The Cell - Week 4 PDF
Document Details
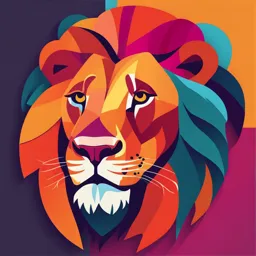
Uploaded by ModernPortland
null
Tags
Summary
This document covers the cell cycle, including G1, S, G2, and G0 phases, as well as cell cycle checkpoints. It also summarizes cell signaling and intracellular signaling transduction pathways.
Full Transcript
The cell cycle: G1- phase – cell cycle grow in size, synthesising mRNA and proteins required for DNA synthesis S- phase – DNA stored cells chromosomes is duplicated producing a second copy of genomic DNA G2- phase – further production of new proteins needed for pr...
The cell cycle: G1- phase – cell cycle grow in size, synthesising mRNA and proteins required for DNA synthesis S- phase – DNA stored cells chromosomes is duplicated producing a second copy of genomic DNA G2- phase – further production of new proteins needed for progression to mitosis G0- another state that is resting state a state were cell is not actively trying to divide and is instead simply trying to maintain normal functions Cell cycle is controlled by different cyclins being expressed by different points of cell cycle Cell cycle progression is controlled by sequential increased expression of 4 cyclins There also key check points of the cyclins levels and there are different check points which determines whether or not cycle progress from that point – G1/S - check points G2/M checkpoint Cell cycle checkpoints: This is where the cell reaches R point during G1 – where the cell checks quality of its DNA The damage is detected the cell cycle is arrested and DNA repair mechanisms are promoted There are 2 DNA checkpoints and each point the activation or deactivation of particular proteins that result in inactivation of cyclin/CDK complexes that control particular part of cell cycle. DNA damage checkpoints occur before cells enter S phase (G1-S checkpoint) and after DNA replication (G2-M checkpoint) G1/S checkpoint: P53 – guards the genome It serves as quality assurance checkpoint for cells for cells wanting to progress from G1 – S phase through R point P53 works in tandem with MDM2 and ATM - Ataxia telangiectasia mutated serine/threonine kinase. Results in production of Bax- Pro-apoptosis factor), p21 (to prevent cell cycle progression ARM/ATR – screens the genome looking for single strand breaks - therefore if anything phosphorylated p53 – this phosphorylation promotes transcription of downstream proteins involved in the upregulation of pro apoptotic factor Bax as well as Mdm2 and p21 P21 arrests the cell cycle and allows for DNA repair mechanisms to do their thing. the DNA can be repaired, the ATM/ATR – stops phosphorylating p53 and is marked by MDM2 for ubiquitination. This stops cell cycle arresting factors P21 and promotes production of cyclins for the next stage of cell cycle – if not repaired…undergoes apoptosis. G2/M – checkpoint: Once the cell has progressed through S phase and entered G2 this consolidate before can progress M phase Consolidation involves the production of pro-mitotic proteins and preparations of mitotic spindle ready for cell division The p53 has an active role in ensuring DNA is not damaged. DNA damage screening by ATM/ATR results in phosphorylation of P53 and CHK1 and 2 CHK1 and 2 halt cell cycle progression by phosphorylating multiple sites on the three Cdc25 phosphatases responsible for CDK1 activation. p53 further supresses CDK1 activity by promoting p21 14-3-3α expression 14-3-3αbinds to CDK/Cyclin B complex preventing its import into the nucleus, preventing mitosis. Check-points role in preventing disease: DNA checkpoints are only activated in response to DNA damage, and are activated depending on where the damage occurs If DNA damage occurs during mitosis or in G1, the G1/S checkpoint is activated If the damage occurs in S phase, the G2/M checkpoint is activated. If DNA damage is not detected and dealt with before progression to the next stage of the cell cycle, that becomes the new “template” for what is considered healthy DNA. Cell Signalling Cell signalling receptors: Signalling molecules (ligands) bind to receptors present either intracellularly or extracellularly on target cells Signalling molecules themselves can have different properties, molecules like steroids for example are small hydrophobic molecules and can therefore move through the plasma membrane unimpeded. Alternatively, signalling molecules are able to bind to extracellular receptors to either be internalised following receptor binding or lead to the induction of a signalling cascade. Summary of cell signalling: 1. Signalling ligand molecule is produced this is released and able to bind to relevant receptor 2. Binding of the ligand to receptor results in a intracellular signal transduction – this involves several intermediates 3. Activation of cellular response – immediate changes/slower changes Cell signalling responses usually relative to actual need of the cell in response to external stimulus – the rate of response reflects this. Signal Reception: Conversely, you would rather a gene expression change be a more gradual process perhaps. Changes in gene expression are tightly regulated by a number of transcriptional regulatory elements. Let’s now break the three stages down, starting with signal reception. Signal reception – interaction between ligand and receptor Receptors like enzymes, have active sites When addressing receptors referred to as Ligand binding domains ( LBD ) LBDs – are regions of receptors which are capable of forming temporary non covalent bonds between themselves and a signalling molecules – this is called Ligand Receptors/Ligand binding complexes usually result in conformational change within receptors activating its function. Ligand and Receptors interaction: Ligands are chemical groups which are able to bind to and effect receptor molecules found both intracellularly and extracellularly A Ligands affinity for a given receptor is described using a disassociation constant (Kd) Smaller number = Increased affinity What chemicals can act as cell signalling ligands? Such as… Hormones (eg: noradrenalin) Cytokines (eg: NF-κβ) Growth Factors (eg: Endothelial growth factor) Neurotransmitters (eg: Serotonin) ATP Pheromones Cell signalling receptors usually fall in to 4 distinct categories. Cell surface receptors fall into 3 of these categories Ion Channel linked (Acetylcholine (Ach) ligand gated channel) G – protein coupled (Rhodopsin) Enzyme coupled receptors (Vascular endothelial growth factor receptor) The final category are Intracellular receptors (eg: Inositol triphosphate (IP3) receptor) Interactions within the receptor/ligand complex are denoted as hydrophilic interactions, or polar interactions. These interaction points are vital for the correct orientation of the ligand within the receptors binding domain pocket. Computer models are capable of predicting this interaction, but there are limitations. Binding of the ligand to the receptor usually causes a conformational change within the receptor. This change varies depending on the type of receptor binding Signal reception – Ion Channel linked receptors: Linked to acetylcholine receptors found in muscles Acetylcholine binds to extracellular binding domain of ion channel – conformational change within structure of membrane protein Results in increased permeability of membrane to sodium ions Signal reception – G protein coupled receptors Most common variety of receptor – 700 different types They are most diverse group of receptors – involved in response to widest variety of signals G-protein coupled receptors characterized by large extracellular ligand binding domain, transmembrane domain – protein binding intracellular domain. Red – extracellular binding domain Purple – 7 TM domain Blue – G- protein binding domain G – proteins – Guanine nucleotide binding proteins act as molecular switches G- proteins contain 3 distinict domains - an α,β and γ subunits. Two of the three subunits are tethered onto the intracellular side of the plasma membrane through short lipid tails G – proteins are held in an inactive state until the GDP within the α subunit is substituted for a GTP group Signal reception – G-Protein coupled receptors GDP containing alpha subunit re- GTP containing associates with alpha subunit beta/gamma associates subunits to return to with target resting state. protein Intrinsic GTPase activity of alpha subunit deactivates protein once signal has been sent Ion channels Once G-protein has performed its function, it needs to return to a resting state Ligand binding promotes a Binding of GTP results in the conformation change in release of the beta/gamma G the intracellular protein complex, resulting in domain, allowing for G- its activation. protein binding Activated β/γ subunit moves to receptor, inducing This results in the conformational change substitution of GDP activating the protein channel with GTP within the (in this instance) alpha subunit Once the signal has been G-Proteins transmitted and the effect has been have intrinsic This allows the realised, the alpha subunit GTPase reformation of the hydrolyses the GTP molecule, activity, and is relaxed g protein state releasing a phosphate returning it to a vital (inactive state). GDP. component for returning the resting state Signal Reception - Enzyme coupled receptors ECR – second group of transmembrane receptors that have intrinsic enzymatic activity Unlike G-protein coupled receptors – enzymatic activity, ECR act as either the following: 1. Act as enzyme itself 2. Facilitate downstream via enzyme linked activation of intermediate complexes. Coupled enzymes receptors are receptors tyrosine kinases, which can be either receptors or non-receptor mediated signalling receptors. There are 4 types of enzyme coupled receptors: Receptor tyrosine kinases (RTK’s) Receptor serine threonine kinases Receptor tyrosine phosphatases Receptor guanylyl cyclases - Of these, RTK’s are the largest group of enzyme coupled receptors, with the 58 known RTK’s being sub divided into 20 sub families - Enzyme coupled receptors have a ligand binding extracellular domain, a single transmembrane alpha helix, and a cytoplasmic tyrosine kinase domain - Inactive enzyme coupled receptors exist as monomeric proteins, following ligand binding RTK’s form protein dimers leading to activation of the tyrosine kinase domain Receptor Tyrosine Kinases – RTK’s 1. Ligand binding leads to formation of RTK Dimer 2. Dimer formation activates intrinsic tyrosine kinase activity 3. Kinase activity self phosphorylates the receptor, activating it 4..Phosphorylated domains recruit cell signalling intermediates, allowing for signal transduction 5. RTK phosphorylation is regulated by protein tyrosine phosphatases (PTP), removing phosphates and deactivating the receptor following ligand release and returning it to the resting state Signal Reception – Non- receptor Tyrosine kinase receptors: Vary slightly from RTK’s in that ligand binding does not induce phosphorylation of the receptor in the first instance. Ligand binding leads to phosphorylation of the non-receptor kinase located on the cytosolic domain of the receptor. Once phosphorylated, the TK works as a protein kinase, phosphorylating the cytosolic domain of the receptor Signalling transduction… The next step in a cell signalling mechanism is signal transduction. Most cell signalling mechanisms have multiple steps, requiring multiple intermediate molecules in order to promote a response. Major components of the intracellular signalling cascade are secondary messengers Intracellular signalling transduction: Secondary messenger molecules are a vital component of some signalling pathways. A few examples are: Calcium Cyclic AMP (cAMP) Cyclic GMP (cGMP) Inositol triphosphate In addition there are a number of proteins which control the progression of a signal via: Phosphorylation Acetylation Methylation The number of steps involved in cell signalling is a means of cells controlling the activation of certain pathways with a number of different checkpoints. Each of these proteins within the pathway are changed in some capacity, allowing them to transmit the signal further down the chain. The incorporation of multiple branching pathways is also important for signal amplification. When a extracellular signal is received by a cell – its common for the ligand to be circulated at low levels to prevent over activation of signal This is so the signal activates the appropriate response it is amplified through a step by step process within the cytoplasmic domain. Intracellular signalling: PPC degrades phosphatidyl inositol (PTI) Extracellular releaseing IP3 signal binds to into cytosol, receptor (in this leaving behind example a G- Diacyl glycerol Protein coupled (DAG) receptor) PKC then functions to activate a number of downstream targets. G-Protein mediated activation of DAG activates protein kinase phospholipase C IP3 binds to calcium channels located within the smooth endoplasmic C (PKC), but is dependent on (PPC) calcium ions to function. reticulum releasing Ca2+ Intracellular signalling transduction – direct ligand/receptor binding Some ligands are small hydrophobic molecules; these are able to diffuse across the plasma membrane e.g. steroids and nitric oxide. These can bind with intracellular receptor proteins in the cytosol or nucleus. This is the point where we are getting dangerously close to the final stages of the cell signalling pathway… Cellular Response: The final step is the cellular response. Once the signal has run along the cytosolic signal transduction pathway, it reaches the response elements The cellular response is usually mediated by the activation of nuclear receptors. Nuclear Receptors: Nuclear receptors directly regulate the transcription, and are able to bind directly to the host DNA. There are 48 known nuclear receptors within the human genome. They have a common structure, comprising of a highly variable amino terminal domain, a central conserved DNA binding domain, and a conserved carboxy-terminal ligand binding domain There are 4 types of nuclear receptors Type 1 – Ligand binding in the cytosol results in disassociation of a heat shock proteins (HSP) which serves as the regulator of the nuclear receptor. Two ligand bound mono-subunits come together to form a homodimer, enter the nuclear membrane, and serve as a transcription factor promoting translation of DNA into mRNA Type 2 – receptor is bound to the DNA sequence directly as hetero dimers, with ligand binding stimulating the activation of transcription through the disassociation of a co-repressor and the incorporation of a co-activator molecule Type 3 – similar to type 1, but they bind to direct repeats instead of inverted repeat hormone response elements (HRE) Type 4 – Can bind as monomers or dimers, but only contains a single DNA binding domain NR/H’s form NR/H dimers and In this are translocated example, a through the lipid soluble nuclear hormone (H) membrane (oestrogen) through nuclear passes pores. through the membrane and binds to the Nuclear receptor(NR)/ Heat shock Once mRNA has been protein(HSP) produced, it is complex translocated out of the nuclear envelope and into the cytoplasm Binding results in disassociation of the HSP, releasing the NR/H complex NR complex binds to response element (in this case a hormone response NR dimer recruits transcription element) once inside the Gene activation results in factor co-activators to promote nucleus changed cell function. transcription Juxtracrine signinaling – DIRECT: Juxtacrine (Direct) signals require direct physical contact between the cell sending the message and the cell receiving the message. There are three main categories of direct signalling: Membrane bound ligands found on one cell are directly interacting with membrane bound cells on the next cell Communicating junction which links the two cells, allowing for transport of small/simple molecules between cells. Extracellular matrix glycoprotein and membrane protein interact. Notch signalling is a highly conserved cell signalling pathway which is present within most eukaryotic cells. Notch proteins are single pass transmembrane proteins, containing a extracellular ligand binding domain, a transmembrane domain and an intracellular effector domain. Ligands are presented by the cell sending the signal, collectively referred to as Delta jagged ligands (JAG1/2). They bind to the extracellular domain of the Notch protein, activating the intracellular domain of the receptor. Both cells are affected by ligand binding. The signal sending cell retains the Notch extracellular domain (NECD) following cleavage via intrinsic protease enzymes found within the Notch protein (activated after ligand binding). The signal receiving cell, γ-secretase complex releases the Notch intracellular domain (NICD) into the cytosol. NICD trans-locates to the nucleus where it acts as a nuclear receptor, associating with CSL promoting Notch associated gene targets. Cell/Cell junctions (aka: Gap Junctions) serve as communication channels between adjacent cells “Coupled” cells are capable of sharing small molecules (Molecular weight