Physiological Template PDF
Document Details
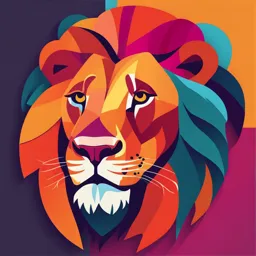
Uploaded by ExaltingRhinoceros
Hyacinth: BSP
Tags
Summary
This document provides a comprehensive overview of drugs, their effects, types and mechanisms of action, and different administrative routes. It covers topics from basic drug definitions to various injection and intake methods.
Full Transcript
Drug It is a medication that we would obtain from a pharmacist—a chemical that has a therapeutic effect on a disease or its symptoms. It is a chemical that people are likely to abuse, such as heroin or cocaine. It is “an exogenous chemical not necessary for normal cellular functioning that significa...
Drug It is a medication that we would obtain from a pharmacist—a chemical that has a therapeutic effect on a disease or its symptoms. It is a chemical that people are likely to abuse, such as heroin or cocaine. It is “an exogenous chemical not necessary for normal cellular functioning that significantly alters the functions of certain cells of the body when taken in relatively low doses.” It is effective in low doses. ○ This qualification is important because large quantities of almost any substance—even common ones such as table salt—will alter the functions of cells. Exogenous means produced from outside the body. Hence, it rules out chemical messengers the body produces, such as neurotransmitters, neuromodulators, or hormones. Chemical messengers produced by the body are not drugs. Drug effects are the changes we can observe in an individual’s physiological processes and behavior. For example, the effects of codeine, morphine, and other opiates include decreased sensitivity to pain, slowing of the digestive system, sedation, muscular relaxation, constriction of the pupils, and at high doses, euphoria. Sites of action are the points at which molecules of drugs interact with molecules located on or in cells of the body, thus affecting some biochemical processes of these cells. For example, the sites of action of the opiates are specialized receptors situated in the membrane of some neurons. Psychopharmacology It is a sub-discipline in the field of pharmacology. It is the study of the effects of drugs on the nervous system and behavior. It has been responsible for the development of psychotherapeutic drugs, which are used to treat psychological and behavioral disorders. It has also provided tools that have enabled other investigators to study the functions of cells of the nervous system and the behaviors controlled by particular neural circuits. Psychopharmacologists study drugs that affect the nervous system and behavior in two broad classes: therapeutic drugs and drugs of abuse. Pharmacokinetics includes the steps by which drugs are (1) absorbed, (2) distributed within the body, (3) metabolized, and (4) excreted. First, drug molecules are absorbed into the body based on how they are administered. After being absorbed, drug molecules are distributed throughout the body by blood in the circulatory system, including to the CNS where many sites of action are located. At the same time, drug molecules are being metabolized, or changed into an inactive form, by enzymes. ○ Most drug-metabolizing enzymes are located in the liver. Finally, drug molecules are excreted and removed from the body, typically by the kidneys. For laboratory animals the most common route is injection. Intravenous (IV) injection — injection into a vein. The drug is absorbed and distributed immediately, reaching the brain within a few seconds. The disadvantages of IV injections are the increased care and skill they require in comparison to most other forms of injection and the fact that the entire dose reaches the bloodstream at once. Intraperitoneal (IP) injection — rapid but not as rapid as an IV injection. The drug is injected through the abdominal wall into the peritoneal cavity—the space that surrounds the stomach, intestines, liver, and other abdominal organs. IP injection is commonly used to administer drugs to small laboratory animals. Intramuscular (IM) injection — made directly into a large muscle, such as those found in the upper arm, thigh, or buttocks. The drug is absorbed into the bloodstream through the capillaries that supply the muscle. Subcutaneous (SC) injection — injected into the space beneath the skin. Oral administration — the most common form of administering therapeutic drugs to humans. Some chemicals cannot be administered orally because they are destroyed by stomach acid or digestive enzymes or because they are not absorbed from the digestive system into the bloodstream. Sublingual administration — placing drugs beneath the tongue. The drug is absorbed into the bloodstream by the capillaries that supply the mucous membrane that lines the mouth. Inhalation — lungs as a provider of another route for drug administration. Many general anesthetics are gases that are administered through inhalation. The route from the lungs to the brain is very short, and drugs administered this way have very rapid effects. Topical administration — drugs absorbed directly through the skin. Natural or artificial steroid hormones can be administered in this way, as can nicotine (as a treatment to make it easier for a person to stop smoking). The mucous membrane lining the nasal passages also provides a route for topical administration. Commonly abused drugs such as cocaine and bath salts are often sniffed so that they come into contact with the nasal mucosa. This route delivers the drug to the brain very rapidly. The technical, but rarely used name for this route is insufflation. Note that sniffing or “snorting” is not the same as inhalation; when powdered drugs are sniffed, they enter circulation through the mucous membrane of the nasal passages, not the lungs. Intracerebral administration — injecting a drug directly into the brain. Intracerebroventricular (ICV) administration — a route used very rarely in humans—primarily to deliver antibiotics directly to the brain to treat certain types of infections. To achieve widespread distribution of a drug in the brain, a researcher will get past the blood–brain barrier by injecting the drug into a cerebral ventricle. The drug is then absorbed into the brain tissue, where it can exert its effects. Drugs exert their effects only when drug molecules reach their sites of action. In the case of drugs that affect behavior, most of these sites are located on or in cells in the CNS. anxiety. The effects of one dose of this drug can last more than 24 hours, in part because some of the metabolized molecules are active in the body for even longer than the original drug molecule. The most important factor that determines the rate at which a drug reaches sites of action within the brain is lipid solubility, or the ability of fat-based molecules to pass through cell membranes. The blood-brain barrier is a barrier only for water-soluble molecules. Molecules that are soluble in lipids pass through the cells that line the capillaries in the CNS, and they rapidly distribute themselves throughout the brain. For example, heroin (diacetylmorphine) is a more lipid-soluble drug than morphine. Thus, an intravenous injection of heroin produces more rapid effects than does one of morphine. Even though the molecules of the two drugs are equally effective when they reach their sites of action in the brain, the fact that heroin molecules get there faster means that they produce a more intense “rush,” and this explains why the abuse potential of heroin is greater than that of morphine. Drugs vary widely in their effectiveness. The effects of a small dose of a relatively effective drug can equal or exceed the effects of larger amounts of a relatively ineffective drug. The best way to measure the effectiveness of a drug is to plot a dose-response curve. Drugs do not remain in the body indefinitely. Many are metabolized and deactivated by enzymes, and all are eventually excreted, primarily by the kidneys. The liver plays an especially active role in the enzymatic deactivation of drugs, but some deactivating enzymes are also found in the blood and brain. Occasionally, the transformed molecule is even more active than the one that is administered. In such cases, the effects of a drug can have a very long duration. For example, chlordiazepoxide (Librium) is used to treat symptoms of Because the molecules of most drugs distribute themselves throughout the blood and then throughout the rest of the body, a heavier individual (human or laboratory animal) will require a larger quantity of a drug to achieve the same concentration as a smaller quantity will produce in a smaller individual. Most drugs have more than one effect. The most desirable drugs have a large margin of safety. Therapeutic index One measure of a drug’s margin of safety. This measure is obtained by administering varying doses of the drug to a group of laboratory animals or human volunteers. The lower the therapeutic index, the more care must be taken in prescribing the drug. Why do drugs vary in their effectiveness? Sites of action Affinity of a drug with its site of action Affinity It is the readiness with which the two molecules join together—for the sites to which they attach. A drug with a high affinity will produce effects at a relatively low concentration, whereas a drug with a low affinity must be administered in higher doses. The most desirable drug has a high affinity for sites of action that produce therapeutic effects and a low affinity for sites of action that produce toxic side effects. Tolerance is a phenomenon that diminishes the effects of a drug when administered repeatedly. Sensitization is a phenomenon when a drug becomes more and more effective. Withdrawal symptoms are primarily the opposite of the effects of the drug itself For example, heroin produces euphoria; withdrawal from it produces dysphoria—a feeling of anxious misery. Heroin also produces relaxation; withdrawal from it produces agitation. Physical dependence is when a person has repeatedly used a drug enough to produce withdrawal symptoms when they stop using it. It is one aspect that contributes to substance abuse. Placebo is an inactive substance that can produce physiological or psychological effects. The placebo effect occurs when an individual expects that a placebo can have a physiological or psychological effect, and the placebo subsequently produces an effect. Scientists have learned to produce completely artificial drugs, known as synthetic drugs, some with potencies far greater than those of naturally occurring drugs. Most drugs that affect behavior synaptic transmission. Antagonists — block or inhibit the postsynaptic effects Agonists — facilitate the postsynaptic effects The sequence of synaptic activity 1. Neurotransmitters are synthesized and stored in synaptic vesicles. 2. The synaptic vesicles travel to the presynaptic terminal membrane, where they become docked. 3. When an axon fires, voltage-dependent calcium channels in the presynaptic membrane open, permitting the entry of calcium ions. 4. The calcium ions interact with the docking proteins and initiate the release of the neurotransmitters into the synaptic cleft. 5. Molecules of the neurotransmitter bind with postsynaptic receptors, causing particular ion channels to open, which produces excitatory or inhibitory postsynaptic potentials. 6. The effects of the neurotransmitter are kept relatively brief by their reuptake by transporter molecules in the presynaptic membrane or by their destruction by enzymes. 7. The stimulation of presynaptic autoreceptors on the terminal buttons regulates the synthesis and release of the neurotransmitter. Enzymes control neurotransmitter synthesis. Effects on Production Neurotransmitters of Neurotransmitters are synthesized by presynaptic neurons. ○ Some drugs act as precursors. Precursor drugs are considered agonists because administering them increases the activity of the neurotransmitter system. ○ Some drugs deactivate enzymes. Such drugs are antagonists because they will prevent the neurotransmitter from being produced. Effects on Storage & Release of Neurotransmitters Neurotransmitters are stored in synaptic vesicles. ○ Vesicle transporters ○ Some drugs block vesicle transporters by binding with a particular site on the transporter and inactivating it. Neurotransmitter release at the terminal button. ○ Some drugs prevent release ○ Some drugs trigger release Vesicle transporters pump molecules of the neurotransmitter across the vesicle membrane, filling the vesicles. What is the difference between vesicle transporters and terminal membrane transporters? The terminal membrane transporters move the molecules from the synapse into the cytoplasm of the presynaptic cell. The vesicle transporters then move the molecules into the vesicles. Botulinum toxin (Botox) prevents the release of acetylcholine. Thus, blocking signals for muscle contraction. Effects on Postsynaptic Receptors Direct agonist — mimics the effects of NT. Direct antagonist / Receptor blocker — binds with receptor but does not activate it & prevents natural ligand from binding with receptor. Indirect agonist — binds to an alternative site & facilitates ion channel opening. Indirect antagonist — Binds to an alternative site & prevents ion channel opening. Drug Actions at Binding Sites Competitive binding — direct agonists and antagonists act directly on the neurotransmitter binding site. Noncompetitive binding — Indirect agonists and antagonists act on an alternative binding site and modify the effects of the neurotransmitter on the opening of the ion channel. What are the two processes that remove the neurotransmitter from the synapse? Molecules of the neurotransmitter are taken back into the terminal button of the presynaptic cell through the process of reuptake or they are deactivated by an enzyme. Effects on Reuptake or Destruction of Neurotransmitters Block reuptake — agonist Prevent destruction — agonist Cocaine Inactivate transporters responsible for reuptake Increased NT Neurotransmitters and Neuromodulators In the brain, most synaptic communication is accomplished by two amino acid neurotransmitters: one with excitatory effects (glutamate) and one with inhibitory effects (gamma-aminobutyric acid, or GABA). In general, neurotransmitters have modulating effects rather than information-transmitting effects. Amino Acids Some neurons secrete simple amino acids as neurotransmitters. Because amino acids are used for protein synthesis by all cells of the brain, it is a challenge to prove that a particular amino acid is a neurotransmitter. Two specific amino acids are especially important because they are the most common neurotransmitters in the CNS: Glutamate GABA GLUTAMATE the main excitatory neurotransmitter in the brain and spinal cord Precursor: glutamine Enzyme: glutaminase Ionotropic receptors ○ NMDA receptor — controls Ca2+ channel AMPA receptor — controls Na+ channel; most common glutamate receptor; when glutamate attaches to the binding site, it produces EPSPs. ○ Kainate receptor — controls Na+ channel Metabotropic receptor ○ Metabotropic glutamate receptor ○ Excitatory amino acid transporter — removes glutamate from the synapse Glutamine synthase — breaks down glutamate A failure to remove glutamate from the synapse can have negative consequences. ○ Too much glutamate stimulation in the synapse can produce glutamate excitotoxicity and damage neurons by prolonged over-excitation. GABA (gamma-aminobutyric acid) is the main inhibitory NT in the brain and spinal cord Precursor: glutamic acid Enzyme: glutamic acid decarboxylase or GAD Allylglycine — prevents GABA synthesis by inactivating GAD Vesicle GABA transporter — packages GABA into vesicles Without the activity of inhibitory synapses, the interconnections of the neurons in the brain would make the brain unstable and lead to seizure. Some investigators believe that one of the causes of seizure disorders is an abnormality in the biochemistry of GABA-secreting neurons or GABA receptors. Ionotropic receptor ○ 𝐺𝐴𝐵𝐴𝐴 — controls chloride channels Muscimol — direct agonist Bicuculline — direct antagonist Indirect agonists ○ Benzodiazepines — anxiolytic, reduced seizure activity, muscle relaxation ○ Barbiturates — an older class of sedative and antianxiety drugs ○ Alcohol — binds with an as-yet-unknown site on the 𝐺𝐴𝐵𝐴𝐴 receptor Indirect antagonist ○ Picrotoxin — has effects opposite to those of benzodiazepines and barbiturates: It inhibits the activity of the 𝐺𝐴𝐵𝐴𝐴 receptor GABA transporters — remove GABA from the synapse ACETYLCHOLINE (ACh) Is the primary neurotransmitter secreted by axons of the PNS that terminate at muscle cells to control muscle contraction Pathways/Major concentrations in the CNS ○ Dorsolateral Pons – REM sleep ○ Basal Forebrain – learning ○ Medial septum — formation of particular kinds of memories Precursors: choline & acetyl coenzyme Enzyme: choline acetyltransferase Vesicle ACh transporter — loads ACh into vesicles Two drugs, botulinum toxin (an extremely potent poison & stops muscular contractions) and the venom of the black widow spider (stimulates the release of ACh & the venom is much less toxic than botulinum toxin), affect the release of ACh. Ionotropic receptor ○ Nicotinic receptors — rapid-acting Metabotropic receptor ○ Muscarinic receptors — slower & more prolonged Acetylcholinesterase down acetylcholine — breaks The Monoamines Monoamines are considered “classical” neurotransmitters. Classical neurotransmitters are a family of relatively small molecules that include the monoamines and ACh. On the other hand, peptide neurotransmitters are larger molecules that undergo a different type of synthesis. DOPAMINE (DA) Catecholamine synthesized from L-DOPA Produces both EPSPs & IPSPs Major CNS dopaminergic systems include ○ Nigrostriatal system — control of movement ○ Mesolimbic system — reinforcement & reward ○ Mesocortical system — short-term memories, planning, strategy preparation for problem-solving ○ Tuberoinfundibular — prolactin release Only neurons that release norepinephrine contain dopamine β-hydroxylase; however, both dopamineand norepinephrine-releasing neurons contain tyrosine hydroxylase. Parkinson’s Disease — degeneration of dopaminergic neurons that connect the substantia nigra with the caudate nucleus. It is a movement disorder characterized by Tremors Rigidity Akinesia Postural instability The cell bodies of these neurons are located in a region of the brain called the substantia nigra (“black substance”). This region is normally stained black with melanin, the substance that gives color to the skin. AMPT — blocks tyrosine hydroxylase and prevents the conversion of tyrosine to l-DOPA The precursor for the two major catecholamine neurotransmitters (dopamine and norepinephrine) is tyrosine, an essential amino acid that we must obtain from our diet. 1. Tyrosine is modified by the enzyme tyrosine hydroxylase and becomes l-DOPA (L-3,4-dihy-droxyphenylalanine). 2. l-DOPA is then modified through the activity of the enzyme DOPA decarboxylase and becomes dopamine. 3. Finally, the enzyme dopamine β-hydroxylase converts dopamine to norepinephrine. L-DOPA — a drug that acts as a precursor for dopamine to replace the lost neurotransmission in Parkinson’s Disease. The drug l-DOPA causes dopamine to be released by surviving dopaminergic neurons in patients with Parkinson’s disease, thus alleviating their symptoms. The drug reserpine prevents the storage of monoamines in synaptic vesicles by blocking the vesicle monoamine transporters. Because the synaptic vesicles remain empty, no neurotransmitter is released when an action potential reaches the terminal button. Reserpine, then, is a monoamine antagonist. Metabotropic receptor ○ 𝐷1 — most common; ○ stimulation of it increases the production of the second messenger cyclic AMP — most common; 𝐷2 ○ decreases the production of cyclic AMP — decreases the 𝐷3 ○ production of cyclic AMP 𝐷4 — decreases the ○ production of cyclic AMP — stimulation of it 𝐷5 increases the production of the second messenger cyclic AMP Dopamine has been implicated as one neurotransmitter that might be involved in schizophrenia, a serious mental disorder whose symptoms include hallucinations, delusions, and disruption of thought processes. NOREPINEPHRINE (NE) Is found in the brain & the sympathetic division of ANS. Precursor: dopamine Enzyme: dopamine β-hydroxylase Chlorpromazine adrenaline = epinephrine noradrenaline = norepinephrine — 𝐷2 receptor antagonist → Treats positive symptoms of schizophrenia Apomorphine — 𝐷2 antagonist Presynaptic autoreceptors have a high affinity for apomorphine; even at low doses many are activated; acts as an antagonist. At high doses, apomorphine binds with postsynaptic receptors; and acts as an agonist. Dopamine transporters — responsible for removing dopamine from the synapse Locus coeruleus — it is where the cell bodies of the most important noradrenergic system begin Fusaric acid — blocks NE production without affecting dopamine Axonal Varicosities — enlarged region along the length of an axon that contains synaptic vesicles. It is where most NE is released. Adrenergic receptors — sensitive to both norepinephrine and epinephrine; metabotropic, coupled to G proteins that control the production of second messengers ○ α1and α2-adrenergic receptors ○ β1and β2-adrenergic receptors Several drugs inhibit the reuptake of dopamine, thus serving as potent dopamine agonists. The best-known of these drugs are Methylphenidate — which blocks DA reuptake → Stimulant used to treat ADHD Cocaine — which blocks DA → reuptake Stimulant and reinforcing effects Amphetamine Methamphetamine Idazoxan — blocks presynaptic noradrenergic α2 receptors → facilitates the synthesis and release of NE Monoamine oxidase (MAO) — an enzyme that regulates the destruction of catecholamines Norepinephrine transporter — responsible for removing excess norepinephrine from the synapse Deprenyl — inhibits MAO-B, dopamine agonist, and treat the symptoms of Parkinson’s disease MAO-A — deactivates NE Moclobemide — blocks activity of MAO-A → Acts as NE agonist SEROTONIN (5-HT, or 5-hydroxytryptamine) Plays a role in the regulation of mood, control of eating, sleep, arousal, and regulation of pain. It is involved somehow in the control of dreaming. It is found mainly in the raphe nuclei. Like norepinephrine, 5-HT is released from varicosities rather than terminal buttons. The two most important clusters of serotonergic cell bodies are found in the dorsal and medial raphe nuclei. The word raphe means “seam” or “crease” and refers to the fact that most of the raphe nuclei are found at or near the midline “seam” of the brain stem. Precursor: tryptophan Enzyme: tryptophan hydroxylase ○ The enzyme tryptophan hydroxylase acts on tryptophan, producing 5-HTP (5-hydroxytryptophan). The enzyme 5-HTP decarboxylase converts 5-HTP to 5-HT (serotonin). PCPA — blocks tryptophan hydroxylase → 5HT antagonist Nine different types of 5HT receptors have been identified 5𝐻𝑇1𝐵 & 5𝐻𝑇1𝐷 — presynaptic autoreceptors 5𝐻𝑇1𝐴 — autoreceptors in the raphe nuclei 5𝐻𝑇3 — only ionotropic receptor; controls Cl- channel ○ Play a role in nausea & vomiting 5𝐻𝑇3 ○ Ondansetron — antagonist that reduces side effects of chemotherapy & radiation Serotonin transporter — responsible for removing 5-HT from the synapse Drugs that inhibit the reuptake of serotonin have found a very important place in the treatment of mental illness. Fluoxetine (Prozac) — SSRI; treat depression, some forms of anxiety disorders, and obsessive-compulsive disorder Fenfluramine — causes 5HT release and inhibits its reuptake → Appetite suppressant MDMA (methylenedioxymethampheta mine or ecstasy) — causes NE & 5HT release & inhibits their reuptake → Excitatory and hallucinogenic effects HISTAMINE cell bodies found in tuberomammillary nucleus of posterior hypothalamus. Send axons to widespread regions of the cerebral cortex and brainstem. It plays an important role in wakefulness. Precursor: histidine Enzyme: histidine decarboxylase Diphenhydramine — crosses the blood-brain barrier to produce drowsiness; blocks histamine receptors → Sedation Peptides These are two or more amino acids linked with a peptide bond. Peptides are released from all parts of the terminal buttons. Once released, peptides are destroyed by enzymes. There is no mechanism for the reuptake and recycling of peptides. Although most peptides appear to serve as neuromodulators, some act as neurotransmitters. Endogenous opioids — are one of the best-known families of peptides Research has revealed that opiates (drugs such as opium, morphine, heroin, and oxycodone) reduce pain because they have direct effects on the brain. Precursors are large polypeptides that are broken into smaller neurotransmitter molecules by special enzymes. Because the synthesis of peptides takes place in the soma, vesicles containing these chemicals must be delivered to the terminal buttons by axoplasmic transport. Although opiate drugs like opium have been used for centuries, receptors for opiate drugs were not discovered until the 1970s. Enkephalins are the natural ligands of opiate receptors. are only two members of a family of endogenous opioids, all of which are synthesized from one of three large peptides that serve as precursors. Three different receptors μ (mu) δ (delta) Κ (kappa) types of opiate So far, pharmacologists have developed only two types of drugs that affect neural communication utilizing opioids: Direct agonists Direct antagonists Many synthetic opiates, including heroin, methadone, and oxycodone, have been developed, and some are used clinically as analgesics. Several opiate receptor antagonists have also been developed. One of them, naloxone (Narcan), is used clinically to reverse opiate intoxication or overdose. This drug has saved the lives of many people who would otherwise have died from an opiate overdose. Lipids are not stored in synaptic vesicles stimulated by THC (tetrahydrocannabinol, the active → ingredient of marijuana) produces analgesia, sedation, and appetite stimulation ○ Asthma ○ Glaucoma ○ Certain motor disorders THC can occur naturally (produced by the marijuana plant) or it can be manufactured synthetically (in drugs such as “Spice” or “K2” as a street drug, or dronabinol as a prescription drug). The best known, and probably the most important lipid, are the endocannabinoids— natural ligands for the receptors that are responsible for the physiological effects of the active ingredient in marijuana. Devane et al. (1992) discovered the first natural ligand for the THC receptor: a lipid-like substance that they named anandamide, from the Sanskrit word ananda, or “bliss.” Lipid neurotransmitters, such as anandamide, appear to be synthesized on demand; that is, they are produced and released as needed and are not stored in synaptic vesicles. Anandamide is deactivated by the enzyme FAAH (fatty acid amide hydrolase), which is present in anandamide-secreting neurons. Recently, investigators have discovered that neurons use at least two simple, soluble gases—nitric oxide and carbon monoxide—to communicate with one another. Drug Effects on Synaptic Transmission Section review pages 111 115 131