Crack Notes - Lipid Metabolism PDF
Document Details
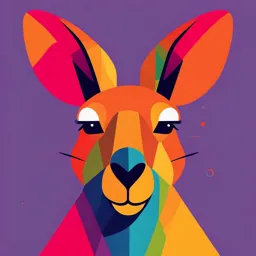
Uploaded by NoteworthyAwe
UMCH
Tags
Summary
This document provides detailed notes on lipid metabolism, covering various pathways including digestion, absorption, synthesis, and breakdown of lipids. It explains the Krebs cycle, electron transport chain, and different processes like beta-oxidation and de novo fatty acid synthesis. The notes include diagrams and key enzymes involved in lipid processing.
Full Transcript
KREBS CYCLE T succinate thiokinase ELECTRON TRANSPORT CHAIN DIGESTION/ABS. : EXOGENOUS PATHWAY (TRIGLYCERIDES, SHORT CHAINS) 1. Food enters mouth where it is broken down via Saliva, TRIGLYCERIDE: which contains an enzyme called saliva lipase....
KREBS CYCLE T succinate thiokinase ELECTRON TRANSPORT CHAIN DIGESTION/ABS. : EXOGENOUS PATHWAY (TRIGLYCERIDES, SHORT CHAINS) 1. Food enters mouth where it is broken down via Saliva, TRIGLYCERIDE: which contains an enzyme called saliva lipase. position 1 (From milk for example) position 2 Saliva lipase: - Ph resistant (optimal ph 2.5-5) position 3 2. Food travels to intestines and are emulsified by bile salts (produced by bladder). ' Omni q - - - c - ont t FA FA d Orrin 3. Pancreatic Lipase (produced by pancreas) hydrolyzes (breaks) bonds in → - - triglycerides in position 1 and 3 forming 2-monoglyceride + 2FA molecules. This can be absorbed into the blood = 2MG 4.( IN CASES OF NECESSARY COMPLETE ONLY DIGESTION (otherwise skip): An Isomerase moves the monoglycerides position 2 bond to position 1, where lipase hydrolyses it to FA and Glycerol. ) 5. Broken down triglyceride (2-monoglyceride or glycerol) is absorbed through the intestinal mucosa, moving into the enterocytes (intestinal wall) where they are reassembled to triglycerides covered in proteins called apolipoproteins as they can't travel through blood in its simple form, called chylomicrons. 6. Chylomicrons travel through lymphatic system into the blood stream, traveling to tissues that have receptors on the walls that recognize the proteins of the chylomicrons. D-Chylomicrons - VLDL 7. Lipoprotein Lipase is found in the junction between capillaries and tissue wall where the chylomicrons travel, which is activated by a protein called apoC-II (found on chylomicron), resulting the chlylomicron to split into fatty acids and glycerol to be able to enter the tissues. -Dincytosol 8. Fatty acids and glycerol travel into the tissues where they are oxidized to produce energy in muscle tissue or stored in adipose tissue. -> If the muscle cells have enough energy then the fatty acids will travel to adipose tissue and if not to the muscles. diatery tryglycerides muscles B adipose tissue cholesterol diatery - < liver 9. Chylomicrons shrink into chylomicron remnants with only a little bit of cholesterol inside, which enter the blood stream and are taken in by the liver to produce hormones, vitamins and bile salt from the cholesterol. ENDOGENOUS PATHWAY (LIPIDS THAT ARE SYNTHESISED IN THE BODY) ->Excess of glucose intake through food that can not be deposited as glycogen will form lipid stores in the liver. 1. VLDL fractions (covered in proteins) are formed, which collect lipids from the liver. 2. VLDL travels via the bloodstream, through the capillaries, to the tissues (adipose tissue or muscle tissue). 3. Lipoprotein Lipase in Junctions between capillaries and tissue walls is activated by the protein apoC-11 on VLDL surface, which releases triglycerides into the tissues out of the VLDL. 4. VLDL shrinks forming IDL., which turns to LDL (containing mostly cholesterol) as the density increases, removing all proteins from the surface accept for ApoB-11. oo 5. LDL travels to Extrahepatic tissues releasing cholesterol inside due to receptors specific to the ApoB-11 protein on oo LDL. 6. LDL fraction goes inside the cell, where it fuses with a lysosome, which contain enzymes that hydrolize the cholesterol esters to simple cholesterol. (Endoctosis) NEGATIVE EFFECT: Cholesterol is needed in membranes more. POSITIVE EFFECT: Cholesterol is a precurser for any steroidic hormones. (Converted into hormones eg. in ovaries, sex hormones) FORMATION OF TRIGLYCERIDE: 1. PRODUCTION OF ACYL-CoA ↓ thioester linkage Fatty acid is activated by CoASH (from pantothenic acid), which requires ATP = Acyl-CoA (IN OUTER MITOCHONDRIAL MEMBRANE IN CYTOPLASM) Actual structure : ↳ (vitamin) 2. PRODUCTION OF GLYCEROL-3-PHOSPHATE 1. In Liver: Lipids (glycerol) that are deposited in adipose tissue, transfer to the liver where they are activated by Glycerol Kinase (only active in the liver), turning into Glycerol.-3-phosphate. (Glycerol thats present in the liver can also be converted.) 2.: In Adipose tissue: Glucose generates DHAP via glycolysis, which can be transformed into Glycerol 3-phosphate. 3. COMBINING GLYCEROL-3-PHOSPHATE & ACYL-CoA Acyl-CoA are attached to each OH of Glycerol-3-Phosphate one by one. (Attaching to position one first gives Lysophosphatidic acid, then attaching to position 2 gives phosphatidic action and finally the third one is attached once a phosphate residue is removed to form triglyceride. BETA OXIDATION OF FATTY ACIDS: (step 7-10) 1. Triacylglycerol lipase is activated through phosphorylation after hormones (eg. glucagon or epinephrine) attach to receptors on the outer membrane of adipose tissue, which act to generate cAMP with the enyzme adenylylcyclase located in the membrane. 2. This causes a chain activation of protein kinase A or AMPkinase, which activates triglycerol lipase through phosphorylation, generating glycerol and fatty acids. 3.Fatty acids are activated in the outer mitochondrial membrane, catalyzed by acyl CoA-Synthetase. Will generate Acyl CoA. 4. Carnitine palmitoyl transferase 1 (enzyme) attaches carnitine (transporter for fatty acids) to acyl CoA in the outer mitochondrial membrane and travel inside the mitochondria. (long fatty acid chain can't get through the mitochondrial membrane) 5. Carnitine palmitoyl transferase 2 removes the acyl residue from the carnitine inside of the mitochondrial membrane. 6. Acyl-CoA travels to the krebs cycle. 7. Acyl-CoA is oxidized by Acyl.-CoA-dehydrogenase (enzyme) (FAD form) removing 2 carbons at a time and 2 Hydrogen protons, forming FADH2 and a geometrical isomer of acyl CoA with a trans double bond. 8. Water hydrates the isomer creating hydroxyacyl-CoA. 9. hydroxyacyl-CoA dehydrogenase (with NAD as Coenzyme) oxidizes hydroxyacyl-CoA by removing 2 hydrogen protons, creating NADH and ketoacyl-CoA. 10 CoA splits an acetyl-CoA molecule off of ketoacyl-CoA, SUMMARY : remaining with a fatty acid. 11. NADH and FADH travel to the electron transfer chain (located in the inner mitochondrial membrane), producing ATP by an oxidative phosphorylation process. 12. Cycle repeats DE NOVO BIOSYNTHESIS ->FATTY ACID SYNTHESIS (step 5-6) Excess of glucose, activated by insulin starts the process by activating acetyl-CoA Carboxylase (Step 5) 1. Acetyl CoA is produced in the mitochondria Acetyl CoA Source 1= comes from oxidative decarboxylation (pyruvate (produced by glycolysis) -> Acetyl CoA catalyzed by pyruvate dehydrogenase with TPP, lypoic acid, FAD-, NAD+, CoA as components) Acetyl CoA Source 2= Beta oxidation of fatty acids 2. Acetyl CoA combines with oxaloacetate, generating citrate synthase. 3. Citrate synthase travels through the mitochondrial membrane to the cytoplasm where ATP- citrate lyase (enzyme) splits Citrate into Acetyl-CoA and oxaloacetate, with the use of ATP. 4. Oxaloacetate can not travel back into the mitochondria, therefore it is reduced by malic dehydrogenase (enzyme) with the use of NADH to malate. Malate is then transformed into pyruvate by malic enzyme, generating NADPH + H+ and then traveling into the mitochondria where it is transformed back into Oxaloacetate by carboxylation via pyruvate carboxylase. (THIS PROCESS GENERATES NECESSARY NADPH FOR DE NOVO SYNTHESIS) 5. Carbon from CO2 is added to Acetyl-CoA catalyzed by acetyl-CoA Carboxylase (activated by Insulin) + ATP, forming Malonyl-CoA. (Rate limiting step) 6. Acetyl-CoA and Malonyl-CoA attach to the SH components of the multi enzyme complex Fatty acid Synthetase (6 enzymes + 1 protein (Acyl carrier protein (ACP) + 2 active thiol groups (SH groups, one originating from cysteine residue, the other from phosphopanthetine)), traveling from one enzyme to another: (see below) 1. Acetyl-CoA attaches to a SH group (Cysteine). 2. Malonyl- CoA attaches to the second (phosphopanthetine) SH group. 3. Condensation between Acetyl-CoA and Malonyl-CoA combining the two, forming a double acetate chain, Beta ketobutyryl-ACP and eliminating CO2. Residue is only attached to the phosphopanthetine SH group. 4. Keto group of Beta ketobutyryl-ACP is reduced to an alcohol forming Beta-Hydroxylbutyryl-ACP with NADPH. 5. Beta-Hydroxylbutyryl-ACP is dehydrated by removing Water, generating a double bond (unsaturated fatty acid), Trans-delta2-Butenoyl-ACP. 6. The double bond is reduced with NADPH, forming a saturated fatty acid Butyryl-ACP. (4 carbons) 7. Butyryl-ACP is transferred from the phosphopanthetine SH group to the cysteine SH group. 8. Another Malonyl-CoA attaches to the phosphopanthetine SH group. 9. Malonyl-CoA and Butyryl-ACP combine, repeating the cycle to make the fatty acid longer (up to 16 carbon atoms). AFTER 16 C= ELONGATION. Elongases and desaturases catalyze the addition of more carbon atoms in the form of malonyl-CoA without the multi enzyme complex. (polyunsaturated synthesis: requires precursor from food) REGULATION OF FATTY ACID SYNTHESIS: ALLOSTERIC REGULATORS: -> Bind to enzyme (not in active site) to inhibit or activate Enzyme. 1. Palmitoyl-CoA tries to inhibit the activity of acetyl-CoA carboxylase when the fatty acid chain becomes too long. (Inhibits polymerization) 2. The accumulation of Citrate in the cytoplasm, transported out of the mitochondria, (splits into acetyl-CoA and Oxaloacetate, catalyzed by citrate lyase) activates acetyl-CoA carboxylase. (Activates Polymerization.) COVALENT REGULATORS: -> by phosphorylation-dephosphorylation. (Hormones act on Acetyl-CoA-Carboxylase (tetrameric enzyme) by covalently modifying it. ) ACTIVATING HORMONES 1. After a meal, rich in carboydrates, insulin is released from beta cells of the pancreas. 2. The increased secretion of insulin will activate the enzyme Phosphodiesterase in the liver, which converts cAMP into the inactive form AMP. (The activity of the enzyme decreases due to the low cAMP concentration, mainting the enzyme in the active form.) INHIBITING HORMONES 1. increased secretion of glucagon (starvation) or epinephrine (muscle effort), attach to receptors, activating the enzyme adenylat cyclase, increasing cAMP production. 2.cAMP activate protein kinase (AMP kinase), stimulating phosphorylation of Acetyl-CoA Carboxylase, making the enzyme inactive. (inhibiting the snythesis of fatty acids.) KETONE BODIES -> Produced with absence of glucose by the liver (ENERGY SUPPLY) Ratio of Insulin/Glucagon changes in the favor of Glucagon (Will encourage beta oxidation and lipolysis). Lack of glucose in Tissue: Starvation or diabetes 3 GROUPS: 1. Acetone 2. Acetoacetate. 3. D-beta-hydroxybutyrate When there is way more acetyl-CoA than Oxaloacetate in the liver, the Acetyl-CoA will form ketone bodies (in the mitochondria of the liver) and the krebs cycle slows down. SYNTHESIS: 1. Three molecules of Acetyl-CoA combine to form B-Hydroxy-B-methylglutaryl-CoA (HMG-CoA) 2. One molecule of acetyl-CoA is removed from HMG-CoA forming Acetoacetate. 3. Via decarboxylation Acetone is obtained or via reduction D-beta-hydroxybutyrate is obtained. 4. Ketone bodies travel through our blood stream to tissues, enter the mitochondria and act as energy supply. 5. (KETOLYSIS begins).D-beta-hydroxybutyrate is oxidised to Acetoacetate catalyzed by DB hydroxybutyrate-dehydrogenase. 6. Carboxyl group of Acetoacetate is activated by coupling the metabolism of the ketone body with the krebs cycle (As GDP is produced in the krebs cycle, which is used to activate the carboxyl group.), forming Acetoacetyl-CoA. 7. Acetoacetyl-CoA splits due to a thiolase and a molecule of CoA, producing Acetyl-CoA. 8. Acetyl-CoA travels to the Krebs cycle to produce reduced Coenzymes which will be used to produce energy in the electron transfer chain. NEGATIVES OF KETONE BODIES: AMOUNT OF ENERGY PRODUCED FROM KETOLYSIS: KETOACIDOSIS: Acetone can be exhaled however acetoacetate and D-beta- hydroxybutyrate will be eliminated through urine in the form of salts. (The bodies alkaline buffering reserves are highly impaired by this excess, resulting in acidosis as the blood ph drops) CHOLESTEROL SYNTHESIS: In the liver (cytoplasm) 1. 2 molecules of Acetyl-CoA are condensed catalyzed by thiolase, forming acetoacetyl-CoA. 2. Another acetyl-CoA molecule is condensed, catalyzed by HMG- CoA synthase, forming HMG-CoA. 3. Enzyme, HMG-CoA reductase removes CoA from HMG-CoA, which is then reduced by 2 NADPH, forming Mevalonate. 4. Phosphate residue from ATP is added to Mevalonate, forming Phosphanevalonate. 5. Another phosphate residue from ATP is added to Phosphanevalonate, forming 5-Pyrophosphomevalonate 6. A carboxylic group is eliminated from 5-Pyrophosphomevalonate with the energy of ATP, forming two activated isoprene units called dimethylallyl pyrophsphate & delta3-isopentenyl pyrophosphate. 7. The two Isoprene units are condensed, releasing one phosphate group and forming Geranyl Pyrophosphate (C10). (Head to tail condensation) 8. Another molecule of an activated isoprene unit is combined (condensed) with the Geranyl Pyrophosphate, forming Farnesyl Pyrophosphate (C15). (Head to tail condensation) 9. 2 activated Farnesyl Pyrophosphate units condense, removing 2 pycrophosphate groups, forming Squalene. (C30). (Head to head condensation) 10. Oxygen and Hydrogen from NADPH binds to Squalene in position 3, introduced by squalene monoyxgenase. (sterol =a compound with hydroxyl group in position 3), forming a cyclic compound lanosterol. 11. Lanosterol goes through 20 reactions where 3 carbon atoms are removed, forming cholesterol. (27 C) PROTEIN DIGESTION {T.mgSUMMING Proteins 1. The presence of Proteins (from food) in the stomach Gastrin stimulates the gastric mucosa to secrete the hormone HCL (parietal cells) & Pepsinogen (chief cells) t { End Pepsin + low PH un Gastrin. t Peptide mixture 2. Gastrin stimulates the secretion of HCL by the : Secretin parietal cells (which leads to the low ph) and Pepsinogen Bicarbonate (buffer) t Cholecystokinin (Zymogen) by the chief cells of the gastric glands. Free amino acids Sodium Potassium transporter 3. Pepsinogen is converted into Pepsin (active form) due to the low PH. 4. Pepsin starts to break peptide bonds, forming a mixture of smaller peptides. (prefers proteins where amino-terminal side has an aromatic amino acid residue: Phe, Trp or Tyr.) 5. As the food passes from the stomach to the small intestines, the acid ph triggers the secretion of secretin. 6. Secretin stimulates the secretion of bicarbonate from the pancreas to buffer the acid, increasing the ph to 7. 7. Amino acids in the upper part of the intestine stimualte the secretion of the hormone Cholecystokinin, which stimulates more Zymogens to be transformed into active enzymes including Trypsinogen, Chymotrypsinogen and Procarboxypeptidases 8. A mixture of free amino acids results, which will be absorbed, entering the blood stream and travel to the liver. ABSORPTION OF AMINO ACIDS: 9. Amino acid transporters (specialized for specific amino acids), located in the intestinal cells, transport sodium into the cell due to a concentration gradient, letting the amino acid also enter the intestinal cell from the lumen. 10. Sodium is exchanged for potassium with the energy of ATP letting the amino acid be transported into the blood stream. THE UREA CYCLE: 1 Urea Synthesis requires = 4 ATP Molecules 1. In the mitochondrial matrix of the liver cells, Caramoyl Phosphate is formed from Bicarbonate and ammonia bonding (2 ATP molecules are used). Catalyzed by Cabamoyl phosphate snythetase I -> 2 forms: 1 = In mitochondria using NH3 to synthesise urea. 2= uses Glutamine to synthesise pyrimidine compounds. 2. Carbamoyl phosphate attaches to an amino acid (Ornithine), removing phosphate residue. 3. Radical from carbamoyl phosphate attaches to Ornithinie to form the amino acid Citrulline (In mitochondria.) 4. Citrulline goes into the cytoplasm where it bonds with aspartate to form Arginosuccinate catalyzed by argininosuccinate synthetase and 2 ATP molecules. 5. Carbon skeleton is spllit off Arginosuccinate due to the enzyme Lyase, forming Arginine and Fumarate. 6. Arginine goes through hydrolysis with the enzyme Arginase, forming Ornithine and Urea. 7. Urea is released into the blood stream and is removed Krebs cycle and Urea cycle run parallel: via the kidneys through urine. UREA CYCLE DISORDERS: (Ammonia is toxic because it may activate the sodium chloride cotransporter in the brain, which disrupts the osmotic pressure in nerve cells.) - General Defects (genetic) = lead to hyperammonemia (Ammonia can't be removed, so it goes in the brain causing hepatic encephalopathy). - Argininosuccinase deficiency = Can be partially bypassed by eating extra arginine and reducing protein intake - Carbamoyl Snythetase/Ornithine transcarbamoylase deficiency = Citrulline and argininosuccinate can not be used to remove nitrogen. (Accumulation of glycine and glutamine) -> can be removed by benzoate/phenylacetate via diet, condensing the excess. METABOLISM OF BRANCHED CHAIN AMINO ACIDS: Takes place in the muscle -> getting rid of ammonia 1. Amino acids are trapped by the muscles. 2. Muscles lack urea cycle enzymes, therefore Nitrogen is removed and transported to the liver. Nitrogen transport type 1: Glutamine. 1. Glutamate attaches to ammonia with energy from ATP in the muscles, forming Glutamine. 2. Glutamine is transported to the liver through the blood. (Non toxic form of transport) Nitrogen transport type 2: Glucose-alanine cycle 1. Glycogen breaks down into glucose, which goes through glycolysis to form pyruvate. 2. Amino group is removed from amino acid and the carbon skeleton is oxidised to produce reducced coenzymes (go to the electron transfer chain, producing ATP through phosphorylation.) 3. Amino group is transferred to pyruvate, generating alanine. 4. Alanine travels through blood to the liver, where it is converted back into pyruvate. (Reversed Transamination. Alpha- Keto-Glutarate takes the amino group of Alanine to convert into Glutamate) 5. Glutamate releases the amino group in the liver, forming Urea. 6. Pyruvate goes through gluconeogenesis to form glucose. DISEASES AND DISORDERS: METABOLISM - Jaundice (Impaired liver function or blocked bile secretion causes bilirubin to leak from the liver into the blood, resulting in a yellowing of the skin and eyeballs) EXCESS BILIRUBIN Treatment = Exposure to a fluorescent lamp, causes a photochemical conversion of bilirubin to compounds that are more soluble and easily excreted. - Congenital erythropoietic porphyria (Defect in synthesis of heme) ACCUMULATION OF UROPORPHYRINOGEN I - Acute intermittent Porphyria (Defect in synthesis of heme) overproduction of porphobilinogen and b-aminolevulinate - Phenylketonuria: Severe mental retardation (Phenylalanine can not be hydroxylated (high levels maintain, leading to the formation of phenylpyruvate)) EXCESS PHENYLALANINE - Maple syrup urine disease: (Decarboxylarion of Alpha-ketoacids from valine, isoleucine and leucine is blocked, eliminating the ketones in urine making the urine smell like maple syrup.) EXCESS ALPHA-KETOACIDS Hyperammonemia= UREA CYCLE General Defects (genetic) (Ammonia can't be removed, so it goes in the brain causing hepatic encephalopathy). - Argininosuccinase deficiency = Can be partially bypassed by eating extra arginine and reducing protein intake - Carbamoyl Snythetase/Ornithine transcarbamoylase deficiency = Citrulline and argininosuccinate can not be used to remove nitrogen. (Accumulation of glycine and glutamine) -> can be removed by benzoate/phenylacetate via diet, condensing the excess. Heart Disease: Excess of Choline due to TMAO production (Bacteria from the gut convert excess choline into trimethylamine (TMA), which is then transformed into trimethylamine-N-Oxide (TMAO) in the liver, which stimulates cholesterol uptake by macrophages = atherosclerosis). ACUTE PANCREATITIS: Obstruction in the normal pathway of the pancreatic secretion that leads to the intestines. (Zymogens are activated in the pancreas rather than where the food molecules are, causing attack of pancreatic tissue.) HARTNUP DISEASE: Defect in the transporter of Tryptophan and other nonpolar amino acids in the intestine. KETOACIDOSIS: (Acetone can be exhaled however acetoacetate and D-beta-hydroxybutyrate will be eliminated through urine in the form of salts. (The bodies alkaline buffering reserves are highly impaired by this excess, resulting in acidosis as the blood ph drops)) EXCESS ACETOACETATE & D- BETA-HYDROXYBUTYRATE Carnitine deficiency: Muscle weakness, heart & liver problems (Can't use fatty acids for energy supply as beta oxidation is reduced.) NOT ENOUGH CARNITINE HYPERCHOLESTEROLEMIA -> Absence of LDL receptors (Cholesterol is no longer uptaken in the tissues and remains in the blood stream, starting the process of Atherosclerosis.) ATHEROSCLEROSIS: EXCESS LDL CHOLESTEROL 1. Cholesterol is deposited in different tissues due to high LDL cholesterol fractions in blood plasma, which forms xanthomas (cholesterol deposits in skin). 2. This accumulates LDL cholesterol in endothelial blood vessels, where it is oxidized to produce oxLDL, triggering an inflammatory response. 3. oxLDL is taken up by macrophages, forming foam cells to which other compounds attach, causing a blockage of blood vessels. Cholesterol Synthesis: (cytoplasm of liver) Glucose ->. Pyruvate. -> Acetyl CoA 2 Acetyl CoA Glycolysis Thiolase HEME SYNTHESIS: > Acetoacetyl-CoA + acetyl CoA Acetyl CoA HMG-CoA synthase. HMG-CoA > HMG-CoA reductase + 2NADPH Succinyl-CoA + Glycine. Mevalonate B-aminolevulinate synthase. Mitochondria s ATP (phosphate donor) B-aminolevulinate (PLP as coenzyme) (2 condense) Cytoplasm. Phosphanevalonate s ATP (phosphate donor) Porphobilinogen B-aminolevulinin dehydrase (4 condense). J § 5-pyrophosphomevalonate I ATP (energy donor) s. Dimethylallyl pyrophosphate + delta3-isopentnyl pyrophosphate Uroporphyrinogen III (4 CO2 removed) KETONE BODIES SYNTHESIS & USAGE s. Geranyl Pyrophosphate (CIO) + phosphate Coproporphyrinogen III Acetyl CoA x3 \ (2 CO2 removed). > 2 x Farnesyl Pyrophosphate (C15) s B-hydroxy-B-methylglutaryl-CoA (HMG-CoA) Protoporphyrinogen Exogenous Pathway (digestion) -1 acetyl CoA (Iron 2+ is attached). s Squalene (C30) +.2 pycrophosphate groups Acetoacetate s Food NADPH (Oxygen & hydrogen donor) Heme Decarboxylation. Reduction. Saliva lipase. (Mouth) → Lanosterol $ Acetone D-beta-hydroxybutyrate. (Enter mitochondria) > Endogenous Pathway (digestion) DB hydroxybutyrate-dehydrogenase Cholesterol (27C). VLDL + lipids. (Liver) Acetoacetate Lipoprotein lipase (activated by apoC-11). (Tissue wall) GDP (from krebs cycle activates carboxyl group) n⇐¥¥=i.. IDL (+ cholesterol) Triglycerides Acetoacetyl-CoA DE NOVO BIOSYNTHESIS OF FATTY ACIDS Thiolase & CoA Cholesterol -> Bile Salt (Liver) Acetyl CoA + Oxaloacetate. Acetyl-CoA (travels to krebs cycle) i Citrate synthase (travels through mitochondrial membrane) ATP-citrate-lyase > E ) ma Acetyl CoA + oxaloacetate ⑤ p las od ¥ (blo Malic dehydrogenase + NADH ters Acetyl-CoA Carboxlase (activated by insulin) +CO2+ATP. , l es s. ero lest Malonyl-CoA Malate + cho LDL (with only ApoB-11 on surface) is: DL hes -> H Pancreatic lipase Malic enzyme TRIGLYCERIDE FORMATION ynt l s lt s ¥ ero AT Sa lest LC Cholesterol esters(fusing with lysosome) Acetyl-CoA attaches to 1 SH group of Synthetase, Malonyl-CoA to the second SH. Pyruvate + NADPH + H+ (travels into mitochondria) Fatty acid + CoASH (Outer mitochondrial membrane) H EM Bile + cho L Lysosomal enzymes HD H em E D Thiokinase & ATP. e EG Cholesterol (Extrahepatic tissues) Pyruvate carboxylase s > He R A Bil me DA ] Acyl-CoA ive rd (re xyg o TIO Beta ketobutyrl-ACP (combined Acetyl-CoA & Malonyl-CoA on Synthetase) -CO2 Oxaloacetate ME N: W in mo en Bil ves ase NADPH i÷ ive iro rd n) s in red uc Beta-Hydroxybutyryl-ACP tas e i. ~ Acyl-CoA+ Glycerol-3-phosphate (Removing H20). * s Trans-delta2-Butenoyl-ACP (double bond) # NADPH I Lysophosphatidic acid + Acyl-CoA(attaching) :: ÷ s Bilirubin diglucuronide Butyryl-ACP (transferred from phosphanthetine SH group to cysteine SH group) (secreted into small intestine) Phosphatidic acid Urobilin (kidney, eliminated via urine) s Urobilinogen Butyryl-ACP + Malonyl-CoA (repeat cycle) " 1,2 diglyceride + Acyl-CoA (attaching) Stercobilin (eliminated in feces) ✓ Triglyceride Smaller food E Bile salts. (Small intestine). in Emulsified triglyceride. Pancreatic lipase. 2-monoglyceride + 2Fatty acids ① ¥⇐. BETA OXIDATION OF FATTY ACIDS: Isomerase & lipase Glycerol + fatty acid + chylomicron remnant. (Target tissue wall). rig Aycl CoA Glycerol + fatty acid. Carnitine palmitoyl transferase 1. (Outer Mitochondrial membrane) Energy or stored (muscle/adipose tissure) E. 2-monoglyceride/glycerol with chylomicrons (Enterocytes) Carnitine (transporter) + Acyl CoA Lipoprotein lipase (actiavted by apoC-11) Carnitine palmitoyl transferase 2. (Inner Mitochondrial membrane) Acyl CoA. (Travels to krebs cycle inside mitochondria) METABOLISM OF BRANCHED CHAIN AMINO ACIDS - Glutamate + ammonia. (Muscles). Glycogen Acyl-CoA-dehydrogenase + FAD ¥÷ ATP Oxidized Acyl CoA (trans double bond) + FADH2 Glutamine (transported to liver) Glucose H20 Glycolysis Pyruvate + amino group (from amino acid) Hydroxacyl-CoA ¥: Hydroxyacyl-CoA dehydrogenase + NAD Ketoacyl-CoA + NADH Alanine. (Travels to liver) - CoA Alpha-keto-glutarate (Reversed o¥ : E e- Fatty acid + Acetyl-CoA Glutamate Pyruvate Gluconeogenesis E Urea. Glucose I GLYCEROPHOSPHOLIPID SYNTHESIS: L-glycerol-3-phosphate + 2 fatty acids. Alcohol + Diacylglycerol is ' ÷÷¥ CTP (activates either alcohol or diacylglycerol). phosphatidic acid CDP-ethanolamine. CDP-diacylglycerol PROSTAGLANDINIS SYNTHESIS: Membrane phospholipids + arachidonate Phosphlipase A2 Arachidonic acid Cyclooxygenase (COX) I e PGG2. COX Did PGH2 (prostaglandinis) ¥ of iE÷÷ LEUKOTRIENE SYNTHESIS: Oxygen + Arachidonate (Lipoxygenases) PURINE NUCLEOTIDES: Nitrogenous Base -> used as energy, DNA synthesis & SAM - Adenosine 5' monophosphate (AMP) Purine's have - Guanosine 5' monophosphate (GMP) II 2 rings. PATHWAY 1: DE NOVO BIOSYNTHESIS PURINE NUCLEOTIDES: 1. Starts with a P-ribose sugar with an NH2 attached (Phosphoribosylamine). 2. Glycine binds to phosphoribosyl-amine, forming Glycinamide ribonucleotide. 3. Vitamin B9 brings an aldehyde group to Glycinamide ribonucleotide, forming formylglycinamide ribonucleotide. 4. Glutamine is hydrated to Glutamate and ammonia. 5. Ammonia binds to formylglycinamide ribonucleotide, forming formylglycinamidine ribonucleotide. 6. The energy of ATP closes the ring and removes a water molecule, forming 5-aminoimidazole ribonucleotide. 7. With the energy of another ATP, a carboxyl group is added, forming an unstable molecule. 8. The carboxyl group is switched from the amino group to a carbon atom, forming carboxyaminoimidazole ribonucleotide (not unstable anymore) 9. With the energy of ATP, aspartate is attached. 10. Fumarate is removed, forming 5-aminoimidazole-4-carboxamide-ribonucleotide. 11. Vitamin B9 brings an aldehyde group to the molecule forming 5-formaminoimidazole-4-carboxamide ribonucleotide. 12. H20 is removed forming Inosinate (IMP). Formation of AMP: 13. Aspartate is added with the help of GTP, forming Adenylosuccinate. 14. Fumarate is removed, forming Adenylate (AMP) Formation of GMP: 13. NAD+ is oxidized and water is added