ECF and ICF, Ingesting Water PDF
Document Details
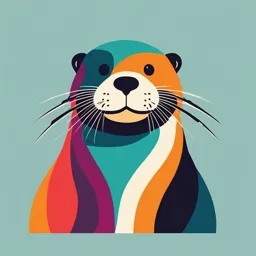
Uploaded by IrreplaceableFriendship8062
Tags
Summary
This document details the movement of water between extracellular fluid and intracellular fluid in response to the ingestion of water or salt. It explains how adding water dilutes the ECF and causes water to move to the more concentrated ICF by osmosis. Specific examples and calculations about volume and osmolarity of body fluids are discussed.
Full Transcript
I am. I am going to look at the ECF, ICF on the ingesting of water. So if you add a liter of water, what compartment will it enter? Where will it enter? Your body. We enter the cells directly. Plasma. It is the plasma, isn't it? It is the plasma. So that is the ECF, yeah? It is the cell in the fluid...
I am. I am going to look at the ECF, ICF on the ingesting of water. So if you add a liter of water, what compartment will it enter? Where will it enter? Your body. We enter the cells directly. Plasma. It is the plasma, isn't it? It is the plasma. So that is the ECF, yeah? It is the cell in the fluid. Okay. So I did one liter of water. So what happens to the ECF? Well this liter of water. The ECF, it kind of expands and is diluted as well. Okay. So that means that it is diluted. That means the ICF is more concentrated. The ECF is more diluted. So water will move to where the solvent is more concentrated. Water will move. We add water to the ECF. It does more dilute and it will move, as most is, from where there is low water level to high water level. Sorry. From where there is high water level to lower water level. High concentration of the solvent. So this new volume, so what happens now to the volume? That is when you add it in. Water enters the plasma. The ECF volume, as the plasma is an ECF, the ECF volume is expanded and diluted. It gets bigger. And then what happens now? Water moves by osmosis from the ECF, which where water has been added, to the ICF, which is more concentrated. Water will move across from where water is pellet 24 to where water is less. So what happens now? Both ECF and ICF, that is the extracellular and intercellular. Now have a larger volume and they are more dilute. So water will move to equilibrate the osmosis pressure across all body fluids. Sodium stays where it is, intercellular material stays, intercellular laterated water that will move by osmosis to create an equilibrium. So what if you do the opposite and ingest 10 grams of sodium chloride, assuming no water? So 10 grams of salt to the ECF, what happens? The ECF becomes what? Like I said, if you eat the large amount of salt, you will enter your blood plasma and the ECF will become a high concentration of salt and low water. So what happens to the sodium chloride enters the blood plasma, has a high concentration of salt. Water will do water from the ICF, we do want. It will move to the ECF. We see it as a high osmotic pressure, high concentration of solute, salt solution. So water will move across in order to create a balance. Same thing as you drink a lot of salt, you take a lot of salt into your body, ECF, osmotic pressure increases. So you will need to basically what it needs to dilute that salt. So water will be drawn from the cells into the blood. So what happens next? So overall, sodium chloride stays in the ECF, it's not going to go anywhere. Water will move by osmosis, it will be drawn, water follows the solute, remember. So water will now enter the ECF, because of the salt, the high amount of salt. And then ECF volume is larger, because water has entered it. And ICF volume is smaller, because water has moved. If the salt in the ECF, the ECF would be considered having a higher osmotic pressure. That's right, which draws in the water. So water will move across in order to try to dilute it basically. Water will always follow the solute. So there's a high concentration on the other side. Water will move automatically in order to maintain equilibrium, to try and dilute it. And biosmosis. So ECF volume is larger, because water has entered it. ICF volume is smaller, because water has moved across. And then the high osmotic pressure in both. And then the water has moved across. It won't consider effective changes to ECF volume and composition. We can assume water equilibrates. Water is aimless to restore homeostasis. It equilibrates the osmotic pressure across all body fluids. Sogenstasia is outside the cell. An intracellular material is staying intracellular. So you don't look at this same scenario in just a money to water. But to look at it. You're going to look at it with a calculation. So water enters plasma, expands the ECF. Lowering is the osmolarity. So ICF now has a higher concentration of solute. And so water will now move across. Water will move across. From where water is highest to where it's lowest. Where there's an increase in amount of solvent. So what's the end result? Sorry, we saw that new volume of both ECF and ICF is larger. But the more dilute, osmotic pressure is less than those. So okay, we have a 70 kilogram man, total body weight. So a total body water is 42 liters. ECF volume is 14 liters. What would be volume and osmolarity of body fluids after drinking 1 liter of water? What happens? We've looked at it, we're just going to calculate it. You'd have a calculation to do for homework. So if you can use these examples to do the problem for homework. You can use this formula. So 42 liters, sometimes 300 milleos moles equals 12,600. So that's the starting condition. So final osmolarity, concentration of solute, is calculated by osmol, divided by final body after drinking water. So what happens to the total volume? So dividing that, you know, it now becomes 293 milleos mole per liter. What would the volume and osmolarity of the body fluids be after drinking 1 liter of water? So it's a new volume of ECF. Again, volume is amount of blood concentration. Okay. So the amount, so the volume of the ECF becomes 14.3 liters. So again, the same thing, what's the volume and osmolarity of the body fluids after drinking 1 liter of water? Still the same problem. So ICF equals total body water minus the ECF because this total body water equals both of that. So 28 liters is the ICF interstitial fluid, 10.5 liters plasma, 3.5 liters. So total ECF is 14 liters. So 2 thirds is ICF and 1 third is ECF. So ICF is 28 liters. So ECF is 14 liters. So a new volume of ICF. So what would the volume and osmolarity of body fluids after drinking 1 liter of water? Again, volume is amount of blood concentration. It becomes 28.67 liters. So the total, since it's tabulated here, is a 70 kilogram man, total body water equals 2 liters. ECF, volume 14 and the osmolarity 300 liters more for the liter. What would the volume and osmolarity of the body fluids after drinking 1 liter of water? So before, it's 42 liters, total body weight, after drinking 1 liter of water, it now increases to 43 liters. So ECF before was 14 liters, now becomes 14.3. ICF, 28 liters becomes 28.67. And the really osmolarity concentration of the solute is now, it was 300 now to 293. So it's basically drinking a liter of water, has made the body fluids more dilute. The volume has increased. The added water has distributed itself evenly across the compartments. So the effect of ingesting 10 grams of sodium chloride, again, this is the starting point. It results in increased ECF because of the higher salt concentration, so water will move across. And then in the end, ECF volume larger, ICF volume smaller because of the movement of water and higher osmotic pressure in both. So it looks at that. And then, what does isotonic saline mean? What is isotonic? Sort of there are these sport strengths which are isotonic. What does that mean? This isotonic. Is isotonic saline. Before we had like 10 grams of salt. This is saline which is isotonic. What is isotonic mean? It's a composition as the salt. Yeah. So what's going to happen? Does water move across or? No, it's not. No, because it's the same. It's the same osmolarity. So it's not going to cause movement of water. It's not going to increase the concentration. It's not going to do anything. It does not cause movement of water or it plays into content and body cells. Nothing will happen. It's isotonic. It's the same concentration. It's the same osmolarity. So if you add isotonic saline into the ECF, it stays in the ECF because you've added it. It will increase volume because you've added. It will increase volume. It will increase the water. It increases the volume because you've added it. But it's not going to change the concentration. It will not cool water across. It will not be able to double that. No osmotic flow occurs because there's no gradients. Yeah. So isotonic saline does not cause any of the acid. It does not cause any movement of water. It doesn't make any difference. It will be slight increase in the volume. ECF volume is larger. The plasma volume will be larger. Add it sodium stays then. But as the mass of culture does not change. Also does not move. ICF stays the same. Sodium non-penic. Sodium doesn't move out. Sodium is mostly because there's no change in the water pressure. It's a concentration that you don't change. There's a question. If you look on the moon all, there's a question which is there. If you have to go home for homework and to use these examples. Use these examples to follow how to work that. We'll look at it next week. Okay, what are we doing? Distribution of fluid between compartments. We're going to... We'll answer some of these questions. These are basically... I can already explain that the capillaries are... Well, the site where exchange takes place. That's where you're going to have a movement. So there's an arterial end. If you're going to have blood coming from the heart, then you've got... You know, the vessels get smaller and smaller. Arteries to arterios, capillaries smaller and smaller. And so you've got these capillaries which are one cell thick, which are going to deliver substances required by the cells. They deliver the oxygen, the glucose, the hormones, whatever is required by the cell, which will be delivered through the capillaries. And then from the cells, and then the... Celestial arterial end and then the venous end. So you can see these arrows coming this way. So at the arterial end, you've got filtration happening. Okay, there's high pressure. So substance is a fluid and substances are filtered out. When the solution is filtered out from the capillaries, what is it called? What is it called? Substance is filtered out from the capillaries. Obviously it contains nutrients and it babes the cells. This becomes... This is forced out. This becomes tissue fluid, interstitial fluid, which babes the cells. And the cells will obtain their nutrients, oxygen, and nutrients from them. But when you get to the venous end, the capillaries will now write further. And then what happens, the veins, these venous capillaries, will now take the blood back to the big deep veins, and take it back to the heart to get rid of the waste. And then it will start again. But at the venous end, what's happening here? Is it still being filtered out? Why is it being filtered here? No, no. Does that make sense? Okay. At the arterial end, you've got the blood pressure, you've got blood pressure, high pressure. When it gets the capillaries, this forces fluid water, glucose, oxygen. The substances required by the stair cell are forced out of the capillaries into the tissue space, into the... It becomes interstitial fluid. That's the fluid that babes the cells. So the force here, the neck force at the arterial end, with the blood pressure, is high, and so fluid is pushed out of the capillaries so that it can supply the cells of what they need. But as you go along the capillary bed, as you go further down, the pressure will drop. This pressure of causing filtration will drop. And then, at the end, the tissue fluid starts coming back and entering the veins. It's a lower pressure. Okay, well, we'll come back. Do you know what the styling forces are? Okay, we'll look at what the styling forces are. There's two styling forces. The styling was an earnest styling, or the professor, scientist who worked in UCL many, many years ago. And this is where he did his work. And he was able to share this discovery of these pressures. So this is an earnest styling forces. These are the forces that are responsible for the fluid filtration and reabsorption between an exchange. So it's called exchange at the capillaries. So in the capillaries, that's where exchange occurs. Substances are filtered out water and dissolved substances, which are what he needs. They're filtered out, picked up by the capillaries. And then, at the venous end, further down, there's less pressure. Also, there's a higher amount, the capillaries contain proteins. And as this fluid is filtered out, pressure has dropped. So at this end, the capillaries still contain these proteins. And these proteins will now cause fluid to be drawn back into the capillaries biosmosis. Because there's proteins which don't pass out, they're too big to pass out. They're called the plasma proteins. So there's a pushing force. Neither arteries, whether pressure is high, this pushes stuff, this pushes fluid and nutrients out. And nutrients out of the capillaries took to the cells. And at the venous end, there's a pulling force, which pulls the tissue fluid back into the capillary venous capillaries and back into the bloodstream. So what are the styling forces? Hydrostatic and oncotic pressure. That's right. What do they do? What do these two forces do? Hydrostatic forces water out of the capillaries. And then the nutrients and then... That's right. So they're both opposing forces. Okay. They're opposing forces. So at the arterial end, high hydrostatic pressure. That's the blood pressure, pressure from the heart. Yeah? High blood pressure. And this pressure is high. I mean, the arterial pressure, you know, when you measure your blood pressure, say it's about 120 mm in mercury, it's high. And how you know is high is because it's painful because you have to apply a high pressure to actually close the artery. The artery is closed and then it's released and then starts coming up again and then you know the pressure. So it's very high pressure and that's the pressure. The arterial end of the capillaries, which is the hydrostatic pressure, which forces out. Remember the capillaries have pores, you know. So if you're forcing blood to your high pressure, it's going to come out through these pores and then into the tissue fluid, into the interstitial fluid, in between the cells. So the cells can actually pick up their nutrients and their oxygen and water. But as you go further down the capillary bed, pressure, this pressure coming from the heart is less. Further away from the heart is reduced in science because the arterial, the big arteries, become smaller arterials and then become capillaries. So become smaller and smaller and the pressure drops. But the capillaries contain protein albumin, it's a protein, a plasma protein which does not pass through, it cannot pass through the capillaries. It stays there. So further down in the venous capillaries, because of the albumin, the proteins, the plasma proteins, there's an osmotic pressure, because of these proteins. And this will now, at this end, the hydrostatic pressure has dropped, by the time it gets to the venous end. The hydrostatic pressure is low. So all the time you have these two forces. So the arterial end, you've got the colloidosmotic pressure or osmotic pressure caused by the proteins. That's there. But the arterial end, the hydrostatic pressure is greater and it forces out liquid, fluid, out of the capillaries into the interstitial fluid that babes themselves. The cells can receive their nutrients. But further down, the plasma proteins are still there, the proteins are still there. But now, because of the hydrostatic pressure, the hydrostatic pressure has dropped further away from the heart. So now, the force-favoring movement is now from the colloid, is from the octonic pressure. Because the hydrostatic has dropped further away from... When it gets to the venous end, hydrostatic pressure has dropped. So now, the colloidosmotic pressure or the aquatic pressure caused by the proteins, that now is the major force. And that force will pull... Because of the proteins, the hydrostatic pressure, it will pull in the fluid. The tissue fluid, interstitial fluid, will now be pulled back. So that's the design. So the arterial end is forced out because the arteries are carrying the life-giving, oxygenated blood, or the nutrients. But time you get to the venous end, it's mainly the wastes. It's mainly commoner upside and waste, which will now enter the venous system. It will be pulled in by the force exerted by the plasma proteins. And then it will go back up to the veins, back up to the heart, back to the lungs, and get basically......you know, pick up oxygen, and then it starts again. So that's the whole point. The whole point of the circulation is the exchange. That's the whole point of the heart, the blood vessels, is to bring the nutrients, water, whatever the cells need. That's the whole point. The exchange, the important part, is at the level of the micro-circulation. That's where the exchange takes place. That's where the cells in the body receive their nutrients and their oxygen. At the level of the micro-circulation. So the whole circulatory system is geared to the capillaries to actually, for this exchange to take place. So the red blood cells will pass through, they'll release their oxygen, and then they'll pick up CO2 on the way back. So it's a total, you know, it's an amazing trans-force system. So the starting forces are the hydrostatic pressure generated by the heart, the blood pressure. It forces the blood out of the capillaries into the cells, delivers nutrients. So that's the hydrostatic pressure. So the two forces are hydrostatic pressure and the aquatic pressure generated by the proteins. So the arterial end is the hydrostatic pressure, which is the dominant one in a favors filtration. So the renaissance, the force that favors reabsorption, which is a pulling force, pulls the fluid back in, is the aquatic pressure or corn oil or motic pressure, which is, you know, so this pressure is created by the proteins, which remain within the capillaries. And what role does the lymphatic system play in returning filtered water and solute to the sub-emotions? Anyone know that? In fact, it takes, what is the lymphatic system? What the lymphatic system does? There's quite a few functions. It's involved in, you know, in the, in, but we're basically, um, immunity, immune response. But it also plays a role here. What does the lymphatic system do? Does anyone know? The lymphatic system, you don't really notice it until it goes wrong. Is it a drainage system? It's a drainage system, correct. So basically, you've also got the lymphatic capillaries here as well. So basically, okay, the cells, you know, we know the substance is fluid, it's being kicked out into the interstitial space. So you've got increase in tissue fluid, this, you know, from the plasma, the fluid will go and will end up in between the cells. But then, it's drawn back in at the venous end, yeah, this tissue fluid. But not all of it is drawn back in. Ideally, it should be, but they're still remaining tissue fluid in between the cells. So this is picked up by what? What system drains the excess? The lymphatic drainage. The lymphatic capillaries will now pick up this excess tissue fluid. So some of it, most of it will go back into the venous capillaries and back to the veins. But then they'll be excess, which is picked up by, so ordinary, you don't notice it. The lymphatic drainage, it picks up the excess tissue fluid, insertion fluid in between the cells that was pushed out. Some of it is going to be pulled back in at the venous end. And some is not. That's an lymphatic system. Now, picks that up. The lymphatic capillaries will pick up the excess tissue fluid or intracilinidin, intracilinidin, intastitial fluid. And then it will return it to the circulation. That's what the lymph drainage. And normally, you don't notice the action of the lymphatic system until it goes wrong. Because sometimes, you see patients with, what's the condition called? You know what the condition is called when there's a problem with the lymphatic drainage? Okay, we'll come back. We'll come back to it. We'll come back to it. No, not gowns. We'll come back to it. So just have a quick look at the capillary wall. Again, it's one cell thick. It's got pores between the blood in the capillary and intracitial fluid and the last rate exchange. It's fully permeable to water and electrolytes ions. But it's not permeable to proteins. So the proteins in there will stay there. And then at the venous end, they'll create the osmotic braidants. Now draw fluid back in at the venous end because of hydrostatic pressure, which was opposing it has now dropped. So the solid concentration difference due to the proteins arise across the capillary wall. So the proteins stay in the capillaries. And when the hydrostatic pressure has dropped at the venous end of the capillaries, this pressure exerted by the proteins, the colloid osmotic pressure, is now what draws the fluid back in. Okay? So colloid osmotic pressure or on clotic pressure, osmotic pressure due to the protein across the set, you know, any but semi-permeable barrier. So this colloid osmotic pressure, which direction of fluid flow across the capillary wall does this force favor? Does it pull it in or does it push out the fluid? The protein, it pulls it back in because there's now a higher concentration within the capillary at the venous end, high osmotic pressure. Well, osmotic braidant, which now pulls in the tissue fluid, pulls it back in. So it basically is reabsorption. There's reabsorption at the venous end and there's filtration at the arterial end. So direction of fluid flow across the capillary wall from ISF into tissue fluid into the plasma is back into the plasma. Plasma resides in a container, any liquid exerts a force, more than the container, more directions. So this is the hydrostatic pressure, the arterial end, the hydrostatic pressure due to the blood pressure, or try or tend to drive fluid out to the container if there's a way out. So this hydrostatic pressure generated by the heart, the blood pressure, which direction of fluid flow across the capillary wall does this force favor? The hydrostatic pressure generated by the heart and the blood pressure of the arterial end, which direction across the... Is it... Out of the capillaries? Out of the capillaries, it promotes filtration. From plasma into the interstitial fluid, it forces blood out of the blood plasma. Sorry. It forces water and dissolved substances from the plasma into the interstitial fluid and that's how the cells can obtain their nutrients. So it's perfect design. In this context, so these are the starting forces, yeah? Cornelomotic pressure due to the proteins and hydrostatic pressure due to the blood pressure, they're known as the starting forces after the British scientist, from UCL across the road, who figured this out? Yeah. So to see that these starting forces, these two forces are the ones that influence fluid movement between the plasma in the capillaries and the interstitial space. So either the forces will push out the plasma or it will pull it back in. So these are two starting forces. So from the point of view of plasma, hydrostatic pressure tends to force fluid out and on aquatic pressure tends to pull fluid in. So they're opposing forces. So you consider the sum of the forces. So basically net pressure, the overriding pressure, the net pressure equals a hydrostatic pressure minus the colloid of the multi-pressure. So that's the net pressure. So at the arterial end, if hydrostatic pressure exceeds the on aquatic pressure, the net forces positive and fluid will move from plasma into the interstitial space, it's ISF, interstitial fluid, which baves the cells. So the cells can obtain the nutrients, the net filtration is out of it. And if this on aquatic pressure at the venous end exceeds the hydrostatic pressure, because the pressure will drop along the confidory bed as you get further away from the heart, the net forces negative. And this fluid will move from the ICISF into the plasma back into the capillaries. So basically these are two pressures. So net filtration occurs with the hydrostatic pressure, it's greater. And net absorption back into the capillaries occurs if on aquatic pressure is greater. So it's net forces, it's net forces. So one is greater than the other. So basically this is the capillary. So this is the arterial end of the capillary and this is the venous end. So here at the arterial end, near the heart, these are the forces, the starting forces. So the blue is the hydrostatic pressure, so it's 32 here. And then this is the on-quatic pressure, caused by the proteins, that's the red, so that's less. So the overall overriding, what will happen here? What's the main thing that will happen here? What direction will the blood in the plasma go? Today's the system for it. That's right, it would be pushed out to the cells which need it. And then at the other end, because this along, as it travels along the capillaries further away from the heart, basically this hydrostatic pressure has dropped now to 15 millimeters of mercury. But the on-quatic pressure exerted by the proteins is still the same, that's 25. But because the hydrostatic pressure has dropped, it means that on-quatic pressure, because of the proteins, is now dominant. Because it's dominant, it will now cause what? These proteins, before force they exert, will now cause what direction will the fluid go? It will be pulled back in, so the venous end, so the arterial end is pushed out, the venous end is pulled back in because of the osmotic pressure caused by the plasma proteins in the capillaries. So that's capillary fluid exchange. So, approximately, on-quatic pressure is constant along the length of the capillary, that's due to the plasma proteins. It's the hydrostatic pressure that forms. And the hydrostatic pressure declines along the length of the capillary, it reduces due to friction. It just gets lower and lower and lower. Overall, we look at the whole circulatory system, yes. As a term, choroid or esmotic pressure, or constant pressure, it's a changeable. It's the same, it means the same thing. Okay. So overall, across the capillary beds in the body, filtration always exceeds absorption. There's more blood. So basically, approximately three liters of fluid per day moves out of the circulation. Imagine three liters per day is forced out of the circulation into the intuspecial spaces. Three liters per day. Most of it is reabsorbed at the venous end, back, reabsorbed back. The filtration prefers to the things leaving the capillaries. Yeah, it's been forced out into the intuspecial space. It leaves the capillaries. And it enters the ISF, so it babes the cells. So most of it will go back. You know, everything's equal. Most of it will go back into the venous end, drawn in by the oncotic pressure or polo-dosmotic pressure, due to the proteins. Yeah. Most of it will go back in. So there's a lot of three liters of fluid per day. And the fluid, the tissue fluid or interstitial fluid, between the cells. So what happens to the ones that don't go to the fluid that doesn't return back into the circulation? What's it picked up by? What system picks it up? The lymphatic. That's right. So it's all kicked out. And then most of it goes back in at the venous end. And then what's left is mocked up by the lymphatic capillaries. And they return it to the circulation. So that's what deals with it. But what happens if there's a problem? There's a problem that there's something wrong. This is all things being equal. What happens if there's a problem with the lymphatic system? And it can't more pop the excess interstitial fluid. What happens to this tissue fluid? If there's a problem with the lymphatic system. So it returns the circulation by the lymphatic system. This is a good return. But the lymphatic system will pick it up and return it. Problem. Lymphedema. Abnormal accumulation. The veins, the arteries, blood capillaries. And then the green here, the lymphatic and the capillaries. And these are the blood cells. These are the body cells. So they're bathed in interstitial fluid. They're receiving all their oxygen and nutrients and water. And then at the venous end, they're going to pass it back to the veins and it goes up. But this should be picked up by the lymphatic capillaries. Abnormal accumulation of interstitial fluid causes lymphedema. Normally the lymphatic system, you don't notice them. You know, you're thinking, do we have a lymphatic system? But it's when there's a problem that you know you've got lymphatic system. So it can be caused by a damaged lymphatic system. Or problems with movement and drainage of the fluid, often due to an infection, injury or cancer treatments. It could be damaged by surgery, cancer surgery, or other forms of cancer treatment or inflammation of the lymph. So basically the leg or even, well, if you think about tropical disease, maybe we heard of elephantitis. Elephant leg basically is caused by worms, filarial worms, worms which actually, yeah. So that's not, that's not it. Anyway, so, but anyway, the result is a very swollen leg. Because the lymphatic cannot return this, it's called lymph. You cannot return this interstitial fluid back to the circulation. So it's a normal accumulation. And you see in patients with, you know, with lymphedema. And when you press it, because normal swelling, you press it, it indents. You know, it pushes in your finger, but with lymphedema it doesn't. It's almost like robbery, it's harm, but it doesn't go in. Because this is not, this is not just water, it's not just fluid. I don't know, but this is what. So patients have to have, there's lots of treatment, there's compression stockings, there's massage, there's all kinds of things to try and, you know, to try and improve it. How else can, well, what else causes like a swollen leg? It's quite a lot of things actually, but I'm just kind of looking at a few of them. Okay. You heard of deep veins rhombosis. That's formation of a blood clot within the deep veins of the leg, yeah? So it's just basically, it's a blockage in the veins. It's a blockage. Because remember the veins, so the blood in the veins is going back up. It's going back up to the heart and it's going back up to the lungs. But if there's a big blockage in the deep veins and seeing the thigh, how's the blood going to get back up? So as a result, there's a high, there's a pressure, there's a high pressure in the veins in the leg, high pressure. Because there's high pressure, how is the blood going to, the computer is going to get back in? Because high pressure, it doesn't, it would not. If there's a high pressure in the venous system, say that the ankle, then you've got the blood coming in, the arteries are bringing in blood with each heartbeat. So blood is, it's not being cleared. It should be going up the veins and out. So the more blood is coming in, where's it going to go? So you're going to get more filtration. And then, you know, yeah, so it's not increased arterial pressure, but arterial is the same, because the venous side should be low pressure, should be low pressure, so the blood goes back up. But because it's high pressure, because you can't leave the leg, then the blood is still entering the leg from the arteries. But it's not being cleared, it's not being pushed back up because of the clot. So it ends up with, you know, says, acup. So they're swelling below the blood blockage. There's lots of swelling, increasing tissue fluid. There's a lot of swelling. And so it has to be dealt with, blood thinner, you know, hipherin, more furin. And now there's new drugs, injections, which basically thin the blood and prevent clots and break down the clot. And with the ultrasound, you can actually see the clot in the vein. It's just a horrible, you can see this black clots in the vein, you know. So nowadays, the doctors can go in, the surgeons can go in, and they can actually remove it. They don't remove it, or they break it down and remove it. And the patient's given blood thinner. Okay. So they're swelling and inflammation. So that's deep vein from both of them. So where is this clot? So this clot here, where does it end up? Where could it end up? Why is it dangerous? It goes to the heart, and it goes to the lungs. Pulmonary embolism is basically clots within the lungs, and it can be fatal. So they're definitely, so that's an emergency. Whenever I see a patient with a DVT, deep vein from both of them, it's like, okay, you're not going anywhere. You need to get your blood thinner, and you need to be treated, or they can go in and remove it. Because it's going to, because it's, you know, it's aimless to go back up to the heart and the lungs, and that's not where you want blood clots, you know. All right. Because I'm a clinical person, so I do lots of work with patients. So as we do the cardiovascular system, and we do it, I'd like to, you know, just to pinpoint some interesting cases, or just to, you know, just to bring it to life. Yeah. Okay. I think we've finished, actually. So just wanted to see that. Okay, that's fine. Okay, we've finished for tonight. So have a look at your mood, or have a go. Use the examples that we've given, and have a go at that. There's a problem on there for you to look at. Use the equations, and use the examples, and try and work that out. Okay. Okay, thank you. Okay. Okay. Okay. Okay. Okay. Okay. Okay. Okay. Okay. Okay. Okay. Okay. Okay. Okay. Okay. Okay. Okay. Now it's just done. Okay, first.