Equilibrium and Ideal Gases in Pharmaceuticals PDF
Document Details
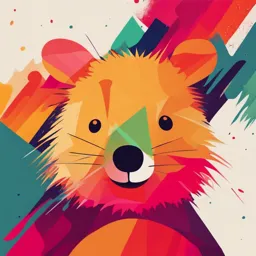
Uploaded by FragrantSpessartine
null
Tags
Summary
This document provides a detailed overview of the concepts of equilibrium and ideal gases within the context of pharmaceutical science. It explains how these concepts relate to dosage formulations and various processes in the body, such as drug binding and metabolism.
Full Transcript
Introduction to thermodynamics: equilibrium, ideal gases Thermodynamics = study of the energy of physical and chemical systems Thermodynamics and Pharmaceuticals 1. Dosage Formulation: o A dosage formulation is a specific preparation of a medicine that contains multi...
Introduction to thermodynamics: equilibrium, ideal gases Thermodynamics = study of the energy of physical and chemical systems Thermodynamics and Pharmaceuticals 1. Dosage Formulation: o A dosage formulation is a specific preparation of a medicine that contains multiple phases (such as solid, liquid, or gas) and components (active pharmaceutical ingredients and excipients). o The behavior of these formulations is governed by thermodynamic principles and equilibrium processes, which dictate how the components interact and how the formulation behaves under different conditions (e.g., temperature, pressure). 2. System: o A system refers to a defined portion of the physical world that is being studied. In pharmaceutical contexts, this could be the entire formulation or specific components of it. o Systems can be classified as open, closed, or isolated, depending on whether they exchange energy or matter with their surroundings. 3. Phase: o A phase is a homogeneous portion of a physical material that is separated from other portions by interfaces. Phases can be solid, liquid, or gas. o In pharmaceutical formulations, you may encounter multiple phases, such as solid drugs in a liquid solvent or emulsions containing both oil and water phases. 4. Component: o A component refers to a chemical ‘ingredient’ of the system. This includes both active ingredients (the pharmaceuticals that have therapeutic effects) and excipients (substances that serve as the vehicle or medium for the active ingredients). o Understanding the components and their interactions is crucial for designing effective dosage forms. 5. Pharmaceuticals(의약품): o Pharmaceuticals are the chemical components of medicines that are responsible for their therapeutic effects. This includes active pharmaceutical ingredients (APIs) and any other chemicals included in the formulation. o The pharmacokinetics (how the body absorbs, distributes, metabolizes, and excretes drugs) and pharmacodynamics (how the drug affects the body) are influenced by thermodynamic principles. Application of Thermodynamics in Pharmaceuticals Solubility: The solubility of drugs in various solvents is a thermodynamic property that influences formulation design. Stability: Understanding the thermodynamic stability of different phases helps in predicting the shelf life of pharmaceuticals. Interactions: The interactions between components can affect drug release and bioavailability. Equilibrium: Many formulations reach a dynamic equilibrium, where the rates of the forward and reverse processes are equal, influencing drug delivery and effectiveness. Conclusion The interplay of thermodynamics, phase behavior, and component interactions is essential for the development of effective pharmaceutical formulations. By understanding these principles, formulators can optimize drug delivery systems to enhance therapeutic efficacy and patient safety. Dosage Formulation and Equilibrium Processes A dosage formulation is a carefully designed system comprising multiple phases and components, where the interactions and transformations are governed by thermodynamics and process equilibria. Understanding these principles is essential for effective drug design and delivery. Examples of Equilibrium Processes 1. Binding of Drugs to Receptors or Enzymes: o The interaction between a drug and its target (receptor or enzyme) is a dynamic process that can be described using equilibrium constants. o The binding affinity of a drug to its receptor is often represented by the equilibrium constant (𝐾𝑑 ), which indicates how readily the drug binds to the receptor. This is a critical aspect of pharmacodynamics and influences the drug's efficacy. 2. Biochemical Reactions in Body Metabolism: o The metabolism of drugs involves a series of biochemical reactions that reach equilibrium, affecting how drugs are processed in the body. o Enzymatic reactions can be described by Michaelis-Menten kinetics, where the concentration of substrates and products defines the equilibrium state. 3. Processes for Manufacturing Active Pharmaceutical Ingredients (APIs): o The production of APIs often involves chemical reactions that can establish equilibria. Understanding these processes is vital for optimizing yield and purity. o Thermodynamic principles help in determining the conditions (temperature, pressure, concentrations) that favor the formation of the desired product. 4. Many Formulation Processes: o Formulation processes, such as mixing, encapsulation, and compounding, are influenced by the equilibria between different phases and components. o For instance, the solubility of a drug in a solvent can be viewed through the lens of solubility equilibrium, which affects the formulation's stability and release characteristics. 5. Measures of Pharmacological Activity: o Many pharmacological measures, such as potency and efficacy, can be described as equilibrium constants. o The concept of equilibrium is foundational in pharmacology, where the ratio of bound to unbound drug molecules dictates the overall activity of the drug in the system. Conclusion Understanding the equilibrium processes that govern drug formulation and interaction is essential for pharmaceutical science. By applying thermodynamic principles, researchers can predict and manipulate how drugs behave in biological systems, leading to more effective and safer medications. This knowledge is crucial for the design and optimization of dosage forms and enhances our understanding of drug action and metabolism. Pharmaceutical Analysis Pharmaceutical analysis is a critical aspect of drug development and quality control, focusing on the quantitative measurement of various properties that influence drug efficacy, stability, and safety. Key methods include: 1. Partitioning Between Phases Partitioning refers to the distribution of compounds between different phases (e.g., liquid-liquid, solid-liquid) and is crucial for understanding drug behavior in biological systems. Common analytical techniques for partitioning include: Chromatography: o Gas Chromatography (GC): Utilizes a gas as the mobile phase to separate volatile compounds based on their partitioning between the stationary phase and the mobile phase. It’s particularly useful for analyzing small, volatile drug molecules. o Thin-Layer Chromatography (TLC): Involves the separation of compounds on a stationary phase (often a glass plate coated with silica gel) using a solvent as the mobile phase. It provides qualitative and semi-quantitative analysis of compounds. o High-Performance Liquid Chromatography (HPLC): Employs a liquid mobile phase to separate compounds based on their interactions with the stationary phase. HPLC is highly effective for analyzing a wide range of pharmaceuticals, including those that are non-volatile or thermally unstable. 2. Enthalpy (Heat) Transfers Thermal analysis techniques measure the heat changes associated with physical and chemical processes, providing insights into stability and purity. 3. Other Quantitative Physical Effects Beyond partitioning and thermal analysis, pharmaceutical analysis can also involve other quantitative measurements: Spectroscopic Techniques: Techniques such as UV-Vis spectroscopy, infrared (IR) spectroscopy, and nuclear magnetic resonance (NMR) spectroscopy can provide qualitative and quantitative information about the chemical composition and structure of pharmaceuticals. Electrophoresis: A technique used to separate charged particles in a solution based on their size and charge, commonly used in the analysis of biomolecules like proteins and nucleic acids. Conclusion Pharmaceutical analysis employs a variety of quantitative methods to assess the properties of drugs and formulations. Understanding partitioning between phases, heat transfers, and other physical effects is essential for ensuring the quality and efficacy of pharmaceutical products. These analytical techniques play a vital role in drug development, quality assurance, and regulatory compliance, ensuring that medicines meet the required safety and effectiveness standards. Thermodynamics in Pharmaceuticals In the study of thermodynamics, particularly in pharmaceutical applications, it is essential to differentiate between the system and its surroundings. Understanding this distinction helps in analyzing how energy and matter are exchanged and transformed during chemical and physical processes. 1. System A system is a defined part of the physical world that is under investigation. It can be isolated or can interact with its surroundings. The system is where the relevant processes occur, and it can be characterized in several ways: Open System: Exchanges both matter and energy with the surroundings (e.g., a reaction vessel where reactants can enter and products can leave). Closed System: Exchanges energy but not matter with the surroundings (e.g., a sealed container where temperature changes occur without mass transfer). Isolated System: Exchanges neither matter nor energy with the surroundings (e.g., a thermos bottle, ideally). 2. Surroundings The surroundings refer to everything outside the system that can influence or be influenced by the system. This can include the environment, other systems, or any part of the physical world that interacts with the system. Understanding the surroundings is crucial for determining how changes in the system affect the overall processes. Examples in Pharmaceuticals 제약분야의 예 Dosage Form (투여 형태): A tablet or suspension can be considered a system where the active pharmaceutical ingredients (APIs) and excipients interact. The surroundings include the environment in which the drug is administered, such as the human body, where absorption and metabolism occur. Chemical Reaction in a Vessel: A reaction occurring in a laboratory flask is a classic example of a system. The reactants and products, along with the energy changes involved (e.g., heat), constitute the system. The surroundings would be the air and equipment surrounding the flask that may influence or measure the reaction. Biochemical System: In the context of pharmacology, the human body itself can be treated as a complex system. The biochemical reactions, interactions between drugs and biological targets (like enzymes or receptors), and physiological processes all represent a system. The surroundings include other physiological processes, external factors (like food or other medications), and environmental influences. Conclusion Understanding the concepts of system and surroundings is fundamental in thermodynamics, especially in the context of pharmaceutical sciences. This distinction helps in studying how drugs behave, their interactions, and the energetic changes that occur during pharmaceutical processes. By analyzing systems in relation to their surroundings, researchers can better understand drug formulation, delivery, and metabolism, leading to more effective therapeutic. Equilibrium in Pharmaceutical Systems Equilibrium refers to a state in which a system's properties remain constant over time, despite ongoing processes. In the context of pharmaceutical systems, understanding equilibrium is crucial for drug formulation, stability, and bioavailability. 1. Components of Pharmaceutical Systems Pharmaceutical systems often consist of multiple components that contribute to the overall properties and behavior of the formulation: Active Pharmaceutical Ingredients (APIs): The biologically active compounds that exert therapeutic effects. Excipients: Inactive substances used as carriers for the active ingredients, aiding in the formulation's stability, bioavailability, and release characteristics. Excipients can include binders, fillers, preservatives, and flavoring agents. 2. Phases in Pharmaceutical Systems Pharmaceutical systems can exist in different physical states or phases, each influencing the drug's behavior and performance: Solid Phase: Involves the crystalline or amorphous state of the API or excipients. Solid-state properties can affect solubility and stability. Liquid Phase: This can include solutions or suspensions where the components are dissolved or dispersed. In some cases, immiscible liquids may coexist, leading to phase separation. Gaseous Phase: Gases may be present in the system, influencing the formulation's properties, particularly in aerosol delivery systems. 3. Partitioning Between Phases Components in pharmaceutical systems can partition between various phases, affecting their concentration and activity. For example: Solubility: The solubility of an API in a liquid phase can determine its bioavailability. The distribution of an API between solid and liquid phases can also influence drug release from solid dosage forms. Interfacial Phenomena: The behavior of APIs and excipients at interfaces (e.g., between liquid and solid phases) can impact emulsification, stability, and absorption processes. 4. Dynamic Equilibrium In pharmaceutical systems, a dynamic equilibrium exists where the rates of the forward and reverse processes are equal, resulting in constant concentrations of components. This can be illustrated through: Chemical Equilibrium: In a reaction involving an API and its receptor, the binding and unbinding processes reach a state where the concentrations remain stable over time. Phase Equilibrium: In a system with multiple phases, such as a drug in a suspension, the solid and dissolved forms may reach a balance in their concentrations. Conclusion Understanding equilibrium in pharmaceutical systems is crucial for optimizing drug formulation and delivery. The interplay between components and phases can significantly affect the stability, solubility, and therapeutic efficacy of pharmaceutical products. By studying dynamic equilibrium and partitioning phenomena, pharmaceutical scientists can design more effective dosage forms that ensure consistent and reliable therapeutic outcomes. Law of Mass Action & Equilibrium Constants The law of mass action is a fundamental principle in chemical kinetics that describes the relationship between the concentrations of reactants and products in a chemical reaction at equilibrium. This law is essential for understanding how reactions proceed and how they can be quantified. 1. Chemical Reaction Representation Consider a reversible reaction where substances A and B react to form substances C and D. The reaction can be expressed as follows: Stoichiometry: The coefficients a, b, c, and d represent the stoichiometry of the reaction, indicating the ratio in which the reactants and products participate in the reaction. Reversible Reaction: The double arrow (⇌) indicates that the reaction can proceed in both the forward and reverse directions, meaning that A and B can form C and D, while C and D can also revert to A and B. 2. Equilibrium Constant (K) The equilibrium constant (K) is a numerical value that expresses the ratio of the concentrations of products to the concentrations of reactants at equilibrium. It is derived from the law of mass action and is defined for the reaction above as follows: Where: [C], [D],[A], and [B] are the molar concentrations of the respective substances at equilibrium. K is specific to a particular reaction at a given temperature. 3. Interpreting the Equilibrium Constant If K>1: The products (C and D) are favored at equilibrium, indicating that the reaction proceeds towards the formation of products. If K1: The drug is more soluble in the lipid phase (hydrophobic phase) than in the aqueous phase. This is typical of lipophilic (fat-soluble) drugs, which are able to easily cross cell membranes, which are lipid-rich. If Keq