tropomyosin chains is all-or-none; that is, the fibers of the unit deeper into the groove of the actin helix, either contract fully in response to a nerve exposing the myosin-binding sites. Binding impulse or not at all. The largest motor units then activates the myosin ATPase and the re¬ may generate a tension 200 times that of the sulting release of energy induces a flexion of smallest motor units. In the graded response the myosin heads that displaces the thin fila¬ that is possible in whole muscles, the strength ments a short distance toward the center of the o