Testing & Simulation of Artificial Organs PDF
Document Details
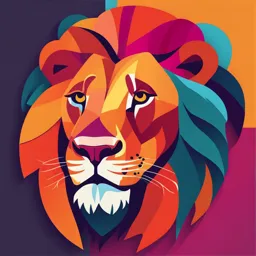
Uploaded by DauntlessLotus
RWTH Aachen University
Tags
Summary
This presentation explores testing and simulation methods for artificial organs, focusing on biocompatibility, hemodynamics, and device-specific parameters. It covers relevant ISO standards, presents various testing approaches, and introduces computational fluid dynamics (CFD) modeling alongside experiment.
Full Transcript
Testing & Simulation Artificial Organs Agenda 1 Testing 1.1 General Aspects 1.2 Biocompatibility 1.3 Hemodynamics 1.4 Device-specific Parameter: Example Blood Pump 2 2 Simulation Testing & Simulation Agenda 1 Testing 1.1 General Aspects 1.2 Biocompatibility 1.3 Hemodynamics 1.4 Device-specific Param...
Testing & Simulation Artificial Organs Agenda 1 Testing 1.1 General Aspects 1.2 Biocompatibility 1.3 Hemodynamics 1.4 Device-specific Parameter: Example Blood Pump 2 2 Simulation Testing & Simulation Agenda 1 Testing 1.1 General Aspects 1.2 Biocompatibility 1.3 Hemodynamics 1.4 Device-specific Parameter: Example Blood Pump 2 3 Simulation Testing & Simulation Medical Device Development Need Design & Prototyping Preclinical Clinical Mechanical strength Functionality 4 Degradation Shelf life Biocompatibility Tissue integration Cytotoxicity … Testing & Simulation Approval Launch Testing – what and how? Thrombogenicity Gas transfer Durability Inflammation … Flow pattern 5 Testing & Simulation Standards & Guidelines ISO: International Organization for Standardization − DIN (German Institute for Standardization) participates as member ASTM International: American Society for Testing and Materials SAC: Standardization Administration of the People’s Republic of China 6 Testing & Simulation Standards & Guidelines General standards: − Biological evaluation of medical devices – ISO 10993, Parts 1-20 − Non-active surgical implants – ISO 14630 −… Device-specific standards − Cardiovascular implants - Cardiac valve prostheses – ISO 5840 − Cardiovascular implants and artificial organs - Blood-gas exchangers (oxygenators) – ISO 7199 − Implants for surgery - Active implantable medical devices – ISO 14708 ▪ Part 5: Circulatory support devices −… 7 Testing & Simulation Agenda 1 Testing 1.1 General Aspects 1.2 Biocompatibility 1.3 Hemodynamics 1.4 Device-specific Parameter: Example Blood Pump 2 8 Simulation Testing & Simulation Definition Biocompatibility is the ability of a material to perform with an appropriate host response in a specific application Mandatory tests depend on − Nature of body contact (e.g. intact skin contact, tissue, bones, blood) − Duration of contact: limited (≤ 24 h), prolonged (24 h – 30 d), permanent (> 30 d) − Existing and transferable material data − Device-specific regulations − Country-specific regulations (ISO ≠ ASTM) 9 Testing & Simulation Biocompatibility Tests (ISO 10993) Cytotoxicity (ISO 10993-5) Sensitization Irritation or intracutaneous reactivity Material-related pyrogenicity Acute systemic, sub-acute, sub-chronic & chronic toxicity Implantation Hemocompatibility (ISO 10993-4) Carcinogenicity Reproductive/developmental cytotoxicity Degradation 10 Testing & Simulation 10993-4: Hemocompatibility Interaction of blood components with artificial material − Hemolysis ▪ Material-induced ▪ Mechanically induced Blood-contacting surface − Thrombogenicity 11 ▪ Coagulation ▪ Platelet activation ▪ Complement activation ▪ Hematology ▪ In-vivo thrombogenicity Testing & Simulation Thrombosis In-vitro Blood constituents Virchow triad Blood flow Hemocompatibility – Hemolysis Destruction of red blood cells → release of cell plasma hemoglobin − Loss of gas transport function − Anemia; organs are undersupplied with oxygen − Triggering coagulation cascade Increasing hemolysis 12 Testing & Simulation Hemocompatibility – In-vitro Thrombogenicity 13 Testing & Simulation In-vitro Thrombogenicity: Test Setup Blood type, volume & species Anticoagulation & analyses Reference circuit/device/material Exposure ratio (3 cm²/mL - 6 cm²/mL) Setup vs test surface area 14 Testing & Simulation In-vitro Thrombogenicity Coagulation Reservoir Flow measurement Platelet activation t1, t2, t3, t4, t5, … Pump (if needed) Complement Sampling port Hematology Test material/device Pressure 15 Testing & Simulation Pressure In-vitro Thrombogenicity: Limitations Test setups not standardized − Device-specific − Lab-specific Analyses not standardized − ISO offers many options to choose → Comparability & reproducibility limited 16 Testing & Simulation Hemocompatibility – In-vivo Thrombogenicity Pre-clinical animal trial − “3R“ principle: Replace, Reduce, Refine − Hemocompatibility values − Implantation procedure & handling − Anatomical fitting − Systemic impact Calf after ReinHeart implantation 17 Testing & Simulation Hemocompatibility – In-vivo Testing TorVAD, Windmill Cardiovascular Systems 18 Testing & Simulation In-vitro vs In-vivo In-vitro tests In-vivo tests + Use of human or animal blood + Physiological conditions + Animal welfare + Complete body interaction + Large screening studies − Transferability to human + Single aspect evaluation − Ethics − Simulated (semi-)physiological conditions − Small study cohort − Limited test duration − Additional influencing parameter 19 Testing & Simulation Agenda 1 Testing 1.1 General Aspects 1.2 Biocompatibility 1.3 Hemodynamics 1.4 Device-specific Parameter: Example Blood Pump 2 20 Simulation Testing & Simulation Hemodynamic Impact High shear rates → hemolysis Regions of stasis & turbulences → thrombosis Malfunction → undersupply of body Heartmate 3, Abbott 21 Testing & Simulation Oxygenator during blood testing Thrombus in mechanical heart valve prosthesis Particle Image Velocimetry (PIV) PIV: flow field visualization + Non-invasive Δt + Flow, shear stress, flow field − Optical access − Blood analogue CVE heart valve thrombogenicity tester (left); PIV results (right) 22 Testing & Simulation Schematic PIV setup Numerical Simulation Numerical calculation of flow fields 23 Testing & Simulation Mock Circulation Loops Simulation of pressure & flow in the circulatory system Combination of − Physiological anatomy − Technical simplification Device testing Training & education CVE pulse duplicator CVE TAH Mock Loop CVE thrombectomy Mock Loop 24 Testing & Simulation Thrombectomy MockLoop Video: Thrombectomy MockLoop 25 Testing & Simulation Durability Video: Heart valve in durability tester 26 Testing & Simulation Agenda 1 Testing 1.1 General Aspects 1.2 Biocompatibility 1.3 Hemodynamics 1.4 Device-specific Parameter: Example Blood Pump 2 27 Simulation Testing & Simulation Device-specific Standards Cardiovascular implants - Cardiac valve prostheses: ISO 5840 Cardiovascular implants and artificial organs - Blood-gas exchangers (oxygenators): ISO 7199 Cardiovascular Implants and extracorporeal systems – Centrifugal blood pumps: ISO 18242 Abbott Regent prosthesis Ebbitides Paragon oxygenator https://www.cardiovascular.abbott/int/en/hcp/products/structural-heart/surgical-valve-solutions/regent-valve.html https://www.ebbtidesmedical.com/paragon-oxygenators/ https://www.getinge.com/de/produkte/rotaflow-zentrifugalpumpe/ 28 Testing & Simulation Getinge Rotaflow blood pump Centrifugal Blood Pump Specifics Biological characteristics − Sterility and non-pyrogenicity − Biocompatibility Physical characteristics − Blood pathway integrity − Prime volume − Connector integrity Performance characteristics − Hydraulic performance − Blood cell damage − Bearing durability − Shelf life 29 Testing & Simulation CentriMag blood pump during application https://tadviser.com/index.php/Product:CentriMag_%28blood_circulation_ support_system%29 Hydraulic performance HQ curve (pressure vs flow) Pump efficiency 30 Testing & Simulation Hydraulic performance 1: water bath 2: reservoir 3: Hoffmann clamp 4: temperature sensor 5: flow sensor 6: pressure sensors 7: pump 8: controller & measurement equipment 31 Testing & Simulation HQ Curve 32 Testing & Simulation Efficiency 𝜂𝑝𝑢𝑚𝑝 𝑃ℎ𝑦𝑑𝑟 𝑉ሶ ∗ Δ𝑝 = = 𝑃𝑒𝑙𝑒𝑐𝑡𝑟 𝑈∗𝐼 η: efficiency P: power 𝑉:ሶ flow 33 p: pressure → measured U: voltage I: current → measured Testing & Simulation Blood Pump Hemolysis Blood pump hemolysis setup 34 Testing & Simulation Test Setup Water bath − Normothermic 37 °C ± 2 °C Static control − Hypothermic 23 °C ± 2 °C Static control Laminar inflow to flow sensor: 15 x dinner Circuit volume Vmax < 500 mL Pre-primed with saline Reference setup with predicate Minimum n = 5 repetitions x2 35 Testing & Simulation Test Fluid: Blood Required volume: 1 L – 5 L depending on setup, number of devices Animal blood (from the abattoir) allowed for testing − Volume > 1.5 L: blood pool from different animals − Blood bottles prepared with heparin (e.g. 15,000 IU/L) Blood arrival − Baseline blood count, ACT & hemolysis samples (initial pfHb < 50 mg/dL) − Fill blood bag via filter, free of air − Adjust base excess to 0 mmol/L ± 5 mmol/L − Drain PBS/saline from circuits 36 Testing & Simulation Blood collection bottle Experiment Preparing test loops − Fill each loop with blood, remove all air − Adjust Hct to 35 % ± 2 % in all circuits (PBS or saline) − Adjust volume in all circuits (e.g. 480 mL ± 15 mL) Static control − Blood bag resting at the same temperature − Same sampling protocol Pre-pumping − 5 min at low flow for mixing − Baseline sample 37 Testing & Simulation Hct ~ 40 % Experiment Experiment runtime − Adjust flow & pressure head according to pump operating point (worst-case scenario testing) − Draw first sample t = 0 min − ASTM: sampling every hour; ISO: 30 min, 180 min, 360 min Sample processing − 1 mL discard from stopcock before sample collection − 2.5 mL sample from all loops and static control − Optional: LDH, vWF, others 38 Testing & Simulation pfHb Analysis HiCN (cyanmethemoglobin) Method − Chemical solution converts Hb to HiCN − Photometric measurement at 540 nm wavelength and 680 nm reference − Calculation of Hb concentration from absorption − Duplicates with CV < 0.1 − Plot pfHb over time − Linear regression with R2 ≥ 0.95 39 Testing & Simulation NIH & MIH Normalized Index of Hemolysis 𝑁𝐼𝐻 = 𝑉 𝑑𝐻𝑏𝑓𝑟𝑒𝑒 ∙ ∙ 1 − 𝐻𝑐𝑡 𝑑𝑡 𝑉ሶ [NIH] = g/(100 L) Modified Index of Hemolysis 𝑉 𝑑𝐻𝑏𝑓𝑟𝑒𝑒 1 − 𝐻𝑐𝑡 𝑀𝐼𝐻 = ∙ ∙ 𝑑𝑡 𝐻𝑏𝑡𝑜𝑡𝑎𝑙 𝑉ሶ V: volume 𝑉:ሶ flow Hb: hemoglobin Hct: hematocrit t: time 40 Testing & Simulation Flow in L/min Testing – Take-home Message Testing is highly important for artificial organs and any medical device In-vitro tests reduce animal trials Tests need to be further developed & standardized Hemocompatibility tests are challenging… …but provide lots of information about blood-material interaction Requirements Validation testing System design Module design Product System testing Verification testing Component Module testing Component testing design Manufacturing 41 Testing & Simulation Agenda 1 Testing 2 Simulation 2.1 Why and What 2.2 Background and One Exemplary Modeling Approach 2.3 Risks and Opportunities 42 Testing & Simulation Agenda 1 Testing 2 Simulation 2.1 Why and What 2.2 Background and One Exemplary Modeling Approach 2.3 Risks and Opportunities 43 Testing & Simulation Why should we model? Biological systems are highly complex ➔ Impossible to understand w/o computers Virtual exploration of many scenarios ➔ Impossible to do in vitro/in vivo Mechanistic understanding of a system ➔ Therapies, devices etc. Regulatory pipeline medical devices 44 Testing & Simulation https://www.fda.gov/medical-devices/device-advice-comprehensive-regulatory-assistance/overview-device-regulation What should we model? τα πάντα ῥεῖ - everything flows 45 Testing & Simulation Agenda 1 Testing 2 Simulation 2.1 Why and What 2.2 Background and One Exemplary Modeling Approach 2.3 Risks and Opportunities 46 Testing & Simulation Background Computational Fluid Dynamics 1. Model flow physics 2. Discretize the geometry (separate in small volumes) 3. Solve the equations for each volume 47 Testing & Simulation 1st step: Modeling ➔ Described via Navier-Stokes-Equations Continuity equation: change of mass = sum of net fluid flows ➔ no mass can be created or destroyed Conservation of momentum: change of momentum = sum of net momentum flows + external forces ➔ external forces: pressure, shear stress, gravity 48 Testing & Simulation 2nd step: Discretization Analytical solutions of N-S equations only for simple geometries Laminar flow in rigid pipe Solve for each small element (cells of mesh) Meshed domain 49 Testing & Simulation 3rd step: Solution v or p v=0 v or p Meshed domain with boundary conditions Set boundary conditions and solve on computer 50 Testing & Simulation Example I : Rotary blood pumps Design of blood pump characterizes flow field ➔ hydraulic output & blood compatibility Use CFD to calculate flow field Use results to increase efficiency & decrease blood damage Heartmate VAD 51 Testing & Simulation Parts of a rotary blood pump Example I: CAD Geometry to mesh 5 l/min ca. 5 mio elements 0 Pa 2500 rpm CAD geometry of an RBP 52 Testing & Simulation Mesh of an RBP Example I: Iteration & results x,y,z momentum continuity time convergence < 1e-4 Convergence plot 53 Testing & Simulation Pressure distribution in RBP Example I: It looks nice, but can we trust it??? ➔ Verification & Validation chapter Simulation Comparison simulation in vitro hydraulic testing Comparison PIV and CFD. Rotational velocity (top), absolute velocity (bottom). Ψ/Φ: Dimensionless numbers pressure/flow 54 Testing & Simulation Example I: CFD based design optimization Homogeneous velocity distribution Stagnation area prone to thrombus formation High velocities at blade outlets Initial and modified design of an RBP and its effect on velocity and shear 55 Testing & Simulation Reduction of shear rates Example I: Parameter based design exploration β1 β2 many variations in β1 and β2 Parameter based design exploration. left: initial design, center: meta-model, right: optimal design 56 Testing & Simulation Agenda 1 Testing 2 Simulation 2.1 Why and What 2.2 Background and One Exemplary Modeling Approach 2.3 Risks and Opportunities 57 Testing & Simulation FDA Round Robin initiative – Let’s test CFD/PIV around the world FDA Round Robin Pump curve/pressure head ➔ hydraulic vs. CFD Velocity distribution ➔ PIV vs. CFD Hemolysis ➔ in-vitro blood vs. CFD 58 Testing & Simulation FDA Round Robin: Velocities Experiment 59 Testing & Simulation Velocity comparison Simulation Recall of CFD theory 1st step: Modeling 2nd step: Discretization 3rd step: Solution 60 Testing & Simulation Errors/assumptions along the pipeline affect our result Modeling error: Turbulence Turbulence in flow: chaotic changes in pressure/velocity Caused by disturbance of the flow field laminar ➔ transitional ➔ turbulent Vortices (eddies) on various spatial scales Big ➔ smaller ➔ smallest ➔ heat Turbulent Free Jet (CFD Calculation) 61 Testing & Simulation Examples of turbulence modeling Effect of turbulence models on jet free flow Sonntag, S. et al. “Annals of Biomedical Engineering 42 (2013): 971-985. 62 Testing & Simulation CFD vs. PIV for various orifice geometries Modeling error: Blood rheology Blood: Suspension of cells (45 %) and plasma (55 %) Thrombocyte η,μ Erythrocyte 𝛾ሶ Human blood cells Dynamic viscosity of blood for various shear rates Newtonian, Non-Newtonian, Newtonian 63 Testing & Simulation Leukocyte Modeling errors: Blood damage – hemolysis and thrombosis Thrombus risk factor Blood Damage = F(σ,t) + Outlet HI = 1.8 10 −6 1.991 dt 0.765 Inlet Hemolysis Model Blood experiment Model of hemolysis Model of thrombosis 64 Testing & Simulation Modeling errors: other examples Many other modelling choices have to capture phenomena like: Blood flow through fibres of an oxygenator Gas transfer (O2 and CO2) Interaction between fluid and vessel wall Interaction between fluid and valves etc. Flow and gas transfer in an oxygenator 65 Testing & Simulation Summary - Modeling errors Many phenomena to consider ➔ many assumptions necessary There is a myriad of approaches to represent these phenomena There is no “best” model ➔ depends on application Keep your assumptions in mind and check for sensitivities! 66 Testing & Simulation Recall of CFD theory 1st step: Modeling ✓ 2nd step: Discretization 3rd step: Solution 67 Testing & Simulation Errors/assumptions along the pipeline affect our result Discretization error: How fine should my mesh be? which one is correct? Meshed domain 3 mesh resolutions for a stenosed artery Jamalabadi, M. Y. Abdollahzadeh et al. PLoS ONE 13 (2018) 68 Testing & Simulation Mesh resolution may affect the entire flow field Unrealistic velocity distribution Development of vortices in wake region Even “simple” numerical setups might be more intriguing than you think Coarse Fine Flow through squeezed tube 69 Testing & Simulation Summary: Meshing errors Mesh strongly influences the results of the simulation Important step in the verification process Know what output is of interest for you Gradient based outputs (shear stresses) need very fine meshes 70 Testing & Simulation Recall of CFD theory 1st step: Modeling ✓ 2nd step: Discretization ✓ 3rd step: Solution 71 Testing & Simulation Errors/assumptions along the pipeline affect our result Solution errors: Equations are solved numerically ➔ Are just approximations!!! Mathematical algorithms to solve the system of equations (PDE) Use convergence plots to see if solution algorithm “works” Not converging? ➔ Go back and adjust “Modeling” and “Discretization” x,y,z momentum continuity convergence < 1e-4 time 72 Testing & Simulation Convergence plot Summary CFD Flow field can be computed numerically by Modeling, Discretization, Iterative solutions Results strongly dependent on boundary conditions Video @ moodle Spatial and temporal resolution (mesh and timestep) turbulence model Model simplifications… If validated: Powerful tool for development Gains importance due to increasing computing power Awareness of modelling limitations & errors crucial for credibility Validation and verification warranted 73 Testing & Simulation A clever citation to end with “All models are wrong, but some are useful”. “Since all models are wrong, the scientist must be alert to what is importantly wrong” Contact for questions or theses projects: [email protected] 74 Testing & Simulation George E. P. Box Thank you for your attention ! Testing Simulation Dr. Johanna Clauser Dr. Michael Neidlin [email protected] [email protected]