NUR 201 Patho Final Study Guide PDF
Document Details
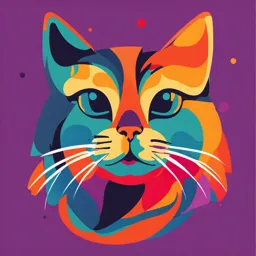
Uploaded by AuthenticBerkelium51
Hofstra University
Tags
Summary
This document is a study guide for a nursing pathophysiology course, focusing on acute myocardial infarction (MI). It details the causes of MI, including coronary artery disease and plaque rupture, leading to myocardial ischemia and necrosis. The document also discusses the location of different types of MIs, their signs, ECG changes, and pathophysiology.
Full Transcript
NUR 201 Patho Final Patho of Acute MI (different coronary arteries) and effect Underlying Cause (FORMATIVE) o Usually begins with coronary artery disease (CAD), characterized by atherosclerotic plaque formation in coronary arteries o Plaque = cholesterol,...
NUR 201 Patho Final Patho of Acute MI (different coronary arteries) and effect Underlying Cause (FORMATIVE) o Usually begins with coronary artery disease (CAD), characterized by atherosclerotic plaque formation in coronary arteries o Plaque = cholesterol, inflammatory cells, connective tissue within arterial walls o Over time may rupture or erode contents into the bloodstream o Plaque rupture triggers FORMATIVE ▪ Activate platelets ▪ Release Tissue Factor (Factor III) + VIIa starts extrinisic pathway → help convert X to Xa (common pathway) → II to IIa → I to Ia (fibrin) ▪ Fibrin cross-links (XIIIa) the platelet plug leading to formation of thrombus (partial or complete obstruction) o Thrombus leads to myocardial ischemia ▪ Reduced or absent blood flow deprives myocardial tissue of oxygen and nutrients ▪ Ischemia affects the subendocardial layer, most susceptible to high oxygen demand and furthest from the blood supply o Myocardial Necrosis ▪ If ischemia >20-30 minutes, irreversible injury occurs ▪ Myocyte necrosis releases intracellular contents (troponin, CK-MB) into bloodstream o Inflammatory response ▪ Necrotic myocardial cells trigger an inflammatory response Leukocytes infiltrate affected areas to remove dead cells, prolonging injury Location Coronary Supplied Areas Signs and ECG Changes Pathophysiology Type of Artery Symptoms MI Left Anterior - Anterior wall - Severe - ST-elevation Large infarcts due to LAD Anterior Descending of LV anterior chest in V1–V4 occlusion can cause Wall MI (LAD) - Anterior 2/3 of pain - Q waves anterior wall dysfunction, septum - Dyspnea over time leading to reduced cardiac - Apex of the - Hypotension output and cardiogenic heart - Possible shock. arrhythmias -Bundle of -Inotropes, assistive device (Ventricular d/t His/LBB reentrant -MOA circuits) -VSD rupture Right - Right atrium - Inferior chest - ST-elevation Right ventricular infarction Inferior Coronary and ventricle pain in II, III, aVF impairs preload; SA/AV Wall MI Artery (RCA) - Inferior wall - Bradycardia - ST- nodal ischemia causes (RV MI - Posterior LV - JVD, clear depression in conduction block, and possible) (PDA in right lungs (RV MI) anterior leads inferior MI can reduce dominance) - Nausea, cardiac efficiency. - SA & AV nodes vomiting -Preload dependent -RBB -Phrenic nerve - MOA involvement Left - Lateral wall of - Lateral chest - ST-elevation Infarction in LCx territory Lateral Circumflex LV pain in I, aVL, V5, reduces lateral wall Wall MI (LCx) - Left atrium - Fatigue, V6 contractility, leading to - Posterior LV dyspnea (if LV - ST- diminished ejection (PDA in left affected) depression in fraction and possible silent dominance) inferior leads ischemia. Left Main - Both LAD and - Severe chest - Widespread Massive ischemia due to Extensive Coronary LCx territories pain ST-depression left main occlusion results Anterior Artery (anterior, septal, - Pulmonary - ST-elevation in extensive myocardial MI lateral, posterior edema, in aVR damage, leading to high LV) profound mortality without urgent dyspnea intervention. - Risk of shock Posterior - Posterior 1/3 - Back pain or - ST-elevation Posterior infarctions often Posterior Descending of septum scapular in V7–V9 accompany inferior or Wall MI Artery (PDA) - Posterior walls discomfort - Reciprocal lateral infarcts, depending of ventricles - Symptoms vary depression in on coronary dominance, by dominance V1–V3 causing posterior wall (RCA or LCx) hypokinesis. Cardiogenic shock Pump failure o Can be caused by ▪ Most commonly MI ▪ Others include HF, myocarditis, valve dysfunction, etc. o Reduced cardiac output ▪ Hypotension Low CO → reduced systemic arterial pressure Impaired coronary artery perfusion, worsening ischemia ▪ Reduced organ perfusion Tissue hypoperfusion leads to ischemia and anaerobic metabolism o MODS ▪ Kidneys: Acute kidney injury (AKI) from ischemia. ▪ Brain: Altered mental status due to hypoxia. ▪ Liver: Hepatic dysfunction and impaired metabolism. ▪ GI tract: Ischemia leading to intestinal barrier dysfunction and possible sepsis. Lactate accumulation results in metabolic acidosis o Compensatory Mechanisms ▪ SNS Catecholamine release (Epi/NE) from the adrenal medulla, increases HR and SVR o Tachycardia and vasoconstriction, increasing afterload and myocardial oxygen demand ▪ High demand and low oxygen delivery exacerbates myocardial injury ▪ Vicious cycle: Reduced contractility → decreased CO → worsened ischemia ▪ RAAS Activated due to decreased renal perfusion o Increases SVR via Angiotensin II and retains fluid via aldosterone, leading to pulmonary congestion ▪ Elevated LVEDP leads to backflow of blood into the pulmonary circulation Pulmonary Edema = dyspnea, hypoxemia, and tissue hypoxia ▪ ADH Release High blood osmolarity and low blood volume lead to the release of ADH, conserving water, increasing preload o Exacerbates congestion in failing heart o Symptoms ▪ Hypotension, Tachycardia, Cold, clammy, SOB, AMS, Reduced UO o Hemodynamic Profile ▪ Low: CO, MAP, SvO2 ▪ High: SVR, CVP, PCWP o Treatment ▪ Inotropes, Vasopressors, Mechanical Support (IABP, Impella, ECMO, LVAD), Revascularize (PCI, CABG) ▪ IABP (FORMATIVE) The balloon is inserted and advanced through the arterial circuit (femoral artery and descending aorta), resting just distal to the outflow area of the aortic valve. When the balloon is “up”, afterload increases and promotes left ventricular filling and coronary vessel perfusion. When the balloon deflates (“goes down”) – this deflation is timed with systole – afterload suddenly decreases; forward flow from the left ventricle is promoted (ejection fraction and stroke volume increases). These combined effects – sudden decrease in afterload at the beginning of systole, increase in ejection fraction/stroke volume during systole, and increase in afterload during diastole – augment blood pressure and cardiac output in the failing heart. Cardiac Tamponade (FORMATIVE) Cardiac tamponade is an example of obstructive shock. The echocardiogram in the patient with severe cardiac tamponade would be expected to show o Pericardial effusion o Diastolic collapse of the right-sided chambers of the heart. The pericardial effusion is the etiology for the significant external pressures experienced by the right atrium and ventricle. Under this pressure, the chambers collapse and are not able to fill. This collapse is greatest when the chambers are most relaxed (diastole). Echocardiogram shows significant collapse of the right atria and ventricles (interestingly, the ventricle is more collapsed in this example). In echocardiogram B, the right-sided structures of the heart are enlarged. This would be more likely in pulmonary embolism, not pericardial effusion causing cardiac tamponade. Relationship between CO, SVR, and BP MAP (BP) = CO x SVR o MAP = (SBP + 2 x DBP) / 3 o BP depends on CO and SVR, a change in either can alter BP o Increased CO: Raises BP if SVR remains constant o Decreased CO: Lowers BP if SVR doesn’t compensate CO: Amount of blood heart pumps per minute (L/min) o CO = SV x HR ▪ SV = amount of blood pumped by LV with each beat (mL) Preload o Volume of blood in ventricles at end-diastole o Influenced by venous return o Increased preload = increased SV Afterload o Resistance heart must overcome to eject blood, influenced by SVR o Increased SVR = decreased SV Contractility o Intrinsic strength of the heart's contraction, influenced by the sympathetic nervous system and medications like inotropes. SVR: Resistance offered by systemic blood vessels to blood flow o High SVR: Increases BP if CO remains constant o Low SVR: Decreases BP unless CO increases to compensate Illustrating Relationships o Low CO with High SVR = Cardiogenic Shock ▪ Low or suboptimal stable o High CO with Low SVR = Septic Shock ▪ BP low due to disproportionately low SVR o Low CO with Normal/Low SVR = Heart Failure ▪ BP low o High CO with High SVR = Hypertensive Crisis ▪ BP severely High Frank-Starling Curve X-axis (Preload/EDV) o The amount of stretch in the ventricular muscle fibers at the end of diastole, determined by the volume of blood in the ventricles (end-diastolic volume, EDV). Y-axis (Stroke Volume/CO) o The volume of blood ejected by the ventricles during each contraction Shape o Low preload ▪ Increasing preload results in a proportional increase in stroke volume due to greater fiber stretch. o High preload ▪ The curve flattens as the fibers become overstretched, reducing their ability to generate force. Shifts o Increased Contractility (Sympathetic stimulation or inotropic drugs) ▪ Curve shifts upwards and to the left, SV increases for a given preload o Decreased contractility (MI, Cardiomyopathy, HF) ▪ Curve shifts downwards and to the right, SV reduced for a given preload o Increased Afterload (HTN, Aortic stenosis) ▪ SV decreases at higher afterload for the same preload o Decreased Afterload (Vasodilation, septic shock) ▪ SV increases for a given preload Clinical Significance o Heart Failure ▪ Systolic HF, curve is flattened due to poor contractility ▪ Even at high preload, SV does not improve significantly ▪ Treatment Reduce preload (diuretics) or increase contractility (inotropes) o Volume Overload ▪ Excessive preload (i.e. mitral regurgitation) can move the heart to the flat portion of the curve, reducing stroke volume o Shock State ▪ Hypovolemic Shock, SV and Preload are both low ▪ Need fluids to restore preload and move heart to optimal part of the curve o Strenuous Exercise (FORMATIVE) ▪ Preload increases with venous return and sympathetic stimulation enhances contractility, maximizing SV (Contractility) ▪ This directly proportional relationship, however, is not maintained indefinitely along all pressures. ▪ Eventually, the ability of the heart to maintain optimal contractility with ever- increasing left ventricular end-diastolic volumes is lost (with too much stretch, myocardial filaments within the sarcomeres lose their overlap and are unable to contract). This rationale is the reason for the “downhill” slope of the curve in the right-sided portions. Strenuous Activity vs Diseased Heart (FORMATIVE) o The “strenuous activity” curve reveals that, for equivalent left ventricular end-diastolic pressures (LVEDPs), there is enhanced myocardial contractility in comparison with resting states. ▪ This is likely a product of increased contractility brought about by beta-1- adrenergic agonism in the setting of strenuous activity and physical exertion. ▪ This heart is likely to demonstrate an increased ejection fraction and stroke volume with increased preload and in the context of increased LVEDP. Note that there is always a limit to this property (the curve reveals diminishing returns with excessive LVEDP). o The “diseased heart” curve reveals that, for equivalent LVEDPs, there is diminished myocardial contractility in comparison with resting states. ▪ This is likely the result of damage to the myocardium and its contractility. This heart is likely to demonstrate an inability to optimally increase the ejection fraction and stroke volume with increased preload. Pulmonary HTN vs Systemic HTN FORMATIVE A similarity is that both conditions are sources of increased afterload against which the corresponding anatomy must act in order to maintain cardiac output. o Other similarities are that both may result in low cardiac output, may present with similar symptoms, and are often treated with similar medications (to start). In pulmonary hypertension, the structure of the heart bearing the burden of maintaining cardiac output against the increased afterload in the pulmonary arterial circulation is the right ventricle. In systemic hypertension, the structure of the heart bearing the burden of maintaining cardiac output against the increased afterload in the pulmonary arterial circulation is the left ventricle. This difference is a major contributing factor to the pathophysiologies of these two disease states. o Another difference is that the pharmacologic management for advanced stage pulmonary hypertension may be different (prostaglandin infusions are often used). The mechanism of action for prostaglandin infusion (e.g., epoprostenol (“Flolan®”), Hypertensive Crisis → pulmonary edema. Starling forces R+R Starling Forces o Net movement of fluid between capillaries and surrounding tissues o Net Filtration Pressure (NFP)= (Capillary Hydrostatic Pressure−Interstitial Hydrostatic Pressure) − (Capillary Oncotic Pressure−Interstitial Oncotic Pressure) o Capillary Hydrostatic Pressure (Pc): The pressure exerted by blood on the capillary walls, promoting filtration (fluid movement out of the capillary). o Interstitial Hydrostatic Pressure (Pi): Pressure within the interstitial fluid, opposing filtration. o Capillary Oncotic Pressure (πc): Osmotic pressure exerted by plasma proteins, drawing fluid into the capillaries. o Interstitial Oncotic Pressure (πi): Osmotic pressure of proteins in the interstitial fluid, promoting filtration. Hypertensive Crisis o Severe elevation in blood pressure (systolic > 180 mmHg and/or diastolic >120 mmHg) o Types ▪ Hypertensive Emergency Severe hypertension with end-organ damage (pulmonary edema, encephalopathy, renal failure, acute coronary syndrome). ▪ Hypertensive Urgency Severe hypertension without acute end-organ damage. o Signs and Symptoms ▪ Dyspnea, orthopnea, pink frothy sputum, crackles, anxiety o Can precipitate pulmonary edema ▪ Elevated Pulmonary Capillary Hydrostatic Pressure (Pc) Hypertensive crisis causes acute left ventricular failure due to excessive afterload or myocardial ischemia. Blood backs up into the pulmonary circulation, dramatically increasing pulmonary capillary hydrostatic pressure. The increased Pc overwhelms the oncotic pressure gradient, leading to net filtration of fluid into the pulmonary interstitial. ▪ Reduced Lymphatic Drainage In hypertensive states, elevated pulmonary venous pressures impair lymphatic drainage, exacerbating interstitial fluid accumulation. ▪ Endothelial Damage Severe hypertension may cause capillary endothelial injury, increasing capillary permeability. This enhances protein leakage into the interstitial space, raising interstitial oncotic pressure (πi) and further promoting fluid accumulation. o Treatment ▪ Reduce preload and pulmonary capillary pressure Nitroglycerin and diuretics ▪ Improve contractility Inotropes ▪ Reduce afterload Nipride, Cardene ▪ Oxygen and Ventilatory support Supplemental oxygen, NIPPV, Intubation and mechanical ventilation Starling Forces in Pulmonary Edema During Hypertensive Crisis Force Effect in Hypertensive Crisis Capillary Hydrostatic Pressure (Pc) ↑ Increased due to elevated pulmonary venous pressure. Interstitial Hydrostatic Pressure (Pi) Slightly increased but insufficient to counteract filtration. Capillary Oncotic Pressure (πc) Normal or slightly reduced due to fluid dilution. Interstitial Oncotic Pressure (πi) ↑ Increased if endothelial damage allows protein leakage. Systolic vs Diastolic Heart failure (FORMATIVE) Categorized based on whether the primary issue: o Systolic Dysfunction ▪ Heart Failure with Reduced Ejection Fraction (HFrEF) ▪ Mechanism Decreased myocardial contractility reduces stroke volume and cardiac output. ▪ Causes CAD Dilated Cardiomyopathy Aortic or mitral regurgitation ▪ Compensation Activation of RAAS: Increases preload and afterload. Sympathetic nervous system activation: Increases heart rate and contractility initially but worsens over time. ▪ Result Ventricular dilation (eccentric remodeling) to accommodate more blood ▪ Clinical Presentation More pronounced fatigue and reduced exercise tolerance due to decreased cardiac output EF < 40 %, chamber dilation S3 Heart sounds (Ventricular Gallop) o Right after S2, early in diastole o Overly compliant, dilated LV ▪ Medications Improve contractility, reduce afterload, and manage volume overload o ACE/ARBs, Beta blockers, Vasodilators, Aldosterone antagonists, Diuretics, Inotropes o Diastolic Dysfunction ▪ Heart Failure with Preserved Ejection Fraction (HFpEF) ▪ Mechanism Reduced ventricular compliance impairs filling, leading to higher end- diastolic pressures. ▪ Causes Hypertension with LVH Pressure overload (Aortic Stenosis, HTN, Hypertrophic cardiomyopathy) Aging (stiff myocardium) Restrictive disease (amyloidosis) ▪ Compensation Elevated left atrial pressure to maintain ventricular filling. Often associated with systemic hypertension and left ventricular hypertrophy. ▪ Result Normal ventricular volume but increased stiffness (concentric remodeling). ▪ Clinical Presentation Exertional dyspnea and pulmonary congestion, even at rest, due to high filling pressures EF >50%, thickened walls, elevated diastolic pressures S4 heart sounds (Atrial gallops) o End of diastole, before S1 o Blood forced into non-compliant LV, stiffened ventricle ▪ Medications Manage comorbidities (a fib, CAD), BP control, diuretics, aldosterone antagonist Similarity (FORMATIVE) o One similarity of systolic and diastolic heart failure is that, in extreme cases, patients will be hypotensive. Differences (FORMATIVE) o One difference between systolic and diastolic heart failure is found in management. o Although systolic failure may cause hypotension, it is often experienced in the setting of hypertension; treatment requires afterload reduction (vasodilators, diuretics, etc.). o In diastolic heart failure, therapies are important to increase preload and left ventricular filling (e.g., fluid administration, beta-adrenergic receptor antagonists, etc.) LVH – Concentric vs Eccentric (FORMATIVE) Concentric Hypertrophy o Cause: sustained pressure overload (hypertension, aortic stenosis) o Response: LV compensates by adding sarcomeres in parallel ▪ Increased wall thickness ▪ Reduces wall stress (Laplace Law: Wall stress = Pressure x radius / 2 x wall thickness) o Result: Chamber becomes stiff leading to reduced compliance and diastolic dysfunction (poor filling) o Pressure overload → Concentric LVH → thickened LV walls → diastolic dysfunction → HFpEF Eccentric Hypertrophy o Cause: Sustained volume overload (valvular regurgitation, high output states) o Response: LV compensates by adding sarcomeres in series ▪ Increases chamber size and ventricular radius ▪ Preserves SV temporarily, despite higher preload o Result: Heart becomes dilated and weak, leading to progressive systolic dysfunction (impaired ejection) o Volume overload → Eccentric LVH → LV dilation → systolic dysfunction → HFrEF Pressure-Volume (PV) loop FORMATIVE R+R The pressure-volume loop → physiology of the left ventricle throughout the cardiac cycle. A graphical representation of the relationship between ventricular pressure and ventricular volume during one complete cardiac cycle o A: Relaxation (Diastole) ▪ Diastolic filling of the left ventricle (preload) → Left ventricular volume ↑ → move from “4” (mitral valve opening) → “1” (mitral valve closing). o B: Isovolumetric contraction (systole) ▪ Tension of the left ventricle ↑ Left ventricular pressure ↑ dramatically ▪ No change in volume o C: Systole ▪ Opening of aortic valve → blood is ejected out of the left ventricle. Occurs when left intraventricular pressure exceeds afterload. ▪ Left ventricular volume rapidly ↓ ▪ Pressure initially spikes, then lowers as blood is ejected. ▪ When afterload exceeds end-systolic left ventricular pressure, the aortic valve closes and diastole begins. o D: Isovolumic relaxation (Diastole) ▪ Left ventricular myocardium relaxes for diastolic filling → left ventricular pressure rapidly ↓ How Aortic Stenosis affects PV loop: Afterload, SV, EDV, CO (FORMATIVE) R+R Aortic Stenosis is characterized by the narrowing of the aortic valve opening, obstructing blood flow from LV to the aorta during systole Increases workload on the heart leading to physiological changes over time PV Loop o Increased Afterload: The stiffened, narrowed aortic valve creates an obstruction, increasing left ventricular (LV) pressure during systole. This elevated pressure represents an increase in afterload. o Decreased SV: With increased afterload, the left ventricle has a harder time ejecting blood. Consequently, SV (volume of blood ejected per beat) decreases. o Increased EDV (Initially): To compensate for decreased SV, preload increases. This results in a higher EDV, as more blood remains in the ventricle to be pumped in subsequent cycles. Over time, chronic AS can lead to left ventricular hypertrophy (LVH), which reduces ventricular compliance and limits further increases in EDV. o Decreased CO: Despite compensatory mechanisms like increased preload, CO (SV × heart rate) often falls in severe AS due to reduced SV and impaired cardiac efficiency. Visual Characteristics o Higher Peak Pressure: Due to increased afterload. o Narrower Loop Width: Indicates decreased SV. o Potential Rightward Shift: Reflects increased EDV in early stages. o Reduced area under the loop: Decreased CO How Mitral Stenosis affects PV loop: Afterload, SV, EDV, CO R+R Mitral Stenosis is characterized by narrowing of the mitral valve, obstructing blood flow from the left atrium to the left ventricle during diastole Results in increased pressure in the left atrium and pulmonary circulation, decreasing filling of the LV PV Loop o Reduced Preload: Mitral stenosis impairs blood flow from the left atrium (LA) to the left ventricle (LV), leading to decreased EDV. This is because the stenotic valve restricts the filling of the LV during diastole. o Decreased SV: With less blood entering the LV (due to reduced preload), the amount ejected during systole is also reduced. o Afterload Remains Normal: Mitral stenosis primarily affects diastolic filling and does not directly increase afterload (resistance against LV ejection). o Decreased CO: Since CO is the product of SV and heart rate, the reduced SV directly leads to lower cardiac output. Visual Characteristics o Reduced Preload (EDV): Lower filling volume. o Narrower SV: Stroke volume is reduced. o Afterload unchanged (in most cases). o Lower CO: Reflecting the diminished ability of the LV to pump sufficient blood. Nodal Cardiac myocyte action potential cycle. All phases and directional flow of ions (FORMATIVE) R+R Nodal cells → Pacemaker cells → SA and AV nodes → unique action potential phases → automaticity SA Node: initiates the impulse → Atrial Depolarization: Impulse spreads across to left atria via Bachman bundle → AV Node → AV Node Delay: ~0.1 seconds delay to allow ventricular filling (fewer and smaller gap junctions) → Bundle of His → Right & left Bundle Branches → Purkinje Fibers: Ventricular depolarization Nodal Action Potential o Phase 4 (Slow Spontaneous Depolarization) ▪ Start @ -70mV → resting membrane potential (RMP) ▪ Funny sodium channels open → slow influx of Na⁺ into the cell → ++ until –40mV (threshold) ▪ Also, T-type calcium channels open → slow Ca²⁺ influx → ++ until -40mV (threshold) ▪ This phase accounts for the pacemaker cells' ability to depolarize spontaneously, and the slow rise in membrane potential is what makes the SA and AV nodes automatic. o Phase 0 (Depolarization) ▪ @ threshold -40 mV → L-type calcium channels open → fast Ca²⁺ influx → +++ (rapid depolarization) → until ~ 0mV - +10mV ▪ This phase is responsible for the upstroke (depolarization) of the action potential in nodal cells. o Phase 3 (Repolarization) ▪ @ ~ 0mV - +10mV → L-type calcium channels close & voltage gated potassium channels open → K⁺ exits the cell → outflow of potassium repolarizes the cell back to a negative membrane potential. ▪ Repolarization is crucial for resetting the cell so it can initiate another action potential. o Phases 1 and 2 are absent in Nodal cells o Entire AP = P wave on ECG Non-nodal Cardiac myocyte action potential cycle. All phases and directional flow of ions R+R Cardiac Myocytes = muscle cells of the heart → make up atria and ventricles → form myocardium o Responsible for contraction Resting membrane potential = -90mV → maintained by Na⁺/K⁺-ATPase Depolarization is initiated by ionic current from Na⁺ and Ca²⁺ leaking in via gap junctions until Threshold potential (~ -70 mV) is met = starts phase 0 Myocyte Action Potential o Phase 0 (Rapid Depolarization) ▪ Starts at -70mV = Threshold ▪ -70mV → @Voltage-gated Na⁺ channels open → fast influx of Na⁺ → less - membrane potential → depolarization → ends @ 0-+10mV ▪ Corresponds with the QRS complex → ventricular depolarization. o Phase 1 (Initial Repolarization) ▪ @ 0-+10mV → Voltage-gated Na⁺ channels close & K⁺ channels open → some K⁺ flows out of cell → brief and small repolarization (slightly more - membrane potential) ▪ Transitional phase before the plateau. o Phase 2 (Plateau Phase) ▪ L-type calcium channels open (extracellular) → fast influx of Ca²⁺ while K⁺ continues to exit ▪ This balance between calcium influx and potassium efflux → plateau. ▪ Triggers → Calcium-Induced Calcium Release (CIRC) → release of stored calcium from the sarcoplasmic reticulum (SR) ▪ The plateau phase is essential for prolonging the contraction of the myocardium, allowing sufficient time for the heart to pump blood effectively. ▪ This phase correlates with the ST segment on the ECG. o Phase 3 (Rapid Repolarization) ▪ L-type calcium channels close and K⁺ channels remain open → significant outflow of K⁺ → repolarizes the cell to its resting membrane potential → -90mV ▪ This phase is responsible for the T wave → ventricular repolarization. o Phase 4 (Resting Membrane Potential -90mV) ▪ The balance between K⁺ leaving the cell and Na⁺/Ca²⁺ entering is @ equilibrium between inside and outside of cell → maintains the resting membrane potential until the next depolarization. ▪ Maintained by Na⁺/K⁺-ATPase ▪ This phase corresponds to the period between heartbeats → ventricles are at rest Non-nodal – electrolyte derangements and alterations to the curve / ECG manifestation Height of the T wave → rate and magnitude of ventricular repolarization Potassium (K⁺) Derangements o Hyperkalemia ▪ Alterations to the curve Accelerates repolarization (phase 3) → shortens duration of action potential Makes RMP less negative (closer to the threshold for action potential initiation) o Less available sodium channels activated ▪ ECG Manifestations: Peaked T waves → accelerated repolarization. Shortened QT interval → faster repolarization Flattened P waves or even loss of the P wave in severe cases. Widened QRS complex Sine-wave pattern in extreme cases → leads to ventricular fibrillation. o Hypokalemia: ▪ Alterations to the curve Prolong repolarization (phase 3) → prolong the action potential duration. RMP is more negative (hyperpolarized), making it harder for the myocyte to reach the threshold and initiate an action potential. Increasing the risk of early afterdepolarization. ▪ ECG Manifestations: Flattened T waves U wave (secondary deflection after T wave) Prolonged QT interval → ↑ risk of torsade de pointes Calcium (Ca²⁺) Derangements o Hypercalcemia: ▪ Alterations to the Curve ↓ duration of plateau phase (phase 2) → shortens action potential duration → ↓ refractory period Enhanced calcium-induced calcium is released from the sarcoplasmic reticulum → increasing myocardial contractility. ▪ ECG Manifestations: Shortened QT interval → faster repolarization. Osborn (J) waves (occasionally in severe cases) o Hypocalcemia: ▪ Alterations to the Curve Prolongs plateau phase (phase 2) → delays repolarization → ↑ action potential duration → ↑ refractory period ↓ intracellular Ca²⁺ levels → ↓calcium-induced calcium release → ↓ myocardial contractions → negative inotropy ▪ ECG Manifestations: Prolonged QT interval → ↑ risk of torsade de pointes. Sodium Derangements o Hypernatremia: ▪ Alterations to the Curve Slightly speeds up depolarization (phase 0) → may shorten action potential duration. Na⁺ conductance is more influenced by the availability of Na⁺ channels than extracellular Na⁺ concentration ▪ Effects on ECG Subtle ECG changes, as sodium primarily affects depolarization: ▪ Possible Features: Mild ↑ in QRS amplitude. Rarely, ↑conduction velocity may shorten the PR interval slightly. o Hyponatremia: ▪ Alterations to the Curve Slightly slows depolarization (phase 0) → may increase action potential duration. Can ↓ overall cell excitability → bradyarrhythmia in severe hyponatremia. ▪ Effects on ECG Subtle ECG changes, as sodium primarily affects depolarization ▪ Possible Features: Prolonged PR intervals (due to slowed conduction). Flattened or broad QRS complex in severe cases. Secondary arrhythmias (atrial fibrillation or ventricular ectopy) due to associated electrolyte disturbances like hypokalemia or hypocalcemia. Magnesium (Mg²⁺) Derangements o Hypermagnesemia: generally, slows down conduction but rarely affects ECG unless levels are severely elevated. ▪ ECG Manifestations (in severe cases): Prolonged PR interval and widened QRS complex due to slowed conduction. o Hypomagnesemia: affects potassium handling and promotes early afterdepolarization. ▪ ECG Manifestations like hypokalemia Prolonged QT interval → ↑ risk of torsade de pointes U-wave Absolute vs Relative Refractory period Refractory Period is the time during an action potential when a cell is either completely or partially unresponsive to a new stimulus. o In non-nodal myocytes ▪ Due to the state of the voltage-gated Na⁺ channels, which cycle through resting, activated, and inactivated states during an action potential. ▪ Voltage-gated Na⁺ channels recovered = active = can depolarize ▪ Cardiomyocytes have a longer refractory period than other muscle cells given the long plateau from slow L-type Ca²⁺ channels (phase 2) ▪ Physiological mechanism allowing sufficient time for the ventricles to contract, empty, and refill before the next contraction ▪ Prevents tetanic contractions o In nodal cells ▪ Determined by recovery of L-type Ca²⁺ channels from their inactive state ▪ Nodal cells have a shorter refractory period than cardiac myocytes because they lack the plateau phase (Phase 2) seen in contractile cells. ▪ This shorter refractory period allows for rapid automaticity to maintain the heart rate. Myocytes ▪ Absolute refractory period (ARP): cell is completely unexcitable to a new stimulus. Phase 0, 1, & 2 o Fast Na⁺ channels are inactivated and cannot reopen until the membrane repolarizes sufficiently (to about -50 mV) ▪ Effective refractory period (ERP): stimulus may cause the cell to depolarize minimally but will not propagate to neighboring cells. Phase 0, 1, 2, and a short segment of phase 3 (ARP + short seg of phase 3). ▪ Relative refractory period (RRP): Greater than normal stimulus will depolarize the cell and cause an action potential Phase 3 o Some Na⁺ channels have recovered = active ▪ Subnormal Period: a hyperexcitable period where even a weak stimulus can cause an action potential Final part of Phase 3 and early Phase 4. Nodal Cells o Absolute Refractory Period (ARP): ▪ The cell is completely unexcitable to a new stimulus because L-type Ca²⁺ channels are inactivated. Phase 0 and most of Phase 3 Prevents overlapping depolarizations, ensuring proper pacing of the heart. o Effective Refractory Period (ERP): ▪ A phase where a strong stimulus might cause local depolarization but will not propagate to neighboring cells because the calcium channels have not recovered sufficiently. Phase 0, Phase 3, and later part of Phase 3 (ARP + later part of Phase 3) Prevents conduction to surrounding cells, maintaining nodal integrity. o Relative Refractory Period (RRP): ▪ A stronger-than-normal stimulus can depolarize the cell and generate an action potential. Late Phase 3 L-type Ca²⁺ channels begin to recover. Increased risk of aberrant firing or reentrant arrhythmias during this period. o Subnormal Period (SNP): ▪ Occurs in early Phase 4 Significance of absolute refractory period Function Significance Prevention of Tetanus Ensures the heart relaxes after each contraction, preventing sustained contraction. Protection Against Arrhythmias Limits abnormal electrical signals from initiating arrhythmias. Regulation of Heart Rate Controls the minimum time between beats, helping to regulate heart rate. Preservation of Coordinated Maintains the sequential and coordinated spread of electrical Conduction impulses. Prevention of Ectopic Beats and Avoids repetitive electrical loops or random stimulation of Re-entry cardiac cells. Facilitation of Blood Filling and Allows adequate time for heart chambers to fill and pump blood Ejection effectively. Action potential cycle nerve vs cardiac myocyte vs skeletal muscle R+R Nerve Cell o RMP: ~ -70 mV o Depolarization: ▪ Voltage-gated Na⁺ channels open → rapid Na⁺ influx. ▪ Membrane potential rises to ~+30 mV. o Repolarization: ▪ Na⁺ channels close; voltage-gated K⁺ channels open. ▪ K⁺ efflux restores negative potential. o Hyperpolarization: ▪ Excess K⁺ efflux causes a slight overshoot of negativity before stabilization. o Duration: 1-2 ms o Refractory: Short (allows rapid, repetitive firing) o Lacks a plateau phase o Propagates via saltatory (myelinated fibers) or continuous conduction Cardiac Myocyte o RMP: ~ -90 mV (Phase 4) o Phase 0 (Rapid Depolarization): ▪ Voltage-gated Na⁺ channels open → rapid Na⁺ influx. ▪ Membrane potential rises to ~+20 mV. o Phase 1 (Early Repolarization): ▪ Brief K⁺ efflux through transient outward channels. o Phase 2 (Plateau): ▪ Ca²⁺ influx via L-type calcium channels balances K⁺ efflux. ▪ Maintains sustained depolarization. o Phase 3 (Repolarization): ▪ Ca²⁺ channels close. ▪ K⁺ efflux restores resting potential. o Duration: 200-400 ms o Refractory: Long (prevents tetany and ensures rhythmic beats) o Plateau Phase 2 o Propagates via gap junctions (syncytium) in intercalated discs. Skeletal Muscle o RMP: ~ -85 mV o Depolarization: ▪ Voltage-gated Na⁺ channels open → rapid Na⁺ influx. ▪ Membrane potential rises to ~+30 mV. o Repolarization: ▪ Na⁺ channels close; K⁺ efflux restores the resting potential. o Duration: 2-5 ms o Refractory: Short (allows rapid contractions) o Lacks a plateau phase o Propogates along the sarcolemma and into the T-tubules Advantages for Cardiac Muscle to be longer than Skeletal muscle (FORMATIVE) R+R o The nature and sequence of events (chamber filling, valve opening and closing, chamber contraction) in cardiac physiology require more time. o One advantage of skeletal muscle having an action potential with a shorter duration of action is that skeletal muscle “resets” quickly, allowing for precise fine motor control and rapid activation/deactivation of muscle groups. o Neurons need even shorter refractory periods to reset even faster. R+R Key Concepts for Nerve and Skeletal Muscle Cells o Summation ▪ Temporal A single presynaptic neuron fires multiple action potentials at the same time in quick succession → @ same postsynaptic target → overlapping postsynaptic potentials. ▪ Spatial Multiple presynaptic neurons fire at different locations on the postsynaptic neuron simultaneously, and their postsynaptic potentials (EPSPs or IPSPs) spread across the neuron's membrane and summate to influence the membrane potential at the axon hillock. o Recruitment ▪ Involves activating more motor units to generate a greater force. ▪ Starts with the activation of smaller motor units (low- threshold motor units), then progresses to larger motor units (high-threshold motor units) as more force is required → size principle. Picking up pencil requires little effort (1 motor neuron to a few muscle fibers) Picking up a table requires more effort (100 motor neurons to thousands of muscle fibers) AVRT (Atrioventricular Reentrant Tachycardia) (FORMATIVE) Presence of an accessory pathway (extra conducting tissue) between atria and ventricles → reentrant loop → allow electrical impulses to bypass the normal conduction route (through the AV node) → reentrant circuit Bundle of Kent in Wolff Parkinson White Syndrome Subtypes: (FORMATIVE) o Orthodromic AVRT (90% of cases): ▪ Impulse travels down the AV node (anterograde) and returns up the accessory pathway (retrograde). ▪ ECG: Narrow QRS complexes with retrograde P waves. o Antidromic AVRT (10% of cases): ▪ Impulse travels down the accessory pathway (anterograde) and returns up the AV node (retrograde). ▪ ECG Wide QRS complexes due to preexcitation. Delta wave: slurred, gradual upstroke at the beginning of the QRS complex Wolff Parkinson White (congenital) o NSR: Wolff Parkinson White Pattern ▪ Delta Wave. ▪ Short PR Interval: Impulse bypasses the AV nodal delay. ▪ Wide QRS Complex: Fusion of precited ventricular depolarization (via the accessory pathway) and normal depolarization Wolff Parkinson White Syndrome → tachycardiac episode → orthodromic (90% of the time) or Antidromic o Avoid AV Node Blockers (beta-blockers, calcium channel blockers, digoxin) o Procainamide or Amiodarone: Preferred for antidromic AVRT or atrial fibrillation with WPW to slow accessory pathway conduction Management: o Acute Termination: ▪ Vagal maneuvers: Carotid massage or Valsalva. ▪ If NOT WPW Adenosine: Temporarily blocks AV nodal conduction to terminate orthodromic AVRT. Beta-blockers or calcium channel blockers: To slow AV conduction. o Definitive Treatment: ▪ Catheter ablation of the accessory pathway AVNRT (Atrioventricular Nodal Reentrant Tachycardia) Reentrant tachycardia, confined to AV node itself rather than an accessory pathway. Typically idiopathic, more common in patients with structural heart disease. Arises from the presence of two distinct conduction pathways within or around the AV node: o Fast pathway: Normal conduction pathway with rapid conduction and slow refractory period. o Slow pathway: A slower conduction pathway with a slower conduction velocity and a shorter refractory period. Slow-Fast AVNRT (Typical AVNRT): o Most common form (~90%). o Impulse travels down the slow pathway (anterograde) and up the fast pathway (retrograde) → forming a reentrant loop within the AV node. o ECG: Narrow QRS with retrograde P waves typically hidden in the QRS complex Management: o Acute Termination: ▪ Vagal maneuvers: First-line therapy. ▪ Adenosine: Highly effective at terminating AVNRT by blocking the AV node. ▪ Beta-blockers or calcium channel blockers: To slow AV conduction. o Definitive Treatment: ▪ Catheter ablation: Targeting the slow pathway GERD, risk factors, pathologic complications if untreated Chronic condition characterized by the backflow of stomach contents into the esophagus, causing symptoms like heartburn and regurgitation. This occurs due to dysfunction of the lower esophageal sphincter (LES), esophageal motility issues, or increased gastric acid production. o Pathophysiology ▪ Lower Esophageal Sphincter Dysfunction: The LES normally prevents gastric contents from refluxing into the esophagus. In GERD, the LES may be: o Weak or hypotensive. o Transiently relaxed, often triggered by meals, alcohol, or specific foods. This allows acidic stomach contents to irritate the esophageal lining. ▪ Increased Intra-Abdominal Pressure: Conditions like obesity, pregnancy, and heavy lifting can increase abdominal pressure and promote reflux. ▪ Delayed Gastric Emptying: Slowed gastric emptying can prolong the time for reflux to occur. Common in conditions like gastroparesis. ▪ Esophageal Motility Disorders: Ineffective esophageal peristalsis can impair clearance of refluxed acid, prolonging mucosal exposure. ▪ Hiatal Hernia: A structural defect where part of the stomach moves above the diaphragm. Weakens the LES and increases reflux episodes. OPTIONAL FORMATIVE** R+R Stomach Cells R+R Peptic ulcer disease, risk factors, pathologic complications if untreated Open sores that develop: o Inner lining of the stomach → gastric ulcer ▪ Antrum is the most common location o First part of the small intestine → duodenal ulcer Occurs with aggressive factors > protective mechanism. o Protective Mechanisms ▪ Mucosal barrier: A layer of mucus that protects the stomach lining from acidic gastric contents. ▪ Bicarbonate secretion: Neutralizes acid near the mucosa. ▪ Prostaglandins: ↑ mucus and bicarbonate production and ↑ mucosal blood flow. ▪ Epithelial cell renewal: Regular repair and replacement of damaged cells. ▪ Adequate blood supply: Maintains nutrient delivery and removes damaging agents ▪ Growth Factors and Cytokines o Aggressive Factors (Risk Factors) ▪ H. pylori (usually duodenum ulcer) Disrupts the protective mucosal barrier and increases acid secretion Types o Antral-Predominant Gastritis ▪ H. pylori infection in antrum ➔ Urease production ➔ Ammonia created to neutralize acid around H. pylori ➔ Damage to protective mucus layer ➔ Increased inflammation ▪ Increased inflammation ➔ Increased gastrin production ➔ Increased acid production (hyperacidity) ➔ Extra acid flows into duodenum ➔ Duodenal ulcer formation ▪ Antral-predominant gastritis → ↓ somatostatin → ↑ gastrin → ↑ acid production. o Pangastritis ▪ H. pylori infection spreads throughout the stomach (pangastritis) ➔ Urease production ➔ Ammonia created, damaging protective mucus layer ➔ Increased inflammation and damage to acid-producing cells ▪ Damage to acid-producing cells ➔ Reduced acid production ➔ Less bicarbonate and weakened mucus layer ➔ Stomach lining becomes vulnerable ➔ Gastric ulcer formation ▪ Diffuse gastritis → damage to parietal cells in the corpus → ↓ acid production (hypochlorhydria). ▪ NSAIDs Chronic NSAID use reduces prostaglandins, impairing the protective mucosal lining. o Systemic Effect ▪ NSAID intake ➔ Inhibition of COX-1 enzyme ➔ Reduced prostaglandin production → Decreased mucus and bicarbonate secretion (which weakens the protective mucus layer) → Reduced blood flow to the gastric mucosa (impairing tissue repair) o Direct irritation of gastric lining by NSAIDs + Increased susceptibility of gastric mucosa to stomach acid and pepsin ➔ Damage to epithelial cells and disruption of the mucosal barrier ➔ Inflammation and localized ischemia in the stomach lining ➔ Development of gastric ulcer ▪ Lifestyle Factors Smoking: Impairs mucosal healing and increases acid production. Alcohol: Damages the gastric mucosa and may increase acid secretion. Diet: Spicy foods or high caffeine intake can exacerbate symptoms but are not direct causes. ▪ Stress ▪ Increased Pepsin Digestive enzyme that degrades stomach lining ▪ Hypersecretory States Example: Zollinger-Ellison syndrome (gastrin-secreting tumors) cause excessive gastric acid production ▪ Genetics ▪ Medications Corticosteroids Antiplatelets and anticoagulants Gastric vs Duodenal o Duodenal Ulcer ▪ Located in the proximal duodenum, most often on the anterior wall ▪ More common in younger patients. ▪ Strongly associated with H. pylori infection (95–100%) ▪ Increased gastric acid production. The acid-base environment is characterized by increased gastric acid secretion. ▪ Epigastric pain relieved by eating, as meals buffer the acid. Pain usually occurs 2–5 hours after eating or during the night when there is no food buffer. Weight gain may occur because patients eat to alleviate pain ▪ Carry a higher risk of perforation, particularly on the anterior duodenum ▪ Rarely associated with malignancy. o Gastric Ulcer ▪ Located in the stomach, usually in the antrum ▪ Less prevalent, occurring more in older adults. ▪ Associated with NSAID use and H. pylori infection ▪ The problem is primarily a weakened protective barrier, not excessive acid production. Even normal levels of stomach acid and pepsin can cause significant damage when the mucosal defenses are impaired. ▪ Epigastric pain worsens with eating, as food stimulates acid production Nausea or weight loss due to the pain associated with meals. ▪ More likely to cause bleeding ▪ Greater potential association with gastric cancer, necessitating biopsy during endoscopy. Pathologic Complications of Untreated PUD o Hemorrhage (Bleeding) ▪ The most common complication. ▪ Ulcers erode into blood vessels Presents as hematemesis, melena, or hematochezia (passage of bright red blood or maroon-colored blood → if rapid bleeding). ▪ Can lead to anemia or hypovolemic shock in severe cases. o Perforation ▪ Full-thickness erosion of the ulcer through the stomach or intestinal wall. ▪ Leads to leakage of gastric contents into peritoneal cavity: peritonitis Presents as sudden, severe abdominal pain with peritoneal signs (rigidity, rebound tenderness). ▪ Requires emergency surgery due to risk of peritonitis and sepsis. o Gastric Outlet Obstruction ▪ Chronic inflammation and scarring → narrowing at the pylorus. ▪ Symptoms: nausea, vomiting (often undigested food), bloating, and weight loss. o Penetration ▪ Ulcer erodes into adjacent organs: pancreas, liver, or biliary tree. ▪ May present with refractory pain radiating to the back or other organs. o Malignancy ▪ Rare but long-standing ulcers, especially gastric ulcers, may lead to gastric cancer. ▪ Risk is higher in individuals with H. pylori infection and intestinal metaplasia. Crohn’s vs Ulcerative colitis Crohn's Disease o Involves the entire GI tract with transmural inflammation and skip lesions, often leading to complications like strictures and fistulas. o Area of Involvement can affect any part of the GI tract, from mouth to anus (most common in terminal ileum and colon). o Distribution: Patchy, "skip lesions" (healthy tissue interspersed with diseased tissue) o Depth of Inflammation: Transmural (affects the full thickness of the bowel wall). o Endoscopic Appearance: Cobblestone mucosa, strictures, aphthous ulcers. o Clinical Features ▪ Diarrhea: Often non-bloody (but may be bloody). ▪ Abdominal Pain: Common, typically in the right lower quadrant (RLQ). ▪ Systemic Symptoms: Fatigue, fever, weight loss. ▪ Rectal Bleeding: Less common unless colonic involvement. ▪ Fistulas/Abscesses: Common due to transmural inflammation. ▪ Perianal Disease: Common (e.g., fistulas, fissures) Ulcerative Colitis o Limited to the colon with continuous mucosal inflammation and a higher risk of toxic megacolon but can be cured with surgery. o Area of Involvement: Restricted to the colon and rectum. o Distribution: Continuous inflammation starting from the rectum and extending proximally. o Depth of Inflammation: Mucosal and submucosal layers only. o Endoscopic Appearance: Erythema, friable mucosa, pseudopolyps ▪ Clinical Features ▪ Diarrhea: Frequently bloody diarrhea. ▪ Abdominal Pain: Less common, typically in the left lower quadrant (LLQ). ▪ Systemic Symptoms: Fatigue, fever, weight loss (less prominent than Crohn's). ▪ Rectal Bleeding: Common. ▪ Fistulas/Abscesses: Rare ▪ Perianal diease: Rare Toxic Megacolon (FORMATIVE) o Potential life-threatening complication of any inflammatory condition of the colon. o Most commonly, it is associated with a severe exacerbation of one of the inflammatory bowel disease (ulcerative colitis is more likely than Crohn’s disease) or an infectious colitis (e.g., pseudomembranous colitis secondary to C. difficile). o It is characterized by total or segmental non-obstructive colonic dilatation and systemic toxicity. o Management priorities include ▪ shock management (intravenous fluid rehydration) ▪ electrolyte correction ▪ decompression of the megacolon ▪ broad-spectrum antibiotics ▪ discontinuation of any agents that reduce gastrointestinal motility ▪ assessment for surgical intervention Bilirubin metabolism, jaundice/hyperbilirubinemia, types of hyperbilirubinemia FORMATIVE Metabolism o Production ▪ Heme Breakdown: Heme → Biliverdin → Unconjugated Bilirubin (indirect). Catalyzed by heme oxygenase and biliverdin reductase. ▪ Occurs in the spleen, liver, and bone marrow. o Transport ▪ Unconjugated bilirubin: Insoluble in water. Transported in the bloodstream bound to albumin to the liver. o Hepatic Uptake ▪ Unconjugated bilirubin is taken up by hepatocytes in the liver o Conjugation ▪ Within hepatocytes, bilirubin is conjugated with glucuronic acid by the enzyme UDP-glucuronosyltransferase (UGT), forming conjugated bilirubin (direct). ▪ Conjugation makes bilirubin water-soluble o Excretion ▪ Conjugated bilirubin is excreted into bile and released into the intestines. ▪ In the intestines: Bacterial enzymes convert bilirubin to urobilinogen. Urobilinogen: o Partly reabsorbed and excreted in urine (urobilin gives urine its yellow color). o Partly converted to stercobilin, which is excreted in feces (gives stool its brown color). Jaundice FORMATIVE o Yellow discoloration of the skin, sclera, and mucous membranes caused by elevated bilirubin levels. o Clinical Presentation ▪ Pre-hepatic (Unconjugated hyperbilirubinemia): Hemolytic anemia, splenomegaly, pale stools, dark urine absent. ▪ Hepatic (Mixed hyperbilirubinemia): Symptoms of liver dysfunction (e.g., hepatomegaly, ascites). ▪ Post-hepatic (Conjugated hyperbilirubinemia): Dark urine, pale stools, pruritus, cholestatic symptoms. Hyperbilirubinemia o Normal Bilirubin Levels ▪ Total bilirubin: 0.3–1.2 mg/dL ▪ Unconjugated bilirubin: 0.2–0.8 mg/dL ▪ Conjugated bilirubin: 0.1–0.4 mg/dL o When total bilirubin exceeds 2–3 mg/dL o Types ▪ Unconjugated (indirect) hyperbilirubinemia Liver has not processed bilirubin to be conjugated Typically indicates ↑ RBC breakdown or impaired liver ability to conjugate Causes: o Pre-hepatic → Excess production ▪ Hemolysis: sickle cell disease ▪ Hemolysis → hemoglobin → heme & globin → ↑ biliverdin → ↑ unconjugated bilirubin → liver cannot keep up → Unconjugated hyperbilirubinemia o Intra-Hepatic → Defective Conjugation ▪ Gilbert Syndrome: mild ↓ in UGT activity ▪ Crigler – Najjar Syndrome: severe UGT deficiency Type I (severe): no UGT activity Type II (milder): reduced UGT activity Unconjugated bilirubin builds up in the bloodstream → jaundice Without dark urine or pale stools o Unconjugated bilirubin is not water-soluble ▪ Conjugated (direct) hyperbilirubinemia Accumulation of bilirubin that was already processed by the liver but cannot be excreted properly into the bile. Typically indicates biliary obstruction or liver disease Causes: o Liver disease → Hepatic → Impaired excretion) ▪ Hepatitis ▪ Cirrhosis ▪ Drug-induced liver injury o Biliary Obstruction → Post-Hepatic ▪ Gallstones, strictures, tumor ▪ Primary biliary cholangitis o Impaired Excretion ▪ Dubin-Johnson Syndrome: Defective transport ▪ Mixed Hyperbilirubinemia Severe hepatocellular damage (e.g., hepatitis, cirrhosis). Sepsis-related cholestasis. Cholecystitis vs Cholelithiasis vs Choledocholithiasis vs Cholangitis FORMATIVE (Least to most severe listed) Cholelithiasis o Gallstones in the gallbladder o Stones form from an imbalance in the bile components (cholesterol, bile salts, and lecithin) → Supersaturation and crystallization o Risk Factors ▪ 4 F’s: Female, Forty, Fat, Fertile o Symptoms ▪ Biliary Colic → intermittent pin in RUQ or epigastric (< 6hrs) Pain radiates to the right shoulder or back Triggered by Fatty meals → fat stimulates gallbladder contraction ▪ Nausea and vomiting ▪ There is no hepatocellular damage or biliary obstruction, liver enzymes (AST, ALT, ALP, GGT) remain normal o Treatment ▪ Cholecystectomy (surgical removal of the gallbladder) Cholecystitis o Inflammation/ infection of gallbladder → obstruction in cystic duct by gallstones o Obstruction → Bile stasis → inflammation → Gallbladder wall edema o Symptoms: ▪ Persistent RUQ pain > 6 hrs., ▪ Leukocytosis, Fever, Nausea, Vomiting (Inflammation/infection process) ▪ Murphy’s Sign: RUQ tenderness with inspiratory rest on palpation ▪ ALT/AST: May be mildly elevated in severe inflammation. ▪ ALP/GGT: Typically normal, unless there is secondary involvement of the bile ducts Choledocholithiasis o Gallstones in the common bile duct → obstruction of bile flow through the CBD (Can progress to cholangitis or pancreatitis if untreated) ▪ Symptoms: ▪ Constant and severe RUQ pain ▪ Nausea, vomiting ▪ Jaundice → from Conjugated hyperbilirubinemia ▪ Dark Urine and Pale Stools → Indicative of bile obstruction. ▪ Elevated liver enzymes ALT/AST: Elevated (mild to moderate). o Temporary hepatocellular injury from bile duct obstruction. ALP/GGT: Markedly elevated o Indicates cholestasis and biliary obstruction o Treatment: ▪ Ultrasound → dilated CBD may visualize CBD stone ▪ MRCP → non-invasive gold standard for confirming stones ▪ ERCP → Stone Removal Diagnostic and Therapeutic ▪ Elective cholecystectomy → prevent reoccurrence Cholangitis o Infection of the biliary tree due to biliary OBSTRUCTION (CBD stones, strictures or malignancy) ▪ Choledocholithiasis → bile stasis → bacterial infection → sepsis ▪ Symptoms: Markedly elevated liver enzymes o ALT/AST: Elevated (moderate to high) ▪ hepatocellular injury due to infection and inflammation. o ALP/GGT: Markedly elevated ▪ biliary obstruction and inflammation. Jaundice →Elevated conjugated bilirubin Dark Urine & Pale stools Charcot’s Triad: Fever, Jaundice, RUQ pain Reynold’s Pentad: Charcot’s Triad + Hypotension, AMS ▪ Treatment: Emergent ERCP o Diagnostic and therapeutic o Risk of pancreatitis IV antibiotics: Broad spectrum → cover gram-negative, gram-positive, and anaerobic o Single agent → Piperacillin-Tazobactam (Zosyn) o Combination therapy → Ceftriaxone + Metronidazole MRCP (Magnetic Resonance Cholangiopancreatography) Non-invasive imagining of bile ducts = Diagnostic Liver Lobule FORMATIVE R+R (EXACT Question) Porta Hepatis o (stomach/spleen/pancreas)→ Portal Vein → portal venules → sinusoidal capillaries → central vein → hepatic vein → IVC o (Abdominal aorta → celiac trunk → common hepatic artery → Hepatic Artery Proper → hepatic arterioles → sinusoidal capillaries → central vein → hepatic vein → IVC o Common Bile Duct Bile Ductules Drain bile from hepatocytes Liver Lobule o Structural and functional unit of the liver o Classic liver lobules: hexagons with a central vein at the center → all central veins → hepatic vein → inferior vena cava. o Portal Triads: Found at each corner of the lobule ▪ Hepatic Arteriole: oxygenated blood to the liver. ▪ Portal Venule: nutrient-rich blood from the gastrointestinal tract. ▪ Bile Ductules: Drains bile produced by hepatocytes. o Hepatocyte Plates: ▪ Rows of liver cells (hepatocytes) radiating outward from the central vein. ▪ Responsible for most liver functions → detoxification and bile production. o Sinusoids: ▪ Capillary-like spaces between hepatocyte plates. ▪ Receive mixed blood from the Hepatic Arteriole and Portal Venule ▪ Blood flows from the portal triad → central vein, allowing an exchange of nutrients, toxins, and oxygen with hepatocytes. o Kupffer Cells: ▪ Specialized macrophages are in the sinusoids. ▪ Play a role in immune surveillance and the breakdown of old red blood cells. o Bile Canaliculi: ▪ Small channels between hepatocytes where bile is secreted. ▪ Transport bile to the bile ducts in the portal triad. o Space of Disse ▪ Exchange space between the sinusoids and hepatocytes, facilitating nutrient and waste exchange. o Stellate Cells ▪ Store fat and fat-soluble vitamins (A, D, E, and K), and play a role in liver repair. Functional Zones of the Liver Lobule (Acinus or Rappaport) FORMATIVE o Zone 1 (Periportal Zone): ▪ Closest to the portal triad. ▪ Receives the most oxygenated blood. ▪ Active in oxidative metabolism, gluconeogenesis, and urea synthesis ▪ Most resistant to ischemia ▪ Susceptible to infections (viral hepatitis), autoimmune damage, toxins acting on first pass o Zone 2 (Intermediate Zone): ▪ Located between Zones 1 and 3. ▪ Transitional metabolic activity. o Zone 3 (Centrilobular Zone): ▪ Closest to the central vein. ▪ Receives the least oxygenated blood. ▪ Metabolically significant → glycolysis, lipogenesis, and detoxification (cytochrome P450 enzymes). ▪ Most susceptible to hypoxia/Ischemia, toxin/drug metabolites (via P450), passive congestion (right heart failure). Receives blood flow last → highly vulnerable to ischemia, similar to a "watershed" area → centrilobular necrosis o Cirrhosis: ▪ Chronic damage leads to fibrosis and nodular regeneration, disrupting the lobular structure. o Hepatic Diseases: ▪ Lobular injury is a hallmark of hepatitis, fatty liver disease, and other liver pathologies. Hepatic injuries – cholestatic vs hepatocellular R+R Cholestatic o Injury or obstruction of bile flow ▪ Intrahepatic (in the liver) → dysfunction of the bile ductules ▪ Extrahepatic (biliary obstruction) → Mechanical obstruction 🡪 Gallstones or strictures o Symptoms ▪ Jaundice ▪ Itching (Pruritus → d/t bile salt accumulation) ▪ Pale stools, dark urine ▪ RUQ pain with tenderness o Labs ▪ Elevated ALP 3x greater than the normal limit and elevated GGT Alkaline Phosphatase (ALP) → found in the biliary epithelium o Elevation alone can also be bone, placenta, kidney, etc. pathology Gamma-glutamyl transferase (GGT) → a marker of biliary injury o Confirms elevation of the ALP is due to the hepatic involvement o Sudden rise in both hepatocellular carcinoma ALP > ALT/AST o Minimal or moderate rise in AST/ALT ▪ Hyperbilirubinemia (Conjugated bilirubin) → elevated because of impaired bile excretion o Common Causes ▪ Gallstones or biliary strictures ▪ Primary biliary cholangitis ▪ Drug-induced Cholestasis ▪ Tumors compressing the bile duct Hepatocellular o Injury/Damage to the hepatocytes (Liver Cells) → ALT/AST release from damaged hepatocytes into the bloodstream o Symptoms ▪ Acute or Chronic Liver disease ▪ Fatigue (may or may not) ▪ Jaundice ▪ Nausea ▪ Pain (diffuse or localized to RUQ) o Labs ▪ Elevated ALT → specific for liver injury because found in hepatocytes ▪ Elevated AST → present in other tissue → Muscle heart, kidney Normal ALT, with elevated AST → think muscle injury (CPK) Exception is AST severely elevated in alcoholic liver disease ▪ AST/ ALT ratio is higher than the ALP Usually, >10x the upper limit of normal ▪ ALP may be NORMAL ▪ ALT/AST Ratio > ALP = Hepatocellular o Common Causes ▪ Shocks → hypoperfusion → Shock Liver (Ischemic Hepatitis) ▪ Viral Hepatitis (A, B, C, D, E) ▪ Drug-induced Liver Injury (acetaminophen toxicity) ▪ Autoimmune Hepatitis ▪ Non-Alcoholic Fatty Liver Disease (NAFLD) ▪ Alcoholic Liver Disease ▪ Alpha-1 antitrypsin deficiency Cirrhosis-induced ascites/varices R+R Cirrhosis-induced ascites and varices are complications of advanced liver disease, primarily due to portal hypertension and associated physiological changes o Cirrhosis is the replacement of normal liver tissue with fibrotic tissue, disrupting hepatic blood flow o Portal Hypertension ▪ Increased resistance to blood flow in the liver (due to fibrosis). ▪ Elevated pressure in the portal vein (>10 mmHg). o This abnormal pressure leads to the development of ascites, varices, and other complications. Ascites o Accumulation of fluid in the peritoneal cavity o Pathophysiology ▪ Portal Hypertension: Elevated hydrostatic pressure in the splanchnic circulation pushes fluid into the peritoneal cavity. ▪ Hypoalbuminemia: Reduced hepatic synthesis of albumin lowers oncotic pressure, exacerbating fluid leakage. R+R ▪ Renal Sodium Retention: Portal hypertension causes systemic vasodilation (via nitric oxide), leading to renal hypoperfusion and activation of the renin-angiotensin- aldosterone system (RAAS). Increased aldosterone levels result in sodium and water retention. o Clinical Features ▪ Abdominal distension. ▪ Shifting dullness or fluid wave on physical exam. ▪ Dyspnea (in severe cases due to diaphragmatic compression) o Diagnoses ▪ Physical Exam ▪ Ultrasound ▪ Paracentesis Analyze ascitic fluid for: o Serum-Ascitic Albumin Gradient (SAAG): ▪ SAAG ≥1.1 g/dL: Indicates portal hypertension. ▪ SAAG 250/mm³) suggest spontaneous bacterial peritonitis (SBP). o Infection of ascitic fluid; treated with antibiotics (cefotaxime) and albumin infusion o Treatment ▪ Dietary: Sodium restriction (1000 U/L ▪ Mildly elevated bilirubin. ▪ Normal to slightly elevated ALP & GGT ▪ Elevated lactate if associated with systemic hypoperfusion. ▪ Prolonged prothrombin time or INR due to impaired synthetic function in severe cases. Diagnosis: o Clinical context of hypoperfusion. o Laboratory findings (dramatic transaminase elevation). o Imaging: May show hepatic hypoperfusion or necrosis. Management: o Treat Underlying Cause: ▪ Restore hemodynamic stability (fluids, vasopressors for shock). ▪ Treat the precipitating cause of shock o Supportive Care: ▪ Monitor liver function and manage coagulopathies o Prognosis: ▪ Reversible if perfusion is restored early. ▪ Persistent ischemia → liver failure. Liver Function Metabolic Functions o Carbohydrate Metabolism: ▪ Maintains blood glucose levels via: Glycogenesis: Stores glucose as glycogen. Glycogenolysis: Breaks down glycogen into glucose. Gluconeogenesis: Produces glucose from non-carbohydrate sources (e.g., amino acids, glycerol). o Lipid Metabolism: ▪ Synthesizes and regulates lipids, including: Cholesterol production and regulation. Formation of lipoproteins (e.g., LDL, HDL). Beta-oxidation of fatty acids to generate energy. ▪ Produces bile salts, essential for fat digestion and absorption. o Protein Metabolism: ▪ Synthesizes non-essential amino acids. ▪ Converts ammonia (a toxic byproduct of protein breakdown) into urea (via the urea cycle) for excretion by the kidneys. Synthetic Functions o Coagulation Factors: ▪ Produces most coagulation factors involved in the clotting cascade Factors I (fibrinogen), II (prothrombin), V, VII, IX, X, XI. Protein C, Protein S, and antithrombin (anticoagulant factors). ▪ Vitamin-K-dependent factors II, VII, IX, X o Synthesis of Fibrinolytic Proteins ▪ Plasminogen (for clot breakdown). ▪ Antithrombin (inhibits coagulation by neutralizing thrombin and Factor Xa). o Clearing Activated Clotting Factors ▪ Liver removes activated clotting factors from circulation to prevent excessive clotting o Plasma Proteins: ▪ Synthesizes albumin, which maintains oncotic pressure and transports various substances in the blood. o Bile Production: ▪ Bile, composed of bile salts, cholesterol, and bilirubin, is crucial for digestion and absorption of fats and fat-soluble vitamins (A, D, E, K). Detoxification o Detoxifies drugs and toxins: ▪ Metabolizes substances into inactive, water-soluble forms for excretion (via urine or bile). ▪ Example: Cytochrome P450 enzyme system. Phase I (cytochrome P450 enzymes) o Adds or unmasks functional groups 🡪 polar metabolites that may be active, inactive, or toxic. o Subject to drug interactions (inhibition or induction of CYP450 enzymes). Phase II o Converts lipid-soluble toxins or metabolites into water-soluble forms for excretion. o Conjugates drugs or their metabolites with polar groups via transferase enzymes (UGT, GST) 🡪 inactive and water- soluble compounds. o Primarily detoxifying, with less variability due to genetics or aging compared to Phase I. o Ammonia Detoxification: ▪ Converts ammonia to urea to prevent toxic buildup. o Alcohol Metabolism: ▪ Breaks down ethanol through enzymes like alcohol dehydrogenase and acetaldehyde dehydrogenase. o Hormone Metabolism: ▪ Deactivates hormones like estrogen, insulin, and cortisol. Storage o Glucose: ▪ Stored as glycogen for energy needs. o Vitamins: ▪ Stores fat-soluble vitamins (A, D, E, K) and vitamin B12. o Iron and Copper: ▪ Stores and regulates iron (bound to ferritin) and copper levels. Immune Functions o Kupffer Cells ▪Specialized macrophages in the liver that filter pathogens, dead cells, and debris from the blood. o Acute Phase Proteins ▪ Synthesizes proteins like C-reactive protein (CRP) during inflammation. Hormonal Regulation o Activates thyroid hormones. o Converts vitamin D into its active form (in collaboration with the kidneys). o Regulates glucose homeostasis through interaction with insulin and glucagon. Excretion o Bilirubin Metabolism: ▪ Breaks down hemoglobin into bilirubin, which is excreted in bile. o Cholesterol Excretion: ▪ Excess cholesterol is eliminated through bile. Liver Dysfunction and coagulation times Prolonged Prothrombin Time (PT): o PT measures the extrinsic and common pathways of coagulation, primarily dependent on Factor VII. o In liver disease, PT is often the first marker to become abnormal because Factor VII has the shortest half-life (4–6 hours). o PT is prolonged in: ▪ Cirrhosis. ▪ Acute liver failure. ▪ Vitamin K deficiency (e.g., due to cholestasis or malnutrition). o Normal PT range: ~11–13.5 seconds. Prolonged International Normalized Ratio (INR): o INR standardizes PT across different labs and is used to assess liver synthetic function. o Elevated INR indicates impaired synthesis of coagulation factors. Prolonged Activated Partial Thromboplastin Time (aPTT): o aPTT measures the intrinsic and common pathways of coagulation. o Factors VIII, IX, XI, and XII contribute to this pathway. o aPTT may be prolonged in severe liver dysfunction. Thrombocytopenia: o Reduced production of thrombopoietin (TPO) by the liver → fewer platelets. o Splenomegaly (due to portal hypertension) → platelet sequestration. D-dimer Elevation: o Seen in liver disease due to impaired clearance of fibrin degradation products. Fibrinogen Levels: o Decreased fibrinogen is a marker of severe liver dysfunction but may be preserved in compensated disease. o Hypofibrinogenemia contributes to bleeding tendencies. Key Clinical Implications o Coagulopathy in Liver Disease: ▪ Liver dysfunction leads to a balanced coagulopathy: Decreased procoagulant factors (increased bleeding risk). Reduced anticoagulant factors like Protein C/S and antithrombin (increased clotting risk). ▪ Despite abnormal PT/INR, spontaneous bleeding is rare without additional insults (e.g., trauma, invasive procedures). o Vitamin K Deficiency: ▪ Common in cholestatic liver disease or malnutrition. ▪ Can exacerbate coagulopathy due to impaired synthesis of Vitamin K-dependent factors. o Clinical Presentations: ▪ Easy bruising. ▪ Mucosal bleeding (e.g., epistaxis, gum bleeding). ▪ GI bleeding from varices. ▪ Spontaneous hematomas or hemarthrosis in severe cases. How smoking contributes to the pathogenesis of stroke (FORMATIVE) Nicotine o Nicotine stimulates the release of norepinephrine and epinephrine resulting in elevated blood pressure (BP), systemic vascular resistance (SVR), and peripheral vasoconstriction. Cigarette smoking is also associated with increased levels of LDLs and reduced levels of HDLs. o Increased BP, SVR, peripheral vasoconstriction, and LDL levels result in increased endothelial injury, which promotes inflammation (likely leading to the development of atherosclerosis) and decreased cerebral blood flow. Smoking is also a prothrombotic stimulus: thrombosis and embolism are much more likely in the context of smoking, and these are well known mechanisms by which strokes occur. Finally, sustained uncontrolled chronic hypertension (which smoking contributes to) may have consequences by causing derangements in healthy (physiologic) cerebral autoregulation. Stroke: hemisphere correlation to symptomology Left Hemisphere Stroke o Generally, controls: ▪ Language and speech (dominant in most people). ▪ Logical reasoning and analytical tasks. ▪ Motor and sensory functions of the right side of the body. o Symptoms: ▪ Aphasia (language impairments): Broca’s aphasia: Difficulty speaking but preserved comprehension. Wernicke’s aphasia: Fluent but nonsensical speech with impaired comprehension. Global aphasia: Severe impairment of all language functions. ▪ Right-sided hemiparesis or hemiplegia (weakness or paralysis on the right side). ▪ Right-sided sensory loss. ▪ Right visual field deficits (hemianopia). ▪ Impaired ability to perform mathematical calculations or logic-based tasks (acalculia). ▪ Difficulty in right-left orientation. ▪ Emotional effects: Often associated with depression or heightened emotional awareness due to intact emotional regulation on the right. Right Hemisphere Stroke o Generally, controls: ▪ Spatial and visual-perceptual processing. ▪ Attention and awareness of surroundings. ▪ Motor and sensory functions of the left side of the body. o Symptoms: ▪ Left-sided hemiparesis or hemiplegia (weakness or paralysis on the left side). ▪ Left-sided sensory loss. ▪ Left visual field deficits (hemianopia). ▪ Hemineglect (spatial neglect): Lack of awareness of the left side of the body or environment. ▪ Difficulty with spatial perception and orientation: Trouble with judging distances, depth, or understanding maps. ▪ Impaired insight and judgment: Lack of awareness of stroke-related deficits (anosognosia). ▪ Emotional effects: May include apathy or emotional blunting, less frequently depression. Circle of Willis Specifics o Anterior Cerebral Artery (ACA) ▪ Supplies medial portions of the frontal lobes and superior medial parietal lobes, especially affecting the paracentral lobule, which corresponds to the lower extremities on the homunculus. ▪ Clinical Findings Motor Deficits: Weakness or paraly