DNA and DNA Replication PDF
Document Details
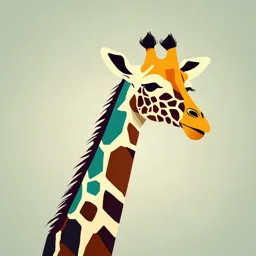
Uploaded by CleanerEllipse1228
İstanbul Okan University
Dr. Hilal Eren Gözel
Tags
Related
- Lecture 1 DNA Structure, Function, Metabolism and Replication v1 PDF
- DNA and the Molecular Structure of Chromosomes PDF
- Lecture 2.1 Molecular and Cellular Biology (DNA Replication) PDF
- FLS L1 DNA Structure and Replication 2024
- Human Genetics and Molecular Biology Notes PDF
- DNA Structure, Replication, Transcription, and Translation PDF
Summary
This document discusses DNA and DNA replication. It includes diagrams and explanations of the processes involved. It is likely a lecture or class presentation on DNA replication.
Full Transcript
DNA and DNA Replication Dr. Hilal Eren Gözel I. Okan University DNA is the genetic material Hereditary information is encoded in the chemical language of DNA and reproduced in all the cells of our body. It is the DNA program that directs the development of many different types of traits...
DNA and DNA Replication Dr. Hilal Eren Gözel I. Okan University DNA is the genetic material Hereditary information is encoded in the chemical language of DNA and reproduced in all the cells of our body. It is the DNA program that directs the development of many different types of traits. 5’ end O OH P Hydrogen bond –O 3’ end O OH O T A O O CH2 O P –O O O– O P O H2C O O Phosphodiester bond G C O O CH2 O P –O O O– O P O H2C O O C G O O CH2 O P O –O O– O P O H2C O O A T O CH2 OH 3’ end O O– P O O (b) Partial chemical structure Figure 16.7b 5’ end DNA Replication DNA replication in most eukaryotic cells occurs only during a specific part of the cell-division cycle, called the DNA synthesis phase or S phase. Since the two strands of DNA are complementary Each strand acts as a template for building a new strand in replication In DNA replication The parent molecule unwinds, and two new daughter strands are built based on base-pairing rules DNA Replication What is the purpose of this? First Second Parent cell replication replication Conservative model. The two parental strands reassociate after acting as templates for new strands, thus restoring the parental double helix. Semiconservative model. The two strands of the parental molecule DNA Replication separate, and each functions as a template for synthesis of a new, comple- mentary strand. Dispersive model. Each strand of both daughter mol- ecules contains a mixture of **Advanced Reading: old and newly synthesized Meselson–Stahl experiment DNA. Figure 16.10 a–c DNA Replication DNA replication is semiconservative Each of the two new daughter molecules will have one old strand, derived from the parent molecule, and one newly made strand Origins of Replication The replication of a DNA molecule begins at special sites called origins of replication, where the two strands are separated A eukaryotic chromosome may have hundreds or even thousands of replication origins However, bacterial genome is circular and has a single origin. Origins of Replication Initiator proteins that initiate DNA replication recognize this sequence and attach to the DNA, break the hydrogen bonds, separating the two strands and opening up a replication “bubble.” By binding and opening up the double helix, initiator proteins also attract a group of proteins that carry out DNA replication. These proteins form a replication machine, in which each protein carries out a specific function. Replication of DNA then proceeds in both directions until the entire molecule is copied. Origins of Replication Origins of Replication At each end of a replication bubble is a replication fork, a Y-shaped region where the parental strands of DNA are being unwound. At each fork, a replication machine moves along the DNA, opening up the two strands of the double helix and using each strand as a template to make a new daughter strand. Origins of Replication Origin of replication Parental (template) strand 0.25 µm Daughter (new) strand 1 Replication begins at specific sites where the two parental strands separate and form replication bubbles. Bubble Replication fork 2 The bubbles expand laterally, as DNA replication proceeds in both directions. 3 Eventually, the replication bubbles fuse, and synthesis of the daughter strands is complete. Two daughter DNA molecules (a) In eukaryotes, DNA replication begins at many sites along the giant (b) In this micrograph, three replication DNA molecule of each chromosome. bubbles are visible along the DNA of Figure 16.12 a, b a cultured Chinese hamster cell (TEM). Problem #1 How to unzip entire DNA double helix and prevent them re-binding? DNA Replication Two types of replication proteins—DNA helicases and single-strand DNA-binding proteins—cooperate to carry out this task. For DNA replication to occur, the double helix must be unzipped ahead of the replication fork so that the incoming nucleoside triphosphates can form base pairs with each template strand. Helicases are enzymes that unzip the double helix at the replication forks, separating the two parental strands and making them available as template strands. DNA Replication The helicase sits at the very front of the replication machine where it uses the energy of ATP hydrolysis to propel itself forward, prying apart the double helix as it speeds along the DNA. DNA Replication Single-strand DNA-binding proteins bind tightly and cooperatively to the single-stranded DNA exposed by the helicase without covering the bases, transiently preventing the strands from re-forming base pairs and keeping them in an elongated form so that they can serve as efficient templates. Replication Machinery An additional replication protein, called a sliding clamp, keeps DNA polymerase firmly attached to the template while it is synthesizing new strands of DNA. Left on their own, most DNA polymerase molecules will synthesize only a short string of nucleotides before falling off the DNA template strand. The sliding clamp forms a ring around the newly formed DNA double helix and, by tightly gripping the polymerase, allows the enzyme to move along the template strand without falling off as it synthesizes new DNA. Replication Machinery Problem #2 Unzipping a helical molecule creates a tension and may break it!!! DNA Replication After the parental strands separate, single-strand binding proteins (SSBP) bind to the unpaired DNA strands, keeping them from re-pairing. Topoisomerase helps relieve this strain by breaking, swiveling, and rejoining DNA strands. These enzymes produce transient nicks in the DNA backbone, which temporarily release the tension; they then reseal the nick before falling off the DNA. DNA Replication https://www.youtube.com/watch?v=EYGrElVyHnU - Video 1 Problem #3 DNA polymerase cannot bind to the template by itself! Initiation of Replication The enzymes that synthesize DNA cannot initiate the synthesis of a polynucleotide; they can only add nucleotides to the end of an already existing chain that is base-paired with the template strand. The initial nucleotide chain that is produced during DNA synthesis is actually a short stretch of RNA, not DNA. This RNA chain is called a primer and is synthesized by the enzyme primase. Initiation of Replication Primase starts a complementary RNA chain from a single RNA nucleotide, adding RNA nucleotides one at a time, using the parental DNA strand as a template. The completed primer, generally 5–10 nucleotides long, is thus base-paired to the template strand. The new DNA strand will start from the 3 end of the RNA primer. Elongating a New DNA Strand Elongation of new DNA at a replication fork is catalyzed by enzymes called DNA polymerases, which add nucleotides to the 3 end of a growing strand. (Heart of replication machine!) New strand Template strand 5’ end 3’ end 5’ end 3’ end Sugar A T A T Phosphate Base C G C G G C G C A T A P P OH C Pyrophosphate 3 end C OH 2 P Figure 16.13 5’ end 5’ end Elongating a New DNA Strand The polymerization reaction involves the formation of a phosphodiester bond between the 3ʹ end of the growing DNA chain and the 5ʹ-phosphate group of the incoming nucleotide, which enters the reaction as a deoxyribonucleoside triphosphate. The energy for polymerization is provided by the incoming deoxyribonucleoside triphosphate itself: hydrolysis of one of its high-energy phosphate bonds fuels the reaction that links the nucleotide monomer to the chain, releasing pyrophosphate. Elongating a New DNA Strand deoxyribonucleoside triphosphate Elongating a New DNA Strand For replication in Eukaryotes, up to 15 different DNA polymerases discovered so far. Eukaryotic DNA pols are designated with greek letters (DNA pols α, β, γ, δ, ε, ζ, η, θ, ι, κ, λ, μ, ν), with the exception of terminal transferase and Rev1. Bacterial DNA pols are designated with roman numerals (DNA pol I, II, III, IV, and V). Antiparallel Elongation In eukaryotes, DNA replication requires three DNA pols: DNA pol α initiates the DNA Replication, DNA pol ε is required for the leading strand, and DNA pol α and DNA pol δ is required for replication of the lagging strand. Rest of the polymerases have important roles in DNA repair. Problem #4 Antiparallel Elongation creates fragments on the lagging strand! How does the antiparallel structure of the double helix affect replication? https://www.youtube.com/watch?v=TNKWgcFPHqw Video 2 Antiparallel Elongation DNA polymerases add nucleotides only to the free 3end of a growing strand. Along one template strand of DNA, the leading strand DNA polymerase can synthesize a complementary strand continuously, moving toward the replication fork. Antiparallel Elongation Antiparallel Elongation – Video 2 Antiparallel Elongation To elongate the other new strand of DNA, the lagging strand, DNA polymerase must work in the direction away from the replication fork. The lagging strand is synthesized as a series of segments called Okazaki fragments, which are then joined together by DNA ligase! A summary video of DNA replication https://www.youtube.com/watch?v=Qqe4thU-os8 Problem #5 Starting point of the lagging strand cannot be filled! Replicating the Ends of DNA Molecules There is a small portion of the cell’s DNA that DNA polymerases can neither replicate nor repair. For linear DNA, such as the DNA of eukaryotic chromosomes, the fact that a DNA polymerase can add nucleotides only to the 3’ end of a preexisting polynucleotide leads to what might appear to be a problem. Replicating the Ends of DNA Molecules The usual replication machinery provides no way to complete the 5’ ends of daughter DNA strands. Even if an Okazaki fragment can be started with an RNA primer bound to the very end of the template strand, once that primer is removed, it cannot be replaced with DNA because there is no 3’ end available for nucleotide addition The ends of eukaryotic chromosomal DNA get shorter with each round of replication 5’ End of parental Leading strand DNA strands Lagging strand 3’ Last fragment Previous fragment RNA primer 5’ Lagging strand 3’ Primer removed but Removal of primers and cannot be replaced replacement with DNA with DNA because where a 3’ end is available no 3’ end available for DNA polymerase 5’ 3’ Second round of replication 5’ New leading strand 3’ New lagging strand 5’ 3’ Further rounds of replication Shorter and shorter Figure 16.18 daughter molecules Telomeres! Eukaryotic chromosomal DNA molecules have special nucleotide sequences called telomeres at their ends. Telomeres do not contain genes; instead, the DNA typically consists of multiple repetitions of one short nucleotide sequence. Telomeres! In each human telomere, for example, the six- nucleotide sequence TTAGGG is repeated between 100 and 1,000 times. Telomeric DNA acts as a kind of buffer zone that protects the organism’s genes. Telomeres provide their protective function by postponing the erosion of genes located near the ends of DNA molecules. Telomerase! An enzyme called telomerase catalyzes the lengthening of telomeres in eukaryotic germ cells, thus restoring their original length and compensating for the shortening that occurs during DNA replication. Telomerase is not active in most human somatic cells, but its activity in germ cells results in telomeres of maximum length in the zygote. Telomerase! Telomerease! Clinical Correlation: Colorectal Cancer Colorectal cancer (CRC) is the third most commonly diagnosed cancer, with over one million new cases every year. Although improved treatments, increased awareness, and early detection have contributed to prolonged survival, CRC still represents an important cause of cancer-related deaths. American Cancer Society Statistics, 2024 Clinical Correlation: Colorectal Cancer The activation of telomerase reverse transcriptase (TERT), the catalytic component of the telomerase complex, allows cancer cells to grow indefinitely by maintaining the length of the telomeres, thus favouring tumour formation/progression. The erosion of telomeres or increased telomerase activity, mainly because of cell proliferation, may be accelerated by specific alterations in the genes involved in CRC carcinogenesis, such as APC (tumor suppressor protein) and MSH2 (DNA repair protein). Clinical Correlation: Colorectal Cancer The prognostic role of telomere length in CRC is still under debate. Several studies indicate that TERT increases with disease progression, and most studies suggest that telomerase is a useful prognostic factor. Plasma TERT mRNA may also be a promising marker for the monitoring of disease progression and response to therapy. References & Thank You! Bertorelle R, Rampazzo E, Pucciarelli S, Nitti D, De Rossi A. Telomeres, telomerase and colorectal cancer. World J Gastroenterol. 2014 Feb 28;20(8):1940-50. doi: 10.3748/wjg.v20.i8.1940. PMID: 24616570; PMCID: PMC3934464. American Cancer Society. Cancer Facts & Figures 2024. Atlanta: American Cancer Society; 2024.