IoT Applications - Value Creation for Industry PDF
Document Details
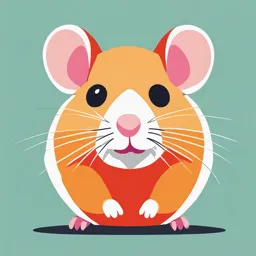
Uploaded by ProactiveSaxophone
2013
Nicolaie L. Fantana, Till Riedel, Jochen Schlick, Stefan Ferber, Jürgen Hupp, Stephen Miles, Florian Michahelles, and Stefan Svensson
Tags
Summary
This paper discusses the value creation for industrial IoT applications. It explores various aspects including challenges, future trends, and specific technologies in industrial environments. The authors present insights from industry, academia and research, focusing on manufacturing, supply chain, and service applications.
Full Transcript
3 IoT Applications — Value Creation for Industry Nicolaie L. Fantana1 , Till Riedel2 , Jochen Schlick3 , Stefan Ferber4 , Jürgen Hupp5 , Stephen Miles6 , Florian Michahelles7 , and Stefan Svensson8 1 ABB CRC, Germany 2 KIT TECO, Germany 3 DFKI, Germany 4...
3 IoT Applications — Value Creation for Industry Nicolaie L. Fantana1 , Till Riedel2 , Jochen Schlick3 , Stefan Ferber4 , Jürgen Hupp5 , Stephen Miles6 , Florian Michahelles7 , and Stefan Svensson8 1 ABB CRC, Germany 2 KIT TECO, Germany 3 DFKI, Germany 4 Bosch Software Innovations, Germany 5 Fraunhofer IIS, Germany 6 MIT, Auto-ID Labs, USA 7 ETH, Auto-ID Labs, Switzerland 8 ABB CRC, Sweden Abstract IoT in industry is a rapidly developing area. Numerous IoT research and application projects have been done by universities or in joint industry- university consortia in recent years. However an important question to be further addressed is on value creation by IoT industry applications. IoT appli- cations in the sense of this paper are solutions using IoT technologies to improve industrial manufacturing processes, enable new and efficient ways to operate production plants, create new service or supervision means for indus- trial installations, offer an optimized infrastructure, reduce operational cost or improve human safety in industrial areas. The present paper brings together Internet of Things: Converging Technologies for Smart Environments and Integrated Ecosystems, 153–206. © 2013 River Publishers. All rights reserved. 154 IoT Applications — Value Creation for Industry experts from academia, research and industry offering a view on the IoT appli- cation in industrial environment, the challenges, expected evolution of IoT technology and use in future factories, on connected and holistic processes. The paper is intended to contribute to an IoT supported paradigm change in manufacturing, industrial service and over life sustainable industrial activities. 3.1 Introduction Internet of things (IoT) has become part of your daily life. The “things con- nected to the internet” idea is continuously evolving in content, areas of appli- cations, visions and technology. New real life and industrial projects have been done and joint future oriented industry and government initiatives such as Industry 4.0 in Germany, have been started. Since Industrial production is one of the world’s biggest economic factors one of the major objectives of these initiatives is to bring the paradigms of the IoT to the factories enabling them to cope with the challenges raised by popular megatrends. The foremost megatrends relevant for factories are globalization, progressing technological evolution, the dynamization of product life cycles, the aging work force and the shortage of resources. Central effects are the acceleration of innovation cycles and the increasing customer demand for individualized mass produces with highest quality expectations. Within the context of industrial production IoT projects and applications are developing in manufacturing, supply chain, supervision and servicing. A major question in all projects is about the value, the benefit such application can bring to the user, to the owner or to society. The value question is extremely pertinent in the industry: in the manufac- turing industry entire factory related processes, but also in industrial applica- tions where it comes to ensure operation of industrial installations and provide supervision, and improved life service. It is the value which such applications bring which will determine their adoption, acceptance and wide use. However, this value is very difficult to quantify and prove, and it depends on multiple aspects which are strongly application area dependent. The present paper is focusing on IoT applications form the point of view of value creation for industry and brings together expert opinions from academia, research and industry. The industrial application of IoT is multi- facetted and each of the subsections in this paper will highlight an aspect related to industrial 3.2 IoT Applications for Industry — Value Creation and Challenges 155 application, discuss or show a case or the evolution and potential of a specific technology from industry application point of view. The paper is having a holistic manner to industrial challenges and requirements. Also it will refer to factory concepts and applications supported by IoT, including processes and flows taking a view on related technologies and their evolution. These types of topics have been also addressed by the authors during an industrial workshop at a recent international conference. At the beginning the paper presents a view form industry regarding IoT applications, the requirements and challenges which have to be overcome or capabilities expected form industrial IoT applications. Subsequent sections discuss items like: future factory concepts and experience in the area, evolution and future of IoT technologies, use of smart objects for creating smart IoT- based applications, technologies inspiring connected life related to industry. A view on the whole chain and flows and information services based on smart objects are presented followed by a shopping basket approach from industry view. Aspects from a real industrial application in the hard environment of oil and gas industry are presented. Some collected opinions on IoT and value aspects, obtained at an industry workshop during the 3rd IoT conference are also shown. 3.2 IoT Applications for Industry — Value Creation and Challenges IoT Applications Throughout the entire document the following pragmatic definition for IoT applications was used in order to focus the scope and to have a common understanding: IoT applications in the sense of this paper are solutions using IoT technologies capable to improve and easy adapt industrial manufacturing processes, enable new and efficient ways to do operate and interact in pro- duction plants, create new service or supervision means for industrial instal- lations, offer an optimized infrastructure, reduce operational cost and energy consumption or improve human safety in industrial areas. Value, Benefit To start a project in industry environment the expected benefit, the expected value to the company has to be estimated and later needs to be re-evaluated and 156 IoT Applications — Value Creation for Industry proved during operation. To define the value of an industrial IoT application or IoT project is difficult. There are numerous reasons for that. The value typically shows up gradually with new process introduction and accumulates over time, the value is often difficult to quantify due to multiple interactions and complex processes, it may contain hard but also soft benefits difficult to assess. Value can be generated and may show up as a result of a combination of IoT applications with other systems or processes, or can originate in new human behavior or new interactions. Fact is that value is the key element finally asked by the project stakeholders or owners. There is agreement that IoT brings benefit in different areas, however num- bers to quantify that value are scarce. More recently CISCO proposed a view called Internet of everything based on IoT and additionally “connecting to internet everything not connected yet”. The global potential, the “value at stake”, for what was called Internet of Everything economy and for the decade 2013–2022, was estimated to $14.4 trillion. Also 5 major drivers have been identified n and 4 of them: asset utilization, productivity, logistics effi- ciency, innovation have strong connections with IoT applications in industry. IoT applications benefit and value creation in an industrial environment may have its origin in different aspects, depending on the application type. There is no value but “values” each contributing to the total benefit such as: Value from visibility identification, location tracking Value form IoT-supported safety in hard industrial environments Value from right information providing or collecting Value form improved industrial operation and flows in industry Value from reduced production losses Value from reduced energy consumption Value from new type of processes made possible by IoT applica- tions Value form new type of maintenance and lifetime approaches Value enabled by smart objects, connected aspects Value from sustainability. The value form visibility was analyzed in a recent 2012 study by Forrester Research. Based on the responses from this study and cumulating the responses “very important to bring value” and “important to bring value” the results are shown in Figure 3.1. The most important IoT technologies perceived 3.2 IoT Applications for Industry — Value Creation and Challenges 157 Fig. 3.1 View on very important and important perceived IoT technologies expected to bring value in applications. by companies, as of 2012 study, are related to barcodes, tracking and mobile computing — the red border area, however RFID and NFC technologies, as well as sensors stand alone or meshed — the blue border area, are important too and show future potential. The status and estimated potential of IoT applications is presented in Figure 3.2 considering three major areas: supply chain, future industry/future factory and over lifetime applications and activities such as logistics, manufac- turing and service/maintenance. A strong potential and additional application is expected in industry operation and industry lifetime applications including lifetime service. IoT applications requirements and capabilities The expectations toward IoT applications in industry are high. The capabilities they have to offer are depending strongly on the industrial area and the concrete application. For example the environment where IoT application may be used may range from clean room condition and normal ambient temperatures to heavy and dirty environment, locations with high temperatures, areas with 158 IoT Applications — Value Creation for Industry Fig. 3.2 Status and estimated potential of IoT applications. explosion risk, areas with metallic surroundings, and corrosive environment on sea or underground. A list of a set of industry related capabilities and requirements is presented below, without claiming completeness. The list items are related to the IoT hardware, software and to serviceability and management aspects. Comments have been added to all items to make the requirement more specific. The IoT application capabilities for industrial application should meet requirements such as: Reliability. Reliable IoT devices and systems should allow a continuous oper- ation of industrial processes and perform on-site activities. Robustness. The IoT application and devices should be robust and adapted to the task and hard working conditions. This should include also the certifications for the specific work environment where they are used. Reasonable cost. Cost aspects are essential and should be fully justifiable and adapted to the benefit. It is basically about the right balance between cost and benefit rather than low cost. Also the costs are related to a more holistic view and life costs and consider the impact on the whole industrial installation in case of a failed IoT device or application. 3.2 IoT Applications for Industry — Value Creation and Challenges 159 Security and safety. Security requirements are related to the cyber security threats and have to be part of the entire security strategy of the company. Safety is mainly related to the device construction and the area of use but also to usability such that no safety threats occur due to use of the IoT applications and devices. Simple use. Simple, intuitive use and (almost) self-explaining are important for the overall IoT application acceptance. The IoT application should ideally be context aware and adapt to the skills of the user and location or environment aspects. Optimal and adaptive set of features. The IoT application should allow to perform desired task with the sufficient, not-richer-then-necessary, set of features Low/No maintenance. Maintenance free or reduced maintenance IoT applications and devices over operational life would be ideal. Maintenance over lifetime is an important aspect impacting the life cycle costs of IoT based solutions. It is affected by the sometimes high number of IoT devices in place, the fact that they are typically distributed over large areas, the required skills, tools and time needed for any type of IoT maintenance operation. This is valid for all devices but especially for active IoT devices or active wireless sensing. Standardization. IoT devices and applications should be using a set of standards to support interoperability of IoT devices, easy exchange and multi- vendor possibilities. Integration capabilities. Easy integration in the IT and automation and process landscape of the industrial plant are required and may decide if a IoT solution will be used. This is particularly important for brown-field projects but also for green field in the view of future plant extensions. Reach sensing and data capabilities. IoT applications will relay more and more on complex sens- ing allowing distributed supervision and data collection and data capabilities. This is a chance in terms of additional data and 160 IoT Applications — Value Creation for Industry real-time information but also a challenge in terms of data and processing. Industry grade support and services. The IoT applications should be supported over years in operation by a set of rich tools and continuously updated services. Typi- cally industry application requires also a centralized management of devices and systems, managed access rights, this might apply to some of IoT devices too. Presently there are also numerous challenges to reach all the above. Challenges faced by IoT industry applications The challenges for IoT industrial applications can be subject of a more extended treatment, however for the needs of present IoT applications and value creation they have been divided in 4 groups: IoT device technical challenges Lifetime and energy challenge Data and information challenge Humans and business The IoT devices technical challenges are numerous and subject of intense research. Some aspects will be addressed also in the following sections. A set of technical features will be especially needed in industrial applications, depending on application, such as extended capabilities for sensing in terms of sensor types and high sampling rate, communication, wireless data transfer and precise time synchronous collection of data both in single-hop and multi- hop industrial networks. Another aspect is related to the easy deployment, configuration and re-use of non-permanently attached devices, such as the ones used for ad-hoc sensing. One critical and often neglected aspect is the device packaging for the industrial application needs which is essential for reliable operation. Last but not least is the heterogeneity aspect which is a problem even today. In industrial environments often encountered are combinations of one or more of: of passive and active RFID with or without sensing, various fix or mobile RFID readers, wireless sensor nodes and networks, wired and wireless technologies in factory automation, use of different frequency bands 3.2 IoT Applications for Industry — Value Creation and Challenges 161 13.5 MHz, 433 MHz, 860–925, 2.4 GHz, use of various “languages” — ISO standards, and different mobile devices and ecosystems. A special challenge related to IoT devices is related to lifetime of the IoT device which is less than of the normal industrial installation. This lifetime mismatch needs to be considered in the complete design and management of industrial installations involving IoT. The energy challenge is also impor- tant, especially for active IoT devices. Depending on application the energy harvesting can be a solution. A very important aspect is the data and information challenge. The IoT devices are important sources of rich and spatial distributed identification, historical and sensor data in industrial environment. With the advent of more intensive use in industry and taking as an example an industrial supervision case the data amounts can really explode. Taking a simple industry sensing and supervision example with 100 sensors installed and collecting sensing data such as: temperature 1 per min, 3axes acceleration data with 10 k sam- ples per second, and 1 audio channel with say 40 ksamples per second, also considering that data is collected only 1% of daytime, approx. 15 min per day, the total amount of raw data for 1 year sums up to 4.4 PB/year. This is only to show that such amounts of raw data need to be processed and condensed and analyzed in order to be usable at all so not data but infor- mation behind it needs to be extracted for industrial use. Adaptive data han- dling and data processing and data fusion methods are required to handle also the industrial IoT data emerging, and will require new methods to visu- alize or inform about the status of real world. Also in industry the IoT data need to be correlated to the already available automation and control data in industrial plants. This blended information will be needed for a specific installation and typically on site too. All this data and information need also special attention regarding handling and management in terms of security and access. Another challenge and one visible in the industrial megatrends is that technology in today’s and future factories has to support the human more and more. Ageing work force and the lack of skilled people in combination with the increasing productivity, quality and cost pressure lead to the need to effectively utilize the unique human capability of purposeful behavior. IoT technologies can help to support the humans and to disburden them from doing hard routine work or wasting their time searching for information. 162 IoT Applications — Value Creation for Industry An important aspect why IoT applications are not as wide-spread as desired is related to human and business aspects. Besides technical challenges there is a lack of business models usable in industrial environment, the business models behind the IoT applications. New types of industrial and business processes for operation and for servicing machineries have to be put in place, considering IoT technologies supported approaches which otherwise would not have been possible. It is also challenging to integrate new IoT applications into existing running and producing plant systems with minor drawbacks – to handle brown field applications. Human resources and skills remain essential and are also a major factor in the new IT and IoT rich industrial environment. The challenges are related to scarce resources, to the complex blend of skills needed by persons on site in a future plant, and by the aspects related to the increasing complexity and knowledge needed for industrial installations. From an industry point of view value creation form IoT applications and sustainability are essential. How these problems will be addressed and solved will influence the use of IoT technologies in the industry, on a larger scale, in the coming years. There is a continuously evolving process both in technology and applications and new future plant, future service and supervision solutions will emerge. 3.3 Future Factory Concepts 3.3.1 Lever Mechanisms for the IoT in Future Factories The term “Internet of Things” describes a wide variety of concepts and appli- cations in the context of equipping everyday items with computing and net- working resources. Even if a common definition of the IoT might not exist, IoT implementations mainly focus three aspects (Figure 3.3): First, the network and addressability aspect. Real world objects are equipped with a computing and communication core and connected to each other. The focus is on high res- olution data acquisition. Second, the ambient intelligence aspect. The network of intelligent objects realizing control loops. The focus is on control. Third, the ambient assistance aspect. High resolution data acquisition and ubiquitous computing are used to offer context sensitive services to the human. This clearly focusses the human. 3.3 Future Factory Concepts 163 io n er s al V i gi n in or le a il ab a v a ge Im The network aspect offers open communication standards reaching down to the sensor-actuator level of today’s factories and the distribution of control intelligence into equipment, infrastructure and products themselves. From a visionary point of view every item in future factories will be equipped with a computing and communication core. Communication allows delivering a detailed and actual virtual representation of the current state of the complete factory. Standardized communication interfaces and distributed control intelli- gence within a factory internet lead to the fact that fine grained and actual information about products, equipment, technological and even organizational processes will basically be available at any time and everywhere within the factory. While this is miles away from today’s state of the art in factories the mere availability of information does not create any added value by itself. The availability of information is only the basis for the optimization of techno- logical and organizational processes. The optimization itself has to be initiated and conducted by humans. This consideration leads to the insight that for future factory concepts the rather technical IoT aspects of networking and communication are only means to an end. The added value of IoT applications emerges out of the fact that humans can take advantage out of the availability of information and the 164 IoT Applications — Value Creation for Industry interoperability of devices. This means that the ambient assistance aspect of the IoT is the one that will be the basis for optimization of future factory processes and that will lead to a number of use cases. Future factory concepts based on the IoT will need to be human centered. The core element is to release the human operator, engineer or manager from doing routine work. Instead humans will be able to concentrate on their unique capability of defining the right strategy and defining the right goals to operate the factory effectively within the triangle of tension between costs, quality and output. In this context the ambient intelligence aspect gets a new meaning. Following the nature of the IoT of making information available, ambient intelligence is the instrument to release the human from routine tasks concern- ing information retrieval and analysis. Autonomous behavior results from the defined reaction of equipment or infrastructure to the results of this analysis. So autonomy of equipment is no contradiction to the need of deterministic behavior at all. 3.3.2 The SmartFactoryKL Initiative In order to transfer the central paradigms of the IoT to factory automation, many technologies working well in the consumer world have to be applied under industrial conditions. One of the biggest obstacles keeping responsibles away from the application of new technologies is missing trust and the lack of best practice examples. For this reason in 2004, a group of people from industry and academia met and formulated the vision of a smart factory of the future. After feasibility study the technology initiative SmartFactoryKL was founded in 2005 as a pub- lic private partnership. Its target is to develop, apply and distribute innovative industrial plant technology. The founding partners represented various indus- try sectors. Meanwhile the number of partners has grown up to 22, including mainly partners from industry as well as universities and research centers. The funding is based on membership fees and public research projects given by German ministries and the EU. The basic equipment of the SmartFactoryKL is an automated production facility for liquid colored soap (Figure 3.4). It contains a process manufactur- ing part as well as a piece handling part. Based on state of the art automation technology the equipment demonstrates the migration path to the application 3.3 Future Factory Concepts 165 Fig. 3.4 SmartFactoryKL production facility. of smart technologies in factory environments. In the meanwhile several addi- tional demonstration modules have been set up showing the application of new technologies and paradigms like Service Oriented Automation architectures, Digital Product Memories, Plug&Play automation, Dynamic orchestration of automated processes and the use of tablet computers in industrial environments. 3.3.3 From Technologies to Technology Concepts Often the discussion about the IoT in the context of industry applications is of technological nature. However, as stated before technology is only a means to an end. Experience with the SmartFactoryKL shows that instead of losing oneself in the discussion of technical implementation details one should focus on the application type of IoT technology. Regarding the future factory this are basically the smart product, the smart equipment, the smart infrastructure and the augmented operator. These concepts can be implemented using a wide range of technology depending on the concrete scenario. 3.3.3.1 Smart products One of the approaches to connect the different information layers of a fac- tory is to take advantage of the product itself as information carrier. Such a smart product primarily stores information about its production history and can 166 IoT Applications — Value Creation for Industry Fig. 3.5 Digital product memories in open-loop processes. actively adapt its production sequence based on the current status of produc- tion (Figure 3.5). Another feature is the capability to realize a tight monitoring of events influencing the smart product. Basic elements are the memory itself and a software component that allows accessing and interpreting the stored information. It is obvious that smart products can be implemented by applying an embedded system to the product itself. However there are many scenarios this is not really reasonable, either for cost, for size or for physical reasons given by the production process such as heat treatment or electrical discharge machining. Therefore the implementation often incorporates infrastructure such as barcode- or RFID Readers and backend server systems enabling to tag the product with robust and cheap items like bar- or data matrix codes or RFID Tags. Using auto-ID tags means that the smartness is only enabled only dur- ing specific time frames of the active production phases by a complemen- tary infrastructure. The complementary infrastructure allows the accessing of the product’s on-board information and couples it with the logical border components. For example these “smart characteristics” are active only in the 3.3 Future Factory Concepts 167 time-frame before a product enters the assembly process, during which it deter- mines the most appropriate assembly station. For most production processes this is not really a disadvantage since the equipment processing or transporting smart products typically has access to the backend infrastructure. Even con- tainers or stores can be equipped with the respective means to access backend server systems. 3.3.3.2 Smart equipment and smart infrastructure Smart equipment and smart infrastructure in future factories are characterized by two major features. First they will be networked. Second they will come with a certain degree of autonomy where networking enables to react on a wide range of context events. The ultimate goal of the smart equipment is to autonomously determine the appropriate processing tasks (e.g., a suitable machining strategy) to compensate failures and to communicate its production states and work-loads with the other production components. The autonomy level will mainly be enabled by reacting on the equipment’s context. The smart infrastructure captures and communicates the environmental changes, like temperature, vibration or the location of the production components within the factory. While smart infrastructure primarily focusses the network aspect, smart equipment more focusses the autonomy aspect. The aspect of networking has to be defined in a more general manner as it is realized today. Today’s networks primarily enable the transport of data. The meaning of this data is typically hardcoded in the applications following some paper definitions. Many industrial networks define such information models for the typical application field such as motion control or real time IO. However networking in the context of the IoT describes the technology independent ad hoc communication of self-describing data. This means that the communication partners are not known at the design time of the network and that the underlying information models describing the meaning of data have to be made explicit and included within the communication protocol. OPC-UA is such a communication technology which already exists and which is being more and more adapted by equipment manufactures. While today’s factory automation is organized in a strictly hierarchical and pyramidal structure, the introduction of distributed control and context sensitivity will lead from the automation pyramid to the factory internet. The 168 IoT Applications — Value Creation for Industry Fig. 3.6 From the automation pyramid to an automation network. strong hierarchical control and communication structures will disappear (Fig- ure 3.6). One of the main reasons for today’s hierarchical structure has been the real time constraint for the past decades. Automated processes typically require the reaction to events within a few milliseconds. Therefore specialized automation networks have been set up not being compatible to the standard TCP/IP protocol. Here, the central logic controller of an automated produc- tion line acts as information gateway by aggregating the various signals to performance indicators and offering them to the upper layers of the pyramid. However today it is foreseeable that future revisions of the TCP/IP standard will offer better real time support. Real time behavior will evolve to a quality of service aspect enabling the realization of real time enabled subnets and offering the addressability of every network partner. 3.3.3.3 The augmented operator The utilization of the smart product, equipment and infrastructure will lead to a huge amount of available data. The human will need to access situation dependent filter mechanisms in order to decode the data noise. Context infor- mation such as the task, the role or the intention of the human as well as 3.3 Future Factory Concepts 169 the location, product status or costumer information can help to identify the situation and to configure the filter mechanisms. While the consumer world already takes advantage of context sensitive applications, industrial applications are still missing. In contrast to the con- sumer world the relevant context information is distributed over a large number of IT systems like enterprise resource planning, plant data collection or prod- uct lifecycle management systems and even sensor systems e.g., to localize the human operator. The key to the realization of context sensitive data tailoring is the effective implementation of data krakens interfacing all the different systems and extracting the relevant context information. The context sensitive assistance requires the integrated access to every layer of the company and so requires the existence of a nonhierarchical factory internet. From the point of view of the human machine interface augmented reality interfaces offer a lot of potential. Notably tablet computers with integrated cameras, high computing power and the capability to wireless networking are very well suited to implement such applications. The camera can be used to identify objects; the networking capability enables the access of various content data bases and the high computing power enables the superposition of the identified context to the real image. A SmartFactoryKL survey showed that about 78% of the participants can imagine the utilization of augmented reality in the context of factory automation. Major application fields are maintenance, logistics and training. 3.3.4 Lessons Learned The mentioned technology concepts have been implemented by a number of SmartFactoryKL partners from industry within public and private projects. The following conclusions reflect the experience gained in those projects since the founding of the technology initiative in 2004. From the pure technological point of view huge advances have been made in recent years. Auto ID technologies, Wireless communication standards and Ethernet based field busses have reached a high level of maturity. With well accepted standards like OPC-UA, specifications and reference implementa- tions of non realtime M2M communication are being developed. Field devices as well as MES and ERP software systems implementing OPC-UA are offered. 170 IoT Applications — Value Creation for Industry So in terms of networks and addressability of factory components basic IoT technology is available. IoT applications often base on the content generated within the complete lifecycle of products as well as equipment. Bringing IoT applications to life means to solve the digital factory dilemma first. I.e., make PLM tools inter- operable, make information from various CAx systems analyzable and bridge the gap between product development and manufacturing. In today’s compa- nies typically various engineering tools are used. The reuse of the generated models is limited due to incompatible data formats and implicit modeling prerequisites. Here the application of semantic meta models is a promising technological approach. However, there is a huge organizational issue in the cooperation of functional entities in today’s companies. Introducing the IoT in factories means to make information streams lean. While the stream of material and products through the plant has been subject to optimization for quite a long time, the meaning of information and knowledge streams is not yet in the focus of production engineers. Analyzing information and knowledge streams in today’s factories is neither a core competence of automation nor of production engineers. We need experts and methods to focus the knowledge and information streams. Applied informatics has to enter our companies focusing the instantiation of methods and algorithms. If the basic technology is available then implementing IoT applications is a matter of specification and integration. The specification of such processes and services is a creative act and requires both technological competence and domain knowledge in the application field. The relation between IoT tech- nology and application relevant performance indicators (i.e. more turnover, better product quality, etc.) is neither trivial nor self-explaining. Not the tech- nology itself but the use of technology yields to better processes and in turn to a benefit. Experts in both domains are very rare. Here, one of the core issues realizing IoT applications seems to reside. Compared to purely technology oriented projects, the number of application projects within SmartFactoryKL is small. Finally, as those lessons learned show, introducing the IoT to the factory has a deep impact on factory processes not only on a technological but also on an organizational level. In order to get the most out of this ongoing change, primarily experts and methods are needed to assess the benefit of new processes and added value services based on the abundant availability of information. 3.4 Brownfield IoT: Technologies for Retrofitting 171 3.4 Brownfield IoT: Technologies for Retrofitting The Internet of Things aims to be a disruptive technology in many ways and may change how future industry will work. However, enabling technologies like RFID or Wireless Sensor Networks are in place, it is often hindered by the fact that huge investments are needed and the local value is considered too low for adoption. The creation of a global network of various ubiquitous networks is one of the driving technological vision behind the Internet of Things. The economical vision of creating domain-and network-wide business fields and usage scenarios by pervasive information networking uses the “Internet” both as a technical and economical analogon. On one hand, as the global IP-based network that connects over 5 billion devices of different networks, and on the other the resulting economic growth and business cases. The interesting fact is, however, that a lot of the enabling technology of the Internet of Things is made to work in a very resource efficient way that hinders such efforts. Novel applications are often enabled only by proprietary technology that uses local optimizations and does not primarily consider inter-networking aspects. Industrial infrastructures are often older that the networks that formed the initial Internet. They can by no means be considered a green field, but consists of a large installed base with machinery that has lifetimes of up to 40 years. Thus many of the applications of IoT technology (as depicted in Figure 3.7) that we consider to have high potential value involve retrofitting Fig. 3.7 High value use cases for IoT retrofitting. 172 IoT Applications — Value Creation for Industry Fig. 3.8 Iot supported interactions as part of a complex Cyber-Physical-System. industrial systems with IoT systems. These “brownfield” use cases are all targeted towards optimizing existing processes by decreasing the gap between the real world and the virtual world. They are thus examples for an evolutionary approach towards an “Industry 4.0” that builds upon IoT Technology. As depicted in Figure 3.8 so called cyber-physical-systems in an indus- trial environment are by definition heavily interconnected. They reflect their physical interdependencies also by communication link and data exchange. Technologies like sensor networks and RFID often builds the missing link in such an environment. IoT technology delivers “smartness” and context aware- ness to otherwise “dumb” objects and environments. It puts the human in the loop of many otherwise ad-hoc and unstructured business processes. As a motivating example of a very simple use case for such a system we consider a mobile maintenance use case. In such a scenario a service technician comes onto the site of a client and can identify all machinery parts easily using RFID and ad-hoc device discovery. Based on this information he can build an up-to-date image of the current system state that can be augmented with historical and semantically interconnected data. He can further deploy wireless measurement devices ad-hoc to gather missing parameters to guide his diagnosis and maintenance strategy. Such an ad-hoc setting is an example for the integration of federated heterogeneous sensor information as core of an informed maintenance strategy with high immediate value coming from IoT technology beyond high infrastructural investments. 3.4.1 Cost-effective Technical Integration of IoT Devices A developer of IoT technology has to take various technical requirements into account such as energy, communication bandwidth, communication topology 3.4 Brownfield IoT: Technologies for Retrofitting 173 or processing resources of different IoT systems. Additionally the interoper- ability is crucial to the value of the system. Assuming that in the future the service technician interconnects with a whole range of different types of wire- less measurement systems and smart machines of different manufacturers, the analysis application must be aware of the semantics of all interfaces. Further- more, the ability of the system which consists of heterogeneous components to integrate in the field, to configure and calibrate crucial for the application of ad- hoc networked sensor system in the maintenance scenario. Loosely coupled, document-based Web services provide a well-defined path to configuration and measurement data from wireless ad hoc systems and automation systems, however, have the disadvantage of a very high runtime overhead. First, standardized ways must be found to obtain comparable quality data sets with opportunistic, distributed measurements. In addition to the demands on the sensor also just coming aspects of this case are concerned. In distributed measurements, such as fine-grained synchronization of distributed measure- ments of importance, and therefore optimized MAC protocols are required. Second, a live data acquisition, needs a high throughput of data (eg ∼, 2 kHz ∗ 3 channels × 16 bits = 96 kbps) to ensure that, while energy efficiency of the hardware, requires a high efficiency of bandwidth usage. The IEEE 802.15.4– 2006 2.4 GHz PHY supports ideally a fixed channel data rate of 250 kbps with a maximum payload data rate of approximately 101 kbps. Without optimiza- tion, the sensing and transmission of a three-axis acceleration value is simply not feasible at 1 kHz bandwidth. Therefore many vendor resort to proprietary solutions. This also explains why, despite increased standardization efforts in this area have shown only limited influence. Even on top of successful standardizations like 802.15.4 there is a fragmentation of protocols like ZigBee (Pro), or the wireless HART OPC binary protocols that address domain specific problems. This foils efforts for cross-domain applications. If we broaden the scope and think of a real Internet of Thing technologies used range from Web Services and Wireless LAN via proprietary sensor and home automation networks globally used wireless technologies such as EPC protocols for RFID applications. More diversity is introduced through a variety of programming models, tool support, operating systems, and programming languages parallelism. Especially, data from different (ad-hoc) measurements, e.g., wireless sens- ing devices, need to be propagated and integrated in a reusable way, to provide a smooth propagation path of data from on-site mobile data collection towards 174 IoT Applications — Value Creation for Industry Fig. 3.9 TECO-Generated gateway architecture for exposing IoT to an ad-hoc SOA. enterprise data management. Mature exchange formats for time series and transient recording in the field already address the problem of describing the measurement conditions. While the time series data is a structured format, the measurement description and the configuration file which are essential for understanding and analyzing the data at a later stage, are not often avail- able in a machine-readable and semantically self-descriptive form. Building on top of this philosophy, for example, loosely-coupled structured message- based web-services provide a well-defined way to exchange configuration and measurement data with sensor nodes and automation systems [12, 13]. We have developed pragmatic light-weight strategies for combining on-site online (real-time) measurement and configuration services with post- measurement enterprise data-exchange in a consistent way by using web ser- vices and model-based data transfers down to device level. In general, wireless ad-hoc measurements highly depend on the measurement context. By building on ideas presented in we can provide a contextual framework for feder- ating this information (with respect to context parameters such as: location, time, causal relation to configuration and setup, measured equipment, sensor, device capabilities). We describe aspects specific to field measurement and maintenance tasks in semantically linked way and the framework will directly integrate existing workflows with a mobile ad-hoc measurement setup on-site. In contrast to common existing approaches we especially trade-off between 3.4 Brownfield IoT: Technologies for Retrofitting 175 complexity and practicability of data gathering in terms of overall cost for implementation by work practices and in terms of overall cost for adoption of third-party vendors (typically problem-focused SMEs). To lower the cost for developing services, protocol translators and gateways we heavily employed model driven development tools. Considering the con- sistency and tool support for data meta-models we decided to use the EMOF (ISO/IEC 19502:2005). We use subset of EMOF as our intermediate represen- tation for generating service that is also known as the class of regular nested word languages. Their properties make it particularly suitable for representing XML structures and for supporting modular design of protocol translators on networked embedded devices. On this basis we have developed a model-driven work flow within the Eclipse Modelling Framework for developing message translation for IoT subsystems that largely automates the process of devel- oping gateway system as well as message binding for the IoT platform. With this system we are able to quickly adapt multiple standard and proprietary platforms to service oriented architecture that seamlessly integrates into the existing IT landscape and enable new forms of human machine interaction like ad-hoc sensor augmented reality for maintenance. 3.4.2 Cost-effective Process Integration of IoT Devices Not only the integration but also the IoT enabled processes needs to be cost- effective by design and well integrated. Our approach is evolving around exist- ing processes and scaling with the human information consumer, rather than solely relying on big data analytics and total connectivity. 1. Opportunistic data collection through local infrastructures and ad- hoc mobile access 2. Context-aware interlinking of heterogeneous data starting from existing processes 3. Human agility and expertise supported by a human-centered infor- mation design Global interoperability in contrast to global connectivity and the use of mobile devices can enable the user to access IoT services ad-hoc. Users are informed in-situ by distributed sensing system, heterogeneous linked data sources and social media paradigms. Building a sensing enterprise from 176 IoT Applications — Value Creation for Industry existing technology will require a considerable jump forward in terms of sensing system deployment and configuration, reasoning on linked data, human-computer interaction and adaptable work flows. New approaches are needed for context-aware annotation, synchronization, visualization and trig- gers on local and remote data. Current studies have shown that huge saving potentials in existing pro- cesses are targeting the work of engineers on site. There is still a potential of 10–40% for making work more efficient and an even higher saving poten- tial (10–50%) from relieving the workload [15, 16]. With more information sources at hand, the overall efficiency may increase in the long run. However, the additional responsibility of collecting and documenting fine granular life- cycle information may add extra responsibilities to each worker, thus increas- ing the workload. In the backend, new intelligent methods based on semantic technologies and tools for processing and analyzing historical and on-site data are still nec- essary to deal with the different qualities of data. Indeed, collection, retrieval and analysis of real- and life-time data need to be seamlessly integrated into real service and design processes without producing unnecessary (cognitive) overhead for the human engineer on and off-site. In conclusion, a combination of integrated technologies is expected to offer new methods, tools and appli- cations to create market opportunities in terms of new services, new methods and software. New business models that support and enhance cooperative net- working among the enterprise assets and artifacts are expected to arise. One of the earliest work in this direction was done in the EU FP6 CoBIs Project (http://www.cobis-online.de) Work with relation to proactive user sup- port for unstructured tasks considering processes and real-time information was also researched in the ADiWa project (http://www.adiwa.net), funded by the BMBF (German Federal Ministry of Education and Research) or European projects like makeSense. The makeSense (http://www.project-makesense.eu) research project is focuses on modeling business processes where a subset is compiled into executable code that is directly executed by a Wireless Sen- sor Network. As enterprise data is usually very long-living and of continuous value, but CPS need to frequently adopt and reflect changes in the produc- tion evolution, the FP7 Timbus project (http://timbusproject.net/) is looking at timeless context-aware business processes and supports the long-term con- tinued access also to IoT generated data and underlying dynamic analysis infrastructures. 3.5 Smart Objects, Smart Applications 177 3.5 Smart Objects, Smart Applications In the Vision of an Internet of Things interconnected Smart Objects play an important role. Such a Smart Object is a bi-directional communicating object which observes its environment and is able to make decisions depending on the application and based on the information extracted from the physical world. One approach to Smart Objects is based on the technology of wireless sensor networks, as they already provide the communication platform and the sensors. The ISO/IEC JTC1/WG7 Working Group on Sensor Networks has designed reference architecture Figure 3.10, which separates the sensor node functionality into three layers: Communication as part of the basic functions layer: describes the communication protocol for the interaction of a smart object with other smart objects, an infrastructure or backbone networks. Service Layer: represents a set of functions commonly required, such as sensor information gathering, filtering by various policies and rules, data comparison and analysis, data mining, context mod- eling, context-aware processing, self-localization, context-aware decision and estimation. Application Layer: realizes the use case of a smart object by a set of functions to users to meet defined requirements. Fig. 3.10 Architecture overview of interconnected smart objects. 178 IoT Applications — Value Creation for Industry From the users prospect the smartness of a smart object is realized within the service and the application layers. Additional value can be achieved through generic, reconfigurable Smart Objects. They offer a set of services and functions for a specific application domain and are adapted to project or user requirements by reconfiguration by a user or a service provider. Smart objects are designed as miniaturized, low power microelectronic systems based on micro controllers, transceivers, sensors and energy supply. As these microelectronic systems provide very limited resources (i.e., processing power, memory) reconfigurable software implementations for smart objects become a challenge, especially when reconfiguration should be possible by a user without code programming (requires easy programming) or if reconfiguration should be done over the air (requires minimum code size). In common service oriented approaches a plurality of service components are defined. Figure 3.10 shows some components for an asset tracking application. The software interfaces of such components are well defined and communication is typically handled by a service manager in a message ori- ented way. Reconfiguration is done by adding or changing components or by changing the functionality behind the interfaces. This is done by code programming of the components and by software update on the smart object. Code Programming and data-intensive software update can be avoided by the new approach of smart applications. Like in the service oriented approach smart applications consist of soft- ware components. In addition the components are supplemented by rule based processing and interfaces. Each component has its own rules set and parame- ters. The rules processing engines are able to handle events, such as messages, interrupts and synchronization. For the definition of rule sets for the application modules a universal con- figuration language SAL (smart application language) has been developed at Fraunhofer IIS. The main goal of this language is, to describe instruction cycles which are triggered by incoming events. On the one hand the instructions can have executive characteristics on the other hand they can act as conditions in if-else constructs. These instructions handle two parameters, which are defined as variables of well-known data types and can be modified at runtime. 3.5 Smart Objects, Smart Applications 179 The smart application technology allows the definition of multiple rule sets for a smart object. Thus, it is possible to configure multiple application modules and interconnect them by logical links. Smart applications can be realized within a node or even distributed over a whole sensor network. In order to make the implementation of smart applications easier for the user, a graphical development environment allows defining rule sets with the help of jigsaw puzzle pieces Figure 3.11. The editor provides SAL ele- ments — such as events, instructions, variables and common language compo- nents — which can be connected to a jigsaw puzzle. The backend generates the corresponding SAL code and the user level is arranged on a higher abstraction layer. The following Figure 3.12 shows the complete workflow of the smart application development. The SAL source code, generated by the graphical editor, is compiled to an optimized and highly compressed byte code. Due to the small size of the resulting data, a complete configuration can be sent via the radio interface of the smart object. It is also possible to send multiple of these configuration sets and store them on a persistent memory as application profiles and switch between them on demand. After receiving a configuration, the smart application manager configures all corresponding modules on the node regarding to the rules. It starts the components in sequence of their application priority. The application is then ready to operate. A reconfiguration can be done at any time and with any Fig. 3.11 Service oriented approach — left and Smart application approach — right. 180 IoT Applications — Value Creation for Industry Fig. 3.12 Smart applications workflow — from a jigsaw puzzle to the application on the node. configuration profile. The node can also be configured with a standard profile, which is loaded at the boot process of the system. In summary the smart application approach allows changing application functionality by changing the rules of interconnected rules engines in the service components. This can easily be done by a graphical puzzle editor. The generated smart application language code is very compact and allows very efficient reprogramming of all or selected nodes over the air. The smart application approach is an ideal solution for adapting generic smart objects to different projects or use cases in the same application domain. 3.6 Four Aspects in your Business to Master IoT 3.6.1 Internet Conquering Product Business In order to deliver value for business it is too narrow to just look at connectivity. It is important to look at the business process and the benefit for the involved stakeholders in a specific application. In recent years, the internet has transformed communications (Voice over IP, Twitter), the media landscape (news, advertising), commerce (eBay, Amazon) and the music industry (file sharing, online music stores). Now, smartphones and tablets are helping it to permeate our professional and private 3.6 Four Aspects in your Business to Master IoT 181 lives. Given that daily life is ever more interactive and networked, and our contacts ever more global, I increasingly expect everyday objects to be more intelligent and networked, too. The Internet of Things (IoT) is the next generation of the internet. It is a global system of interconnected computer networks, sensors, actuators, and devices that use the internet protocol to potentially connect every physical object. By merging this physical world with software from the virtual world, organizations, companies, and consumers will benefit from new services that emerge from web-based business models. In the final analysis, however, IoT stands for the start of a series of technological and above all economic changes that will revolutionize not only the marketplace as we know it but also the lives of each and every one of us. The Internet of Things & Services is merging the physical and virtual world. Impressive is the growth that is seen in internet access. Whereas in 1995, less than 1% of the world’s population was online, this number has exploded: 2.3 billion people were online in 2011, while for the year 2015 we expect 5.5 billion people to have internet access (source: ITU). This equates to around 75% of the world’s population, Figure 3.13. Expected devices connected to internet have been estimated by Bosch Software Innovations, to 6.593 billion by 2015, Figure 3.14. People connected to the internet Fig. 3.13 Impressive is the growth that is seen in internet access. 182 IoT Applications — Value Creation for Industry Devices connected to the internet Fig. 3.14 By 2015 expected IP-ready devices, connected to the internet, 6,593 billion. 3.6.2 Strategic Business Aspects For us the Internet of Things & Services, Web 3.0, m2m, or cyber physical systems are much more than just buzzwords for the outlook of connecting more than 6 billion devices by 2015. It is a chance and a challenge to bring the internet and physical world closer to each other. We understand the Internet of Things & Services along four dimensions: Four Aspects of the Internet of Things & Services Technology: The internet and its technology are vivid drivers offer- ing an established platform for interconnecting billion things — from tiny sensors, smart phones, PCs, to high performance comput- ers. Open Source communities scale and accelerate the technology development and the implementation of open standards. Therefore, business moves to system and software platforms in the internet. Business Innovation: Weaving smart things, enterprises, and people leads to innovation in services and business models. The spirit of internet business models is turning up in traditional product business (e.g., pay-per-use for car sharing). Market: Different industries meet the first time as the Internet of Things & Services crosscuts some of today’s separate markets (e.g., Electric Vehicle Roaming with Energy and Mobility com- panies). The players of these markets compete and cooperate in 3.6 Four Aspects in your Business to Master IoT 183 Business Innovations Market Disruptions Weaving smart things, enterprises, and Players of different industries compete and people leads to innovation in services and cooperate in new market segments leading to business models. business ecosystems linked to communities. People Competences Technology Advances Software and system competencies linked to Internet technologies offer a platform to inter- deep domain knowledge and enlightened with connect billions of things. Open Source creativity are the core for innovation in communities accelerate the development and technology and business. implementation of standards. Fig. 3.15 Internet of Things & Services four dimensions. new segments leading to business ecosystems that are linked to social communities (e.g., Google+) and open source communities (e.g., Eclipse). Competencies: Software and system competencies linked to deep domain knowledge and enlightened with creativity are the core for innovation in technology and business. 3.6.3 Vertical Business Domains for IoT The value of the Internet of Things & Services technology is delivered in vertical application domains. There are many hot candidates to be early movers such as connected energy and connected industry. 3.6.3.1 Connected energy We are currently witnessing a paradigm shift in today’s energy market. From the dogma of a production structure with large power plants to a world of many small, distributed power generation systems. Low voltage networks are especially affected by these changes and are facing new challenges. Today, the many distributed power generation systems are connected to the low voltage grid, but not transparently. Consequently, distribution grid operators are forced to react instead of being able to act in order to ensure network stability. 184 IoT Applications — Value Creation for Industry Connected Mobility Connected City Enabling business success in a connected world Connected Energy Connected Industry Connected Enterprise Connected Life Fig. 3.16 Applications for the Internet of Things & Services. While large power plants operate based on accurate and agile schedules, decentralized power generation plants are often operated along subsidy poli- cies and not according to the forces of the electricity market. This leads to an uncontrollable volatility in production and highly fluctuating market prices. It also forces transmission and distribution network operators to maintain high balance energy capacities in order to ensure grid stability. Aiming for a better integration of distributed power generation systems, regulations are changing, and with it the demand for intelligent energy man- agement systems is increasing. Their purpose is to provide for a transparent integration and control of distributed systems to improve the efficiency of sub-systems (e.g., a microgrid) in the smart grid as well as to give both new and established market players the opportunity to profit from new market potentials. The virtual power plant is an example of how the operation of such a sub-system can already be profitable based on Internet of Things & Services technology. The service is supposed to help implement new business mod- els, not only for traditional market players such as local energy producers, traders, aggregators and operators, but also for large companies and indus- trial parks. They could use the service to make profit, e.g., by offering spare capacities of unused facilities. 3.6 Four Aspects in your Business to Master IoT 185 3.6.3.2 Connected industry The Internet of Things in production and logistics is coined with the term “Industrie 4.0” in Germany. Industrie 4.0 is paving the way for a social and technological revolution that will drastically change the entire industrial land- scape. Why 4.0? As since the 18th century, when the first Industrial Revolution began, it is the fourth wave of major technological changes. Industry 4.0 is a sophisticated approach changing the entire global value chain: communica- tion, planning, logistics and production. The German industry, academia, associations, and unions compiled a report on how this transformation can be achieved: “Industrie 4.0 holds huge potential. Smart factories allow individual customer requirements to be met and mean that even one-off items can be manufactured profitably. In Indus- trie 4.0, dynamic business and engineering processes enable last-minute changes to production and deliver the ability to respond flexibly to disruptions and failures on behalf of sup- pliers, for example. End-to-end transparency is provided over the manufacturing process, facilitating optimised decision- making. Industrie 4.0 will also result in new ways of creating value and novel business models. In particular, it will pro- vide start-ups and small businesses with the opportunity to develop and provide downstream services. In addition, Indus- trie 4.0 will address and solve some of the challenges facing the world today such as resource and energy efficiency, urban production and demographic change. Industrie 4.0 enables continuous resource productivity and efficiency gains to be delivered across the entire value network. It allows work to be organised in a way that takes demographic change and social factors into account. Smart assistance systems release workers from having to perform routine tasks, enabling them to focus on creative, value-added activities. In view of the impending shortage of skilled workers, this will allow older workers to extend their working lives and remain productive for longer. Flexible work organisation will enable worker to combine their work, private lives and continuing professional 186 IoT Applications — Value Creation for Industry Fig. 3.17 Bosch Software Innovations reference model for the Internet of Things & Services. development more effectively, promoting a better work-life balance.” [source: acatech 2013] The national academy of Science and Engineering (acatech): Recommendations for implementing the strategic initiative INDUS- TRIE 4.0, April 2013 Securing the future of German manufacturing industry, Final report of the Industrie 4.0 Working Group. 3.6.4 Reference Architecture and the Core Competence for Business The business success in one vertical domain is the key entry point, but success- ful architectures will reach out to other verticals later. Only architectures that can cover multiple domains will be successful in the long run, as the domain “silos” of the past still prevents a lot of innovation between the domains: e.g., between automotive and energy in electromobility. 3.7 Auto_ID — Value Creation from Big Data and Serialization in the Pharmaceutical Industry Industries are maturing at a faster rate than ever before — manufacturing is increasingly distributed and outsourced — and where costs have been taken out 3.7 Auto_ID — Value Creation from Big Data and Serialization 187 of production systems through initiatives such as Lean Production [18 Womak, 1990], companies are increasingly looking to optimize savings across the total product lifecycle. This chapter explores IoT technology as a value creation capability rather than as a cost optimization strategy, specifically exploring the value of data that is collected from multiple infrastructures across a prod- uct lifecycle and where the Auto-ID serialized identifier may serve as a key to linking relevant data to individual products, processes and related outcomes. Ultimately the hope would be that data from anywhere in the product lifecy- cle might facilitate the development, trials and approvals of New Chemical Entities (NCEs) and New Biological Entities (NBEs). 3.7.1 Background — Serialization Role in an ‘Internet of Things’ As industries instrument complex processes beyond manufacturing plants in the supply chain and aftermarket services, Automated Information Data Col- lection (AIDC) technologies including optical scanning of printed linear or 2D bar codes, radio frequency “reads” of passive RFID tags together with new telemetry technologies, provide a powerful portfolio of tools for product lifecycle visibility. Whether sensors are connected in an ‘Internet of Things’ via M2M protocols, via Enterprise applications or via the Cloud [19 Auto-ID “Cloud of Things”], leveraging AIDC standards is important to making data accessible for value creation. Serialized identifiers are the keys to building an Internet of Things; just as unique IP addresses are integral to the web itself. One global system of such identifiers, the MIT Auto-ID Center Electronic Product Code (EPC), was licensed by GS1 for use by its member manufacturers in all 124 coun- tries, together with EPCGenII RFID specs, are now instantiated in ISO Auto- matic Identification and Data Capture Techniques [20ISO18000-6c]. The GS1 serialized Global Trade Identification Number (sGTIN) is being used for mon- itoring — a single Item Class in a 96 bit tag supports up to 200Billion #’s. Examples of serialization initiatives from this researcher’s experience include part-marking schemes for aero-defence, automotive and high tech where up to 80% parts communality is shared between competitors, to tracking tobacco sales tax compliance using INTERPOL Global Register [21 IGR] or EPCGenII serialized RFID tags [22NXP, Quanray] for authenticating 100 m+ alcoholic beverages in China and the UPU Global Mail Quality tracking system (10,000 readers in 50 countries). 188 IoT Applications — Value Creation for Industry Fig. 3.18 MIT CBI BIOMAN biomanufacturing technology roadmap. 3.7.1.1 Big data in the pharmaceutical industry A radical transformation of the pharmaceutical manufacturing industry is taking place, much as occurred previously in the textile and electronics man- ufacturing sectors. According to the FDA, imports from China to the US are expanding at a rapid pace, from 8 m shipments in 2002 to 28 m in 2012, and are expected to grow to 34 m in 2013. In fiscal year 2011, more than two mil- lion lines of FDA-regulated products were presented for entry into the United States from China. Simultaneously with the growth in data from normal supply chain processes, an industry transition to distributed, disposable and contin- uous manufacturing processes as foreseen in the MIT Center for Biomedical Innovation BIOMAN consortium roadmap promises explosive growth of Big Data in this industry. Big Data can be compared to the discovery of the microscope, Professor Eric Byrnolfsson, Ph.D. said in his keynote at the MIT Sloan “Big Data: The Management Revolution” conference and in a recent Harvard Business Review article [23 Brynolfsson, 2012]. Big data holds huge, largely untapped potential to change the way healthcare functions as an industry. MIT is studying it in a five year US$ 12 million partnership [24 BigData@CSAIL] with Intel to explore techniques for organizing and making sense of the huge amounts of information generated by Web users and networked sensors. Furthermore data from the ‘last mile’ of the supply chain such as the ability to track product and the data associated with patient interactions and outcomes as recorded in the electronic Health Record (eHR) has eluded the industry until 3.7 Auto_ID — Value Creation from Big Data and Serialization 189 now. The biologics industry has been a pioneer in re-establishing data linkages between pharmaceutical manufacturers and clinical service providers in the context of developing stratified medicine and autologous cell based therapies which require matching the right cells with the right patients. We can see this linkage of product and patient outcomes at the center of the “7 Flows” model proposed by Genzyme executive Laurent Boer, VP Global Distribution & Logistics and General Manager of the Genzyme Northborough Operations Center, as a framework to think about the kinds of data that are required for managing pharmaceutical industry processes [25 Boer, 2013]. Fig. 3.19 The 7 flows of supply chain information — Laurent Boer, VP global distribution & logistics, Genzyme — Sanofi. In the following section we will review these (7) data protocols. The connection between a product and a patient outcome is an area of great promise for pharmaceutical manufacturers developing stratified medicine therapeutics. 3.7.1.2 Tracking serialized products In the healthcare industry there is no question that both regulatory agencies and pharmaceutical product manufacturers would be better off with identification systems for tracking products, producers, production sites as well as individual 190 IoT Applications — Value Creation for Industry patients and associated product-patient outcomes in order to optimize the development and distribution of cost effective and quality therapeutics. The industry is already making significant investments, in response to regulatory mandates from countries including Argentina, China, Turkey and the State of California, to modify production-labelling systems to support serialization. In the first phase of implementing serialization the pharmaceutical industry is adopting 2D optical bar code as a transport and the GS-1 serialized Global Trade identification Number (sGTIN) symbology. In tracking physical goods there is some debate about whether e-Pedigree specifications should include a requirement for tracking the aggregation/disaggregation of each item as it moves across the supply chain — adding expense to the process. One industry expert cites the difference in some businesses as a cost differential between $20,000 per manufacturing line and $200,000 per line for the manufacturer. The distributor/retailers however support tracking aggregation so that they can ship and receive items at a higher shipping hierarchy, i.e. pallets versus cases, or cases versus “eaches” in the supply chain, since reading 2D barcodes on conveyors running at speeds of 200+ crates per minute will be difficult. Further research and guidance from regulators is required to ensure tracking systems interoperability. 3.7.1.3 The value of supply chain data Product identifiers on a label can serve as a “key” to information about the processes and conditions through which the product has travelled. Once prod- uct label serialization is implemented, as in the case of recent deployments by apparel companies including Macy’s and American Apparel, the first question these organizations must address is, as Joseph Andraski, President of Voluntary Interindustry Commerce Solutions Association (VICS) ecommerce Standards Data Organization (SDO) has been asking is, “What to do with the data?” One answer is that the pharmaceutical industry would clearly benefit from knowing “who” is making “what”; “where” products are being manufactured; under what conditions they are being produced; and how the aforementioned factors interplay with these elements to lower commercial friction or, by con- trast, to create elevated risk in the manufacturing and supply chain. One example of the value that can be derived from serialization was presented in a keynote by AbhiDhar, CIO of the eCommerce division, 3.7 Auto_ID — Value Creation from Big Data and Serialization 191 Walgreens, at the Auto-ID Labs 2012 Big Data Conference [26Auto-ID Big Data] organized with GS-1 and VICS: the use of the serialized identifier for prescription refills. For Walgreens today, the majority of web orders for refills are now placed by consumers using a smartphone barcode scanning appli- cation that ‘reads’ the 2D barcode on the pill-box label which the system uses to look up the initial order which is to be refilled. As we can see from this example, Big Data, when tied to individually identified products and/or transactions, allows a company to link data captured in the physical world or somewhere on the web, to Enterprise systems processes. 3.7.1.4 Quality by design The cornerstone of FDA’s quality initiative, Pharmaceutical CGMPs for the 21st Century — A Risked Based Regulatory Approach — takes a Process Analytic Technology (PAT) and Quality be Design (QbD) holistic approach to identifying sources of variability (in raw materials, in-process materials and process factors), to managing variability through process understanding and risk-mitigating control strategies to improve productivity and product quality throughout the product lifecycle. Underlying this approach is the notion that quality should be built-in (i.e., by design). Harmonization achievements in the Quality area include milestones such as the conduct of stability studies, defining relevant thresholds for impuri- ties testing and a more flexible approach to pharmaceutical quality based on Good Manufacturing Practice (GMP) risk management. Specific areas include Stability, Analytical Validation, Impurities, Pharmacopoeias, Pharmaceutical Development and the Development and Manufacture of Drug Substances. As manufacturing supply chains extend around the world, ASTM in E2500-07 and ICH in Q8 (R2), Q9 and Q10 SDO’s have developed guidelines for GMP regulations for the industry. In consortia such the MIT CBI BioMANufacturing Research Program [27 BIOMAN], academia is also working with regulators and industry sponsors to define advanced GMP, PAT and QbD processes for optimized quality man- ufacturing and to develop tools to assess and mitigate risk in biopharmaceu- tical production. One approach the group has been discussing with industry sponsors is to investigate QbD applicability to quality oversight of the global pharmaceutical supply chain. 192 IoT Applications — Value Creation for Industry 3.7.1.5 Legal information flows Legal issues around data exchange can be divided into two separate concerns, one is the question of jurisdiction over data, and secondly, within that con- text, what party(s) own the data. In the US for example, despite a common interest in ensuring efficient oversight and promoting safe and environmen- tally friendly products, the FDA has not been active in developing national standards on transportation and drug packaging, Unit of Use labelling and sustainability guidelines and has instead relied on local and state governments to regulate material composition, reuse, recycling, and recovery of packaging through legislation and ordinance. By contrast EU Directive 94/62/EC, “Pack- aging and Packaging Waste Directive” (PPWD) and the associated ISO/TC 122/SC 4, “Packaging and environment” are driving packaging changes in that jurisdiction. Even where there are no jurisdictional issues, “who owns the data” is a challenge issue for industry stakeholders. Under current US healthcare leg- islation a patient has rights to access their records, but who owns this data, the doctor, the hospital, the software or hardware/services provider? Develop- ing secure marketplace mechanisms for the exchange of patient data was the topic of one session at the PDA/FDA Pharmaceutical Supply Chain Integrity Conference [28 Miles, 2012]. 3.7.1.6 Finance flows Data is a core asset of every company and a strategic resource that can be harvested with advances in AIDC technologies. Every step in the devel- opment, clinical trials, manufacturing, distribution and service delivery of a biologics product involves massive amounts of data. Extensive industry guidelines and best practices are established for the use of this information in specific contexts such as quality control or product authentication. Good Manufacturing Practice (GMP) production and testing practices ensure quality products. A parallel assessment of data asset values, liabilities and exchange mechanisms for these data assets is warranted in light of pharmaceutical devel- opment, technology transfer, commercial manufacturing and product discon- tinuation processes. The value of products as they move through the supply 3.7 Auto_ID — Value Creation from Big Data and Serialization 193 chain impacts what hazards they are exposed to. For example while Viagra still is the most counterfeited product in the world today, cancer drugs now represent an increasing share of that market as reported in the Wall Street Journal.1 It is not surprising to see that those willing to put people’s lives in danger to make money see greater potential in counterfeiting a vial of Avastin that sells for $2,400, than manufacturing a $15 or $20 bottle of fake Viagra. An integrated financial risk management system is required for curating and valuing data assets. 3.7.1.7 Regulatory oversight Automating FDA inspection/data collection processes is but one example of where changes from manual to automated data collection processes is needed. Using today’s onsite inspection methodology, according to the FDA budget request to Congress in 2013, the Agency said it’s inspections of foreign manu- facturers jumped 10% last year to 813 inspections in 62 countries. The Agency said nearly half of the 46 warning letters issued to foreign manufacturers resulted from these inspections. What might those tallies look like if the FDA were able to inspect (200,000) pharmaceutical ingredient production sites in Asia? To quantify the size of the problem, working with FDA partner agencies International Criminal Police Organization (Interpol) and the World Health Organization (WHO), approximately 20 million pills, bottles and sachets of counterfeit and illegal medicines were seized in a five-month operation in China and nearby Asian countries in 2009 [29 WHO, 2010]. Asia has the largest amount of counterfeit medications but these illegal acts can be found worldwide. Automated mechanisms for manufacturing and supply chain com- pliance reporting is an area requiring further collaboration. 3.7.1.8 Product lifecycle management data In the US healthcare market, the lack of visibility over the product lifecy- cle of drugs results in a $11 billion in “revenue leakage,” according to a study by IDC, that could be reduced by better drug tracking [30 IDC Tech Insight, 2010]. In addition to chargeback’s, reverse chargeback’s, duplicate 1 “Counterfeit Cancer Medicines Multiply;” By JEANNE WHALEN And BENOIT FAUCON; (December 31, 2012, WSJ) http://online.wsj.com/article/SB100014241278873233204045782114924523530 194 IoT Applications — Value Creation for Industry chargeback’s, product returns, and concealed shortages, managed care and Medicaid rebates have been a growing concern. In total, the study found that revenue leakage causes pharmaceutical companies to lose approximately 4.4% of overall revenue on an annual basis. For 2009, most forecasts predict U.S. pharmacy sales will be roughly $252 billion. That means as an industry, phar- macy manufacturers will collectively lose approximately $11 billion through channel inefficiencies. That’s equivalent to total revenue for a top 20 pharma- ceutical manufacturer simply disappearing each year. In light of these concerns the FDA has ranked — in order of risk of adulteration — more than 1,000 active drug ingredients. A risk based assessment and model to analyse supply chain data to address lifecycle issues may be an area that big data technologies can be useful — once enough information is collected electronically using Auto- mated Information Data Collection (AIDC) technologies to create meaningful exploratory data. 3.7.1.9 Keeping better track of things In each of the above scenarios we see a convergence of supply chain security, product authenticity and patient safety data and the promise that this data may be incorporated in optimal data sets to facilitate the development, trials and approvals of New Chemical Entities (NCEs) and New Biological Entities (NBEs). In summary, as biologics industry stakeholders, including manufacturers, distributors, health service providers and regulators, invest in serialization ini- tiatives by, equipping production lines with linear and 2Dbarcode and/or RFID labelling machinery and outfitting distribution centres, pharmacies and hospi- tals with RFID/2D barcode readers, this author recommends a commensurate investment in defining and collaborating with biologics industry stakeholders on where the data that is collected can most effectively be used to optimize product security, patient safety and eventually, improved patient outcomes which in turn will feed new product development for research sponsors. 3.8 What the Shopping Basket Can Tell: IoT for Retailing Industry? The Internet of Things has become a dominant term for describing the inte- gration of information with real-world products, items, and things. Internet 3.8 What the Shopping Basket Can Tell: IoT for Retailing Industry? 195 of Things is broad term comprising applications from manufacturing, smart power grids, RFID, mobile applications, track & trace, traffic monitoring, smart cities and retail. Whereas it is not completely agreed upon who coined the term of Internet of Things, there can be generally identified two streams where Internet of Things roots back to. Firstly, there is the Internet-oriented development which aims at expanding the traditional Internet of data from computer desktop devices to mobile hand- sets, lower power devices, down to micro-controller devices integrated and attached physical objects and things. Accordingly, research questions are how to tailor established communication and data protocols for low-power devices with limited computing capabilities (e.g., 6 lowpan). Secondly, there is the thing-oriented development which comes from associating items and things with unique identifiers in order to relate to static descriptions and dynamic status information throughout the lifetime of things. Early developments have been based on barcode and RFID (e.g., GTIN, EPC, UID) and include archi- tecture frameworks helping to resolve unique identifiers to database locations where the information can be stored and retrieved (e.g., ONS, EPCIS). Either way the Internet of Things gives access about real-world processes and phenomena in real time. For instance, it offers the opportunity to integrate social media into the sales floor. This allows retailers to gain more insights into the opinions of their customers and to benefit from viral marketing, as depicted by Figure 3.20. As such, a much more fine-grain understanding of real-world processes can be obtained. Thus, processes can be optimized, decisions can be based on data, and innovative services can build upon new sources of data. In retail where margins are low and revenues are high there has been a long tradition of introducing information systems for making processes more efficient. About 40 years ago barcode stripes have been introduced on product items for accelerating price tagging and check-out at the register. This very same technology, originally designed to be used internally among supply-chain part- ners, has started to reach consumers, as they can use their mobile phones today for retrieving further information about products, such as consumer opinions or price comparison. With the advent of mobile apps from e-tailers allowing to retrieve items consumer experience in stores at cheaper prices online put a new threat retailers: retailers run into the danger of becoming the free show rooms for online retailers. 196 IoT Applications — Value Creation for Industry Fig. 3.20 Embedding social media on the sales floor. Fig. 3.21 Opportunities for retail using IoT. As this technological move is hard to stop retail has to explore new oppor- tunities and services to offer to their clients, see Figure 3.21. Thus, using IoT retails can retrieve valuable inputs from their consumers with regards to their shopping behavior and opinion on products and adapt their offerings more 3.9 IoT For Oil and Gas Industry 197 instantly to the customers’ needs. Furthermore, the allowing the sharing of opinions of customers can yield a new way of establishing trust between the retailer and his clients. With these services retailers should be empowered to gather attractiveness over the electronic retailers. 3.9 IoT For Oil and Gas Industry Internet of Things is per definition access to information everywhere. For process automation IoT can be divided into Service Applications, solutions for the mobile workforce, into wireless Field Devices utilizing different radio solutions to make information in systems and devices accessible and into long range wireless communication solutions for the Remote Monitoring of a process and a plant. In this chapter we present a wireless Field Device targeting the Oil and Gas environment. Process industry in general and Oil and Gas in particular put special requirements on field devices. Devices have to operate under harsh conditions; dirt, often high temperatures and sometimes in explosion prone environments. It is a challenge to develop a field device that not only is easy to install and maintain, have a long enough life length but also withstand this tough environment. Problem description Why is the information from installed Field Devices not already available? Modern process control systems often have the facility to pass HART com- mands through the I/O modules so that instrument configuration can be modi- fied at the host system level. Remote access to HART instruments at the HOST system has been available for years however because of large number of brown field installations with a legacy communication architecture it is estimated that less than 10% of the entire population of Field Devices is connected so that all the information it provides is made available to the higher level system (See Figure 3.22) for a picture of a system). To get access to the information you need to wire in a HART multiplexor which is difficult to do and normally require a plant shutdown. Integrated operations With better connectivity to Field Devices better concepts for safe and cost- effective operations of facilities can be developed which is for both environ- mental and business reasons extremely important for the Oil & Gas industry. 198 IoT Applications — Value Creation for Industry Fig. 3.22 4-20 mA HART has been available since 1990 and is mainly used for instrument commissioning where an engineer connects a hand held at the instrument to configure parameters. Therefore Statoil, ABB, IBM, Aker Kvaerner and SKF joined forces in an R&D effort known as the CORD project in the beginning of the 2000’s to develop new technology for more efficient operation of oil and gas field. The objective was to develop technology, processes and knowledge to extend the lifetime of Statoil’s oil and gas fields, and thus improve the recovery factor. It was to evaluate, test and apply new and open standardized communication system architectures that allow handling increased amount of data from field devices to corporate systems in a cost-efficient and reliable manner. The vision did not stop at the Field Devices but ambition is also to make the maintenance workplace accessible both at site as well as in the onshore operations center. Information should be possible to share both with inter- nal as well as with external experts, i.e., experts from the product or system manufacturer thus facilitating co-operation between user groups and between different locations. Potential with wireless Much of the envisioned solutions only become possible with wireless commu- nication. Wireless technologies have undergone various industrial trials over the last years, which have demonstrated that wireless communication can be 3.9 IoT For Oil and Gas Industry 199 deployed in a wide variety of use cases, ranging from monitoring to safety critical applications. The main obstacle for a rapid adoption of wireless tech- nologies is no longer the lack of suitable technologies; rather it’s the lack of established industrial standards. Without standards, there is no effective means to achieve the interoperable, multi-vendor solutions which is required by cus- tomers. In addition, as many technologies operate in the same frequency band, standards are also required to ensure co-existence of wireless technologies (as wireless is an open medium). The first wireless standards for process automation emerged only some years ago but already in 2002 the CORD project started investigating the pos- sibility to do wireless condition monitoring on small AC motors. The project had mapped degradation mechanisms of these motors by interviewing spe- cialists from the Oil companies and found that the most common and costly failure modes were bearing breakdown due to vibration and insulation failure. In 2004 the project tried to identify and assess different technologies and tech- niques to monitor the degradation mechanisms and the conclusion was that none of the existing “off-the-shelf” condition monitoring systems seemed to meet the requirements. In 2005 the project started to evaluate if and how the monitoring could be achieved by utilizing micro technology and in 2006 the spin-off project Wireless Condition Monitoring project was formed. Why Motors Why was condition monitoring of small AC motors selected? Some of the small AC motors on an oil rig are highly critical with respect to regularity, some are critical with respect to safety if for instance placed in EX (explosion proof) zone. Larger machines are normally always monitored but for these smaller AC motors the then prevailing maintenance strategy was “run-to-failure” due to their high numbers — of up to 1000 units per offshore installation — and the cost associated with monitoring them. A failure leads to high maintenance costs estimated to approximately 10 000 per motor and per repair with a bearing failure being the most critical, causing the larger cost. The definition of AC motors is that they are below 400 kW in size and normally run at around 3000 r/min. At an oil rig these are the motors used to drive equipment such as pumps, air compressors and fans. 200 IoT Applications — Value Creation for Industry WiMon 100 The outcome of the project was an ABB product called the WiMon 100. It is a battery operated device with an expected lifetime of five years that intends to reduce the cost of maintenance as well as extend the lifetime of electric motors. The sensor is Ex-proof and is ideal for use in the offshore sector. In principle, however, it can be used in all industrial sectors with the same benefits. The product uses the new WirelessHART™ communications protocol, which was ratified in September 2007. The Wireless Vibration system delivers a cost effective, secure and reliable data acquisition, analysis, and sharing of real time information. The small, autonomous WiMon 100 unit comprises a vibration sensor, temperature sensor, long-life battery and a WirelessHART™ radio. WiMon 100 was jointly developed by ABB researchers in Norway and Sweden, in cooperation with SKF Reliability Systems and SINTEF (an inde- pendent research organization based in Norway). The project received finan- cial support from the Research Council of Norway through its Petromaks and DEMO2000 programmers, as well as from several oil companies including Statoil and BP. Due to the cost efficiency, small size and ease of mounting and installation of the WiMon 100 sensor, continuous vibration monitoring can be realized for all types of rotating machines. WiMon 100 units form a mesh communication network; providing a secure, reliable and redundant path from WiMon 100 sensor to a gateway and onwards to monitoring and analysis tools (the central system). The central system (PC, network, DCS…) performs the necessary data analysis and storage and makes data available in real time. The system (visualized in Figure 3.23) also contains a WirelessHART™ gateway that coordinates the sensor communication and manages the network security. The gateway device converts wireless device data to a format that is compatible with the wired automation systems. A WiMon Data Manager provides the following main functionalities: a sys- tem browser, a system for commissioning and maintenance support (including firmware upgrade), automated data acquisition and storage of waveforms and dynamic data (velocity, envelope and temperature) in an OPC server, an oper- ator interface for showing vibration waveforms and trends and temperatures and a waveform export tool for the interfacing of analysis packages like the ABB Analyst. 3.10 Opinions on IoT Application and Value for Industry 201 Fig. 3.23 A possible deployment of the WiMon 100 sensor and gateway. The WiMon has been successfully deployed on oil rigs for the North Sea and more wireless Field Devices, also energy harvesting without the need of batteries, are developed and marketed by ABB. More and more information is made available to the benefit of a more efficient and environmental friendly operation of the future oil and gas fields! 3.10 Opinions on IoT Application and Value for Industry At a recent international workshop on IoT application and value creation for industry a quick survey was done asking participants at the workshop on their opinion on value creation using industry IoT applications. The structure of the survey, as shown in Figure 3.24, has asked on IoT areas of application and expected tim