3 Trilaminar Germ Disc - Third Week of Development PDF
Document Details
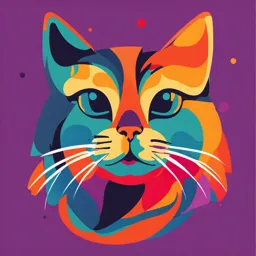
Uploaded by CleanlyNobility9545
Near East University
Gizem SÖYLER, PhD
Tags
Related
- Embryology (002) 1st Week of Development PDF
- Overview of Human Embryologic Development PDF
- BIU Human Embryology Lesson 05 Week 06 Development PDF
- BIU Human Embryology Lesson 04 Development Week 06 PDF
- GEMD-102 Introduction to Embryology and the 1st Week of Development PDF
- Histology Class Embryology - First Three Weeks of Development PDF
Summary
This document details the 3 trilaminar germ disc formation. The document includes an overview of the third week of embryo development, including the appearance of the primitive streak, development of the notochord, and differentiation of three germ layers. Crucial processes such as gastrulation are also mentioned.
Full Transcript
TRILAMINAR GERM DISK FORMATION Gizem SÖYLER, PhD Near East University Faculty of Medicine Department of Histology & Embryology Summary of the last lecture 3rd Week of Embryo Development At the end of the second week, the embryo consists of two flat layers of cells: the epiblast and the hypob...
TRILAMINAR GERM DISK FORMATION Gizem SÖYLER, PhD Near East University Faculty of Medicine Department of Histology & Embryology Summary of the last lecture 3rd Week of Embryo Development At the end of the second week, the embryo consists of two flat layers of cells: the epiblast and the hypoblast. As the third week of pregnancy begins, the embryo enters the period of gastrulation. Rapid development of the embryo from the trilaminar embryonic disc during the third week is characterized by: 1. Appearance of primitive streak 2. Development of notochord 3. Differentiation of three germ layers The third week of development coincides with the week following the first missed menstrual period, that is, 5 weeks after the first day of the last normal menstrual period. Cessation of menstruation is often the first indication that a woman may be pregnant. Gastrulation Gastrulation is a formative process by which the three germ layers, which are precursors of all embryonic tissues, and the axial orientation are established in embryos. During gastrulation, the bilaminar embryonic disc is converted into a trilaminar embryonic disc. Extensive cell shape changes, rearrangement, movement, and alterations in adhesive properties contribute to the process of gastrulation. Gastrulation is the beginning of morphogenesis (development of body form) and is the most significant event occurring during the third week. During this week, the embryo is referred to as a gastrula. Each of the three germ layers (ectoderm, mesoderm, and endoderm) gives rise to specific tissues and organs: 1. Embryonic ectoderm gives rise to the epidermis, central and peripheral nervous systems, eyes and internal ears, neural crest cells, and many connective tissues of the head. 2. Embryonic endoderm is the source of the epithelial linings of the respiratory and digestive tracts, including the glands opening into the gastrointestinal tract, and glandular cells of associated organs such as the liver and pancreas. 3. Embryonic mesoderm gives rise to all skeletal muscles, blood cells, the lining of blood vessels, all visceral smooth muscular coats, serosal linings of all body cavities, ducts and organs of the reproductive and excretory systems, and most of the cardiovascular system. Primitive Streak The primitive streak is the first morphological sign of gastrulation and appears on the surface of the epiblast in the bilaminar embryonic disc during the third week. This thickened linear band of epiblast forms caudally in the median plane of the embryonic disc's dorsal aspect. It is crucial for establishing the embryo's craniocaudal axis, cranial and caudal ends, dorsal and ventral surfaces, and right and left sides. The streak elongates as cells are added to its caudal end, and its cranial end forms the primitive node, while a groove called the primitive groove develops along with a small depression, the primitive pit. As more and more cells move between the epiblast and hypoblast layers, they begin to form mesenchyme, an embryonic connective tissue that gives rise to intraembryonic mesoderm and contributes to the formation of various tissues and organs. As the primitive streak functions, it produces extraembryonic mesoderm covering the yolk sac and amnion. After that, it diminishes in size and becomes insignificant, eventually undergoing degenerative changes and disappearing by the end of the fourth week. Through this process, the epiblast generates all three germ layers— ectoderm, mesoderm, and endoderm—which are the foundations for all tissues and organs in the embryo. Notochord Formation The notochord is a critical structure in early embryonic development, playing a key role as a signaling center for the formation of various structures, including the central nervous system (CNS) and axial skeleton. Mesenchymal cells migrate through the primitive streak, acquiring mesodermal cell fates. These cells migrate cranially from the primitive node and pit, forming a median cellular cord called the notochordal process. The notochordal process develops a lumen, becoming the notochordal canal. The notochordal process continues to grow cranially between the ectoderm and endoderm until it reaches the prechordal plate (an area where the ectoderm and endoderm are fused). The prechordal plate is crucial for signaling the development of cranial structures, including the forebrain and eyes. Additional mesenchymal cells from the primitive streak and notochordal process migrate laterally and cranially between the ectoderm and endoderm, contributing to the formation of the cardiogenic mesoderm (important for heart development). Instructive signals from the primitive streak induce notochordal precursor cells to form the notochord, a rod-like structure. The notochordal process elongates by invagination of cells from the primitive pit. An indentation forms, which extends into the notochordal process, further developing the notochordal canal. The floor of the notochordal process fuses with the underlying embryonic endoderm. This fusion leads to the degeneration of the fused layers, forming openings that connect the notochordal canal with the umbilical vesicle. As the openings in the floor of the notochordal process become confluent, the floor disappears, and the remaining structure forms the notochordal plate. The cells of the notochordal plate proliferate and undergo infolding, creating the definitive notochord. he proximal part of the notochordal canal temporarily persists as the neurenteric canal, forming a communication between the amniotic cavity and the umbilical vesicle. This canal is eventually obliterated as development progresses. The notochord detaches from the endoderm of the umbilical vesicle, which then becomes a continuous layer. The notochord extends from the oropharyngeal membrane to the primitive node, eventually contributing to the formation of the nucleus pulposus of each intervertebral disc as it degenerates. The notochord functions as a primary signaling center, inducing the overlying embryonic ectoderm to thicken and form the neural plate, the primordium of the central nervous system (CNS). Functions of the Notochord Axis Formation: The notochord defines the primordial longitudinal axis of the embryo, providing rigidity and serving as the initial scaffold for body structure. Signaling Center: It acts as a primary signaling center, inducing the overlying embryonic ectoderm to thicken and form the neural plate, the primordium of the CNS. Induction of Structures: The notochord sends signals necessary for the development of axial musculoskeletal structures and the CNS, and contributes to the formation of intervertebral discs. Allantois Around day 16 of embryonic development, the allantois appears as a small outpouching from the caudal wall of the umbilical vesicle. This structure extends into the connecting stalk. The allantoic mesoderm expands beneath the chorion and forms blood vessels that eventually supply the placenta. The proximal part of the allantoic diverticulum persists as a stalk called the urachus, which extends from the bladder to the umbilical region during much of development. In adults, the urachus is represented by the median umbilical ligament. The blood vessels of the allantoic stalk develop into the umbilical arteries. The intraembryonic part of the umbilical veins has a separate origin from the allantois. Neurulation The processes involved in the formation of the neural plate and neural folds and closure of the folds to form the neural tube constitute neurulation. Neurulation is completed by the end of the fourth week, when closure of the caudal neuropore occurs. The notochord induces the overlying embryonic ectoderm to thicken, forming the neural plate. This plate consists of thickened epithelial cells called neuroectoderm, which gives rise to the CNS, including the brain, spinal cord, and other structures like the retina. The neural plate initially matches the length of the notochord but eventually extends cranially to the oropharyngeal membrane, surpassing the notochord's length. Around the 18th day, the neural plate begins to invaginate along its central axis, creating the neural groove flanked by neural folds. The neural folds, especially prominent at the cranial end of the embryo, are the first indicators of brain development. By the end of the third week, the neural folds begin to fuse, transforming the neural plate into the neural tube, the primordium of the brain vesicles and spinal cord. The neural tube then detaches from the surface ectoderm as the neural folds meet. As the neural folds fuse, neural crest cells undergo an epithelial-to-mesenchymal transition and migrate away. The surface ectoderm fuses over the neural tube, later differentiating into the epidermis. Neurulation, the neural tube forming process, is completed during the fourth week. As the neural folds fuse to form the neural tube, some neuroectodermal cells along the inner margin of each fold lose their epithelial characteristics and detach from neighboring cells. These cells form a flattened, irregular mass called the neural crest, located between the neural tube and the overlying surface ectoderm. The neural crest soon separates into right and left parts, shifting to the dorsolateral aspects of the neural tube. Here, neural crest cells give rise to the sensory ganglia of spinal and cranial nerves. These cells then migrate both into and over the surface of somites, following predefined pathways, as revealed by special tracer techniques. Derivatives of Neural Crest Cells: Spinal ganglia (dorsal root ganglia) Autonomic nervous system ganglia Ganglia of cranial nerves V, VII, IX, and X Neurolemma sheaths of peripheral nerves Leptomeninges(arachnoid mater and pia mater) Pigment cells Suprarenal medulla (part of the adrenal gland) Various tissues and organs throughout the body Neural Tube Defects Neural tube defects (NTDs) are serious congenital anomalies that occur when neurulation, the process by which the neural tube forms, fails to occur properly during the third and fourth weeks of gestation. These defects can be open (exposed to the surface) or covered with skin, with open NTDs being more severe. Types of Neural Tube Defects (NTDs) 1. Open NTDs: Craniorachischisis: Total dysraphism where the entire neural tube is open along the head and back. Cranioschisis (Anencephaly): The brain fails to develop properly, resulting in the absence of a functional forebrain. Most infants with anencephaly do not survive long after birth. Myeloschisis (Spina Bifida Aperta): Localized dysraphism, typically at the lumbosacral level, where the spinal cord is open to the surface. Variants include: Meningocele: Only the membranes protrude from the vertebral canal. Meningomyelocele/Myelomeningocele: The spinal cord and membranes protrude, often associated with severe neurological deficits. 2. Skin-Covered NTDs: Encephaloceles: Brain tissue protrudes through the skull, which is covered by skin, potentially leading to severe neurological impairment. Spina Bifida Occulta: A hidden defect where the spinal cord is covered by skin, often marked externally by signs like a tuft of hair, mole, or dimple. It affects about 2% of the population. Prevalence and Detection NTDs occur in approximately 0.1% of live births, with about 4,000 pregnancies affected annually in the U.S. Globally, 500,000 infants are born with spina bifida aperta yearly. Early detection is possible through maternal serum alpha-fetoprotein (MSAFP) screening which is confirmed with ultrasound or amniocentesis. Elevated alpha-fetoprotein levels in maternal serum are associated with NTDs, while lower levels suggest conditions like Down syndrome. Development of Somites Somites are segmented blocks of mesodermal tissue that form along the sides of the neural tube during embryogenesis. Somites develop from the paraxial mesoderm, a thick longitudinal column of cells derived from the primitive node. The paraxial mesoderm is continuous laterally with the intermediate mesoderm, which in turn thins out into the lateral mesoderm. Toward the end of the third week, the paraxial mesoderm begins to differentiate, condense, and divide into paired cuboidal bodies called somites. Somites form in a craniocaudal sequence, meaning they develop from the head region (occipital) downward along the body. During the somite period (days 20 to 30), about 38 pairs of somites form. By the end of the fifth week, there are 42 to 44 pairs of somites. The number and appearance of somites are used as criteria to determine the age of the embryo. Functions of Somites: Most of the axial skeleton (bones of the head and trunk) Associated musculature The adjacent dermis of the skin Motor axons from the spinal cord innervate the muscle cells in the somites, guided by specific pathways. Development of Intraembryonic Coelom The intraembryonic coelom begins as isolated coelomic spaces in the lateral intraembryonic mesoderm and cardiogenic mesoderm. These spaces merge to form a single horseshoe-shaped cavity, the intraembryonic coelom. The intraembryonic coelom divides the lateral mesoderm into two layers: 1. Somatic (Parietal) Layer: Located beneath the ectoderm, continuous with the extraembryonic mesoderm covering the amnion. 2. Splanchnic (Visceral) Layer: Located adjacent to the endoderm, continuous with the extraembryonic mesoderm covering the umbilical vesicle. The somatic mesoderm and the overlying ectoderm form the embryonic body wall (somatopleure). The splanchnic mesoderm and the underlying endoderm form the embryonic gut (splanchnopleure). During the second month, the intraembryonic coelom divides into three major body cavities: 1. Pericardial Cavity 2. Pleural Cavities 3. Peritoneal Cavity Early Development of Cardiovascular System Initially, the embryo obtains nutrition from maternal blood through diffusion via the extraembryonic coelom and umbilical vesicle. Blood vessels begin to form in the extraembryonic mesoderm of the umbilical vesicle, connecting stalk, and chorion. Embryonic blood vessels start developing approximately two days later, driven by the need to deliver oxygen and nutrients from the maternal circulation via the placenta. Development of the Vascular System: Vasculogenesis: Formation of new vascular channels by the assembly of angioblasts (endothelial cell precursors). Angiogenesis: Formation of new blood vessels by budding and branching from existing vessels. Blood Islands: Angioblasts aggregate to form these clusters, where they differentiate into endothelial cells and form the endothelium, which eventually fuses to create networks of vascular channels. Mesenchymal cells around the endothelial vessels differentiate into muscular and connective tissue components of the blood vessels. Blood cells first develop from specialized endothelial cells (hemangiogenic epithelium) associated with the umbilical vesicle and allantois at the end of the third week. Hematopoiesis, the formation of blood, begins in the fifth week, initially in areas along the aorta and later in the liver, spleen, bone marrow, and lymph nodes. Fetal and adult erythrocytes are derived from hematopoietic progenitor cells. The heart and great vessels develop from mesenchymal cells in the cardiogenic area. Paired endocardial heart tubes form and fuse to create the primordial heart tube. This heart tube connects with blood vessels in the embryo, forming a primitive cardiovascular system. By the end of the third week, blood circulation begins, and the heart starts beating on the 21st or 22nd day. The cardiovascular system is the first organ system to become functional, with the heartbeat detectable by Doppler ultrasonography during the fourth week (about six weeks after the last menstrual period). Development of Chorionic Villi Primary chorionic villi appear at the end of the second week and begin to branch. Early in the third week, mesenchyme grows into these villi, forming secondary chorionic villi, which cover the entire surface of the chorionic sac. Some mesenchymal cells in the villi differentiate into capillaries and blood cells, leading to the formation of tertiary chorionic villi with visible blood vessels. Capillaries in the villi fuse to form arteriocapillary networks, connecting with the embryonic heart through vessels in the chorion and connecting stalk. By the end of the third week, embryonic blood flows through the villi's capillaries, allowing for the exchange of oxygen, nutrients, carbon dioxide, and waste products between maternal and embryonic blood. Cytotrophoblastic cells in the villi proliferate, forming an extravillous cytotrophoblastic shell that surrounds the chorionic sac and attaches it to the endometrium. Types of Villi: Stem Villi (Anchoring Villi): Attach to maternal tissues through the cytotrophoblastic shell. Branch Villi: Grow from the sides of stem villi and facilitate the main exchange of materials between maternal and embryonic blood.