Guidelines for the Evaluation of Prosthetic Valve Function PDF
Document Details
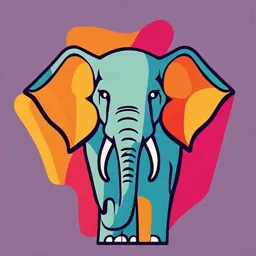
Uploaded by UseableRisingAction
William A. Zoghbi, MD
Tags
Summary
Guidelines for the evaluation of prosthetic valve function with cardiovascular imaging. This document reviews echocardiographic techniques and provides recommendations and general guidelines for the evaluation of prosthetic valve function. The report was developed in collaboration with the Society for Cardiovascular Magnetic Resonance and the Society of Cardiovascular Computed Tomography.
Full Transcript
GUIDELINES AND STANDARDS Guidelines for the Evaluation of Prosthetic Valve Function With Cardiovascular Imaging: A Report From the American Society of Echocardiography Developed in Collaboration With the Society for Cardiovascular Magnetic Resonance and the Society of Cardiovascular Computed Tomogra...
GUIDELINES AND STANDARDS Guidelines for the Evaluation of Prosthetic Valve Function With Cardiovascular Imaging: A Report From the American Society of Echocardiography Developed in Collaboration With the Society for Cardiovascular Magnetic Resonance and the Society of Cardiovascular Computed Tomography William A. Zoghbi, MD (Chair), Pei-Ni Jone, MD (Co-Chair), Mohammed A. Chamsi-Pasha, MD, Tiffany Chen, MD, Keith A. Collins, MS, RDCS, Milind Y. Desai, MD, MBA, Paul Grayburn, MD, Daniel W. Groves, MD, Rebecca T. Hahn, MD, Stephen H. Little, MD, Eric Kruse, RDCS, Danita Sanborn, MD, Sangeeta B. Shah, MD, Lissa Sugeng, MD, Madhav Swaminathan, MD, MBBS, Jeremy Thaden, MD, Paaladinesh Thavendiranathan, MD, SM, Wendy Tsang, MD, SM, Jonathan R. Weir-McCall, MD, MBChB, PhD, and Edward Gill, MD, Houston and Dallas, Texas; Chicago, Illinois; Philadelphia, Pennsylvania; Cleveland, Ohio; Aurora, Colorado; New York and Manhasset, New York; Boston, Massachusetts; Richmond, Virginia; Durham, North Carolina; Rochester, Minnesota; Toronto, Ontario, Canada; and Cambridge, United Kingdom In patients with significant cardiac valvular disease, intervention with either valve repair or valve replacement may be inevitable. Although valve repair is frequently performed, especially for mitral and tricuspid regurgitation, valve replacement remains common, particularly in adults. Diagnostic methods are often needed to assess the function of the prosthesis. Echocardiography is the first-line method for noninvasive evaluation of prosthetic valve function. The transthoracic approach is complemented with two-dimensional and threedimensional transesophageal echocardiography for further refinement of valve morphology and function when needed. More recently, advances in computed tomography and cardiac magnetic resonance have enhanced their roles in evaluating valvular heart disease. This document offers a review of the echocardiographic techniques used and provides recommendations and general guidelines for evaluation of prosthetic From the Houston Methodist Hospital, DeBakey Heart & Vascular Center, Houston, Texas (W.A.Z., M.C.-P., S.H.L.); Ann & Robert H. Lurie Children’s Hospital of Chicago, Northwestern University Feinberg School of Medicine, Chicago, Illinois (P.-N.J.); Hospital of the University of Pennsylvania, Perelman Center for Advanced Medicine, Philadelphia, Pennsylvania (T.C.); Northwestern Medicine Healthcare, Chicago, Illinois (K.A.C.); Heart and Vascular Institute, Cleveland Clinic, Cleveland, Ohio (M.Y.D.); Baylor Scott & White Health, University of Texas Southwestern, Dallas, Texas (P.G.); UC Health Heart and Vascular Center, University of Colorado Anschutz Medical Campus, Aurora, Colorado (D.W.G.); Columbia Structural Heart & Valve Center, Columbia University Irving Medical Center, New York, New York (R.T.H.); Heart & Vascular Imaging Services, University of Chicago Medical Center, Chicago, Illinois (E.K.); Massachusetts General Hospital, Boston, Massachusetts (D.S.); VCU Pauley Heart Center, Virginia Commonwealth University, Richmond, Virginia (S.B.S.); Northwell Health Physician Partners Cardiology, North Shore University Hospital, Manhasset, New York (L.S.); Cardiothoracic Anesthesiology and Critical Care Medicine, Duke University, Durham, North Carolina (M.S.); Department of Cardiovascular Medicine, Mayo Clinic, Rochester, Minnesota (J.T.); Toronto General Hospital, University Health Network, Toronto, Ontario, Canada (P.T.); Toronto General Hospital Research Institute, University of Toronto, Toronto, Ontario, Canada (W.T.); Department of Radiology, University of Cambridge School of Clinical Medicine, Cambridge, United Kingdom (J.R.W.-M.); and Anschutz Medical Campus, University of Colorado School of Medicine, Aurora, Colorado (E.G.). The following authors reported no actual or potential conflicts of interest in relation to this document: William A. Zoghbi, MD, Pei-Ni Jone, MD, Mohammed A. Chamsi-Pasha, MD, Tiffany Chen, MD, Keith A. Collins, MS, RDCS, Milind Y. Desai, MD, MBA, Daniel W. Groves, MD, Stephen H. Little, MD, Eric Kruse, RDCS, 2 Danita Sanborn, MD, Sangeeta B. Shah, MD, Madhav Swaminathan, MD, MBBS, Paaladinesh Thavendiranathan, MD, SM, Jonathan R. Weir-McCall, MD, MBChB, PhD, and Edward Gill, MD, FASE. The following authors reported relationships with one or more commercial interests: Rebecca T. Hahn, MD, has participated on speakers bureaus for Edwards Lifesciences, Philips Healthcare, and Abbott Vascular and on advisory boards for Abbott Vascular, Boston Scientific, and Edwards Lifesciences. Lissa Sugeng, MD, has participated on speakers bureaus for Siemens Healthineers and Philips Healthcare. Paul Grayburn, MD, has participated on advisory boards for Abbott Vascular and Edwards Lifesciences. Wendy Tsang, MD, SM, has participated in equipment research for Philips Healthcare. Jeremy Thaden, MD, has participated in Medtronic trials for assessing valves. Reprint requests: American Society of Echocardiography, Meridian Corporate Center, 2530 Meridian Parkway, Suite 450, Durham, NC 27713 (E-mail: ase@asecho.org). Attention ASE Members: Login at www.ASELearningHub.org to earn continuing medical education credit through an online activity related to this article. Certificates are available for immediate access upon successful completion of the activity and postwork. This activity is free for ASE Members, and $40 for nonmembers. 0894-7317/$36.00 Copyright 2023 Published by Elsevier Inc. on behalf of the American Society of Echocardiography. https://doi.org/10.1016/j.echo.2023.10.004 Zoghbi et al 3 Journal of the American Society of Echocardiography Volume 37 Number 1 valve function on the basis of the scientific literature and consensus of a panel of experts. This guideline discusses the role of advanced imaging with transesophageal echocardiography, cardiac computed tomography, and cardiac magnetic resonance in evaluating prosthetic valve structure, function, and regurgitation. It replaces the 2009 American Society of Echocardiography guideline on prosthetic valves and complements the 2019 guideline on the evaluation of valvular regurgitation after percutaneous valve repair or replacement. (J Am Soc Echocardiogr 2024;37:2-63.) Keywords: Echocardiography, Doppler echocardiography, Prosthetic valves, Cardiac valves, Magnetic resonance imaging, Computed tomography This document is endorsed by the following ASE International Alliance Partners: Argentine Federation of Cardiology; Argentine Society of Cardiology; ASEAN Society of Echocardiography; Australasian Society for Ultrasound in Medicine; Australasian Sonographers Association; British Heart Valve Society; British Society of Echocardiography; Canadian Society of Echocardiography; Cardiovascular Imaging Society of the Inter-American Society of Cardiology; Chinese Society of Echocardiography; Echocardiography Section of the Venezuelan Society of Cardiology; Indian Academy of Echocardiography; Indonesian Society of Echocardiography; Interventional Imaging Group of the Saudi Arabian Cardiac Interventional Society; Iranian Society of Echocardiography; Italian Society of Cardio-Thoracic Anesthesia and Intensive Care; Japanese Society of Echocardiography; Korean Society of Echocardiography; National Association of Cardiologists of Mexico, AC; Philippine Society of Echocardiography, Inc.; Vietnamese Society of Echocardiography. TABLE OF CONTENTS J. Other Techniques for Assessing PHVs I. General Considerations With Prosthetic Valves A. Types of Prosthetic Valves 4 B. PHV Dysfunction 5 i. SVD i. Cine fluoroscopy 4 ii. Cardiac catheterization iii. CT 6 c. Other nonstructural causes of dysfunction iii. Endocarditis 6 6 i. Clinical information 7 ii. Echocardiographic imaging 7 iii. Doppler echocardiography 7 b. Effective orifice area E. F. G. H. I. 7 7 i. Intraoperative echocardiography during prosthetic valve placement 11 ii. Image guidance during percutaneous prosthetic valve replacement 11 a. Two-dimensional and 3D TEE 17 iv. Considerations for TAVI and ViV 18 B. Echocardiographic and Doppler Evaluation of Prosthetic Aortic Valve Regurgitation 18 c. Doppler velocity index 9 Pressure Recovery: Hemodynamic Conditions and Clinical Implications 9 Prosthesis-Patient Mismatch 10 Physiologic Regurgitation 10 Pathologic Prosthetic Regurgitation 10 Changes During Stress 11 Considerations for Intraoperative and Intraprocedural Guidance 11 b. TAVI 16 ii. TEE 17 iii. Doppler echocardiography a. Determination of gradients across prosthetic valves D. i. TTE 6 iv. Thrombus 6 C. Evaluation of Prosthetic Valves 13 v. Cardiac positron emission tomography (PET) 14 II. Evaluation of Prosthetic Aortic Valves 16 A. Echocardiographic and Doppler Evaluation of Prosthetic Aortic Valve Function 16 ii. Nonstructural valve dysfunction 6 a. Prosthesis-patient mismatch 6 b. Paravalvular leak 13 13 iv. CMR 6 13 13 i. Stenosis 12 d. Tricuspid valve repair or replacement for native tricuspid regurgitation (TR) 13 20 21 ii. Regurgitation 21 D. Role of CMR in the Evaluation of Prosthetic Aortic Valves i. Prosthetic aortic valve stenosis a. Anatomic valve area 21 b. Phase-contrast imaging 21 22 a. Phase-contrast imaging 22 III. Evaluation of Prosthetic Mitral Valves 23 A. Types of Prosthetic Valves in the Mitral Position 23 B. Echocardiographic Evaluation of Prosthetic Mitral Valves i. Evaluation of prosthetic mitral valve function 24 24 25 iii. Role of TEE 25 C. Role of CT in the Evaluation of Prosthetic Mitral Valves i. Valve stenosis 25 ii. Valve regurgitation 26 21 21 ii. Prosthetic aortic valve regurgitation ii. Evaluation of prosthetic MR 11 11 c. Mitral valve repair or replacement i. TTE and TEE 18 ii. Doppler echocardiography 20 C. Role of CT in the Evaluation of Prosthetic Aortic Valves 25 4 Zoghbi et al Abbreviations 2D = Two-dimensional 3D = Three-dimensional 4D = Four-dimensional AR = Aortic regurgitation ASE = American Society of Echocardiography CHD = Congenital heart disease CMR = Cardiac magnetic resonance CT = Computed tomography CW = Continuous-wave DVI = Doppler velocity index EOA = Effective orifice area EROA = Effective regurgitant orifice area FDA = US Food and Drug Administration ICE = Intracardiac echocardiography LV = Left ventricular LVOT = Left ventricular outflow tract MR = Mitral regurgitation PA = Pulmonary artery PET = Positron emission tomography PHT = Pressure half-time PHV = Prosthetic heart valve PPM = Prosthesis-patient mismatch PR = Pulmonary regurgitation PVL = Paravalvular leak PVR = Pulmonary valve replacement PW = Pulsed-wave RA = Right atrial RV = Right ventricular RVOT = Right ventricular outflow tract SAVR = Surgical aortic valve replacement SSFP = Steady-state free precession SVD = Structural valve dysfunction Journal of the American Society of Echocardiography January 2024 D. Role of CMR in the Evaluation of Prosthetic Mitral Valves 26 i. Valve stenosis 26 ii. Valve regurgitation 28 IV. Evaluation of Prosthetic Pulmonary Valves 30 A. Surgical and Transcatheter PVR 30 B. Evaluation of Prosthetic Pulmonary Valve Stenosis 30 i. Echocardiographic and Doppler evaluation 30 ii. Role of TEE and 3D 31 iii. Role of CMR 31 iv. Role of CT 32 C. Evaluation of Prosthetic Pulmonary Valve Regurgitation 34 i. Echocardiographic and Doppler evaluation 34 ii. Role of TEE and 3D 35 iii. Role of CT 35 iv. Role of CMR 35 V. Evaluation of Prosthetic TVs 36 A. Echocardiographic Assessment of Prosthetic TV Function 37 B. Evaluation of Prosthetic TV Stenosis 37 i. Echocardiographic evaluation 37 ii. Role of CT 40 iii. Role of CMR 40 C. Evaluation of Prosthetic TV Regurgitation 40 i. Echocardiographic evaluation 40 ii. Role of CMR 40 iii. Role of CT 40 VI. Evaluation of Prosthetic Valves in CHD 41 A. Prosthetic Valves in CHD 41 B. Echocardiography in the Evaluation of PHVs Associated With CHD 42 i. TTE 42 ii. Stress echocardiography 42 iii. TEE 42 iv. Three-dimensional echocardiography 42 C. Role of Cardiac CT 42 D. Role of CMR 43 VII. Conclusions and Future Directions 43 VIII. Appendix 51 In patients with significant valvular disease, intervention with either valve repair or replace- ment is often required. Despite advances in valve repair, valve valve implantation replacement remains common, TEE = Transesophageal particularly in adults. The first echocardiography American Society of Echocardiography (ASE) guideTTE = Transthoracic line for the evaluation of prosechocardiography thetic heart valves (PHVs) was TR = Tricuspid regurgitation published in 2009.1 Subsequently, there has been a TV = Tricuspid valve European Association of TVR = Tricuspid valve Cardiovascular Imaging guideline replacement on prosthetic valves in 20162 VC = Vena contracta and an ASE guideline in 2019 on the evaluation of valvular regurgiViV = Valve-in-valve tation after percutaneous valve VTI = Velocity-time integral repair or replacement.3 Although many principles and recommenVTIPrMV = Prosthetic mitral dations detailed in the 2009 ASE valve velocity-time integral guideline are still current and valid, it lacks several important developments: function of percutaneous valves, the use of three-dimensional (3D) echocardiography, and the role of computed tomography (CT) and cardiac magnetic resonance (CMR) in the evaluation of PHVs. With the evolution of structural heart disease interventions and imaging of valvular heart disease, a comprehensive update is necessary. The present document replaces the 2009 ASE guideline and complements the 2019 guideline on valvular regurgitation after percutaneous valve repair or replacement.1,3 TAVI = Transcatheter aortic I. GENERAL CONSIDERATIONS WITH PROSTHETIC VALVES A. Types of Prosthetic Valves A wide variety of PHV types and sizes are available, with selection dependent upon implantation location, underlying valvular pathology, implantation technique, and patient-specific factors. Although percutaneous valves are bioprosthetic, surgically implanted prosthetic valves can be either bioprosthetic or mechanical, with the latter associated with greater durability4 but necessitating chronic anticoagulation. The shared decision-making surrounding valve choice and implantation technique must integrate patient anatomy, procedural risk, expected patient longevity, the expected PHV durability, and patient preferences and lifestyle.5 The prevalence of mechanical valve implantation has declined over the past 10 years for several reasons, including patient preference. Transcatheter valve repair and replacement have changed the demographics and clinical characteristics of patients undergoing surgical valve replacements.6,7 The need for concurrent procedures such as aortic root and ascending aorta modification, as well as left ventricular outflow tract (LVOT) or right ventricular outflow tract (RVOT) alteration may also affect PHV choice. The most common type of mechanical valve is the bileaflet tilting disk valve (e.g., St. Jude Medical, Carbomedics, On-X), which offers the best hemodynamics of currently available mechanical valves.8 Single tilting disk valves with low thrombogenicity (e.g., Medtronic-Hall) are infrequently used in contemporary practice. Last, the Starr-Edwards ball-in-cage valve is no longer implanted; however, given its durability, some of these valves continue to function satisfactorily and may be encountered in clinical practice. Examples of Journal of the American Society of Echocardiography Volume 37 Number 1 Zoghbi et al 5 Figure 1 Mechanical valves: (A) bileaflet, (B) single-leaflet, and (C) caged-ball valves and their 2D and 3D transesophageal echocardiographic characteristics taken in the mitral position in diastole and systole (second and third panels from left). The arrows in diastole point to the open occluder mechanism of the valve and in systole to the characteristic physiologic regurgitation observed with each valve. Three-dimensional transesophageal echocardiography images (fourth panel) from a midesophageal window are displayed from a left atrial view. LA, Left atrium; LV, left ventricle. mechanical prosthetic valves are depicted in Figure 1 and examples of stented and percutaneous bioprosthetic valves in Figure 2. Surgical bioprosthetic valves may be xenografts comprising porcine or bovine pericardial tissue, homografts from cadaveric donors, or autografts (such as in the Ross procedure). Stented xenografts are most frequently used; the pericardial leaflets are mounted onto either the inside or outside of a stent frame. Externally mounted leaflets and stentless bioprostheses have the advantage of larger valve areas and lower transvalvular gradients but recent studies show high rates of early structural valve dysfunction (SVD), particularly in younger patients.9 In the setting of SVD, transcatheter valve-in-valve (ViV) procedures offer patients an alternative to surgical reoperation.10 Although the risk for coronary obstruction with externally mounted leaflets as well as stentless valves following a ViV procedure is greater than for internally mounted bioprosthetic valves, percutaneous leaflet laceration procedures may mitigate this risk. Different bioprosthetic valves can often be identified by the fluoroscopic and computed tomographic appearance of the stent posts’ configuration and sewing ring. Transcatheter heart valve technology has continued to evolve with expanding indications.5 Transcatheter aortic valve implantation (TAVI) prostheses in commercial use include balloon-expandable intraannular devices (e.g., SAPIEN valves; Edwards Lifesciences), selfexpanding supra-annular valves (e.g., Evolut valves; Medtronic), and intra-annular valves (Navitor valves; Abbott Structural Heart). Other TAVI prostheses are in trials or early human use. On the other hand, several mitral and tricuspid transcatheter valves are currently under clinical investigation. These feature a wide variety of designs and anchoring mechanisms, including radial force, leaflet capture, annular engagement, and apical tethering. In addition, a ViV transcatheter mitral valve implantation with a balloon-expandable TAVI prosthesis is feasible and has US Food and Drug Administration (FDA) approval. The SAPIEN valve has also been approved for implantation in the pulmonary position. Last, the self-expanding Harmony valve (Medtronic) recently received breakthrough device designation from the FDA and is also available for treatment of pediatric or adult patients with severe pulmonary regurgitation (PR). From an imaging standpoint, the type, position, and size of a prosthetic valve influence its hemodynamic profile and rate of complications. Normal transvalvular velocities and gradients are flow dependent but can vary depending on the particular valve size and type.11,12 The valve type also affects the amount of artifact seen with echocardiography, CT, and CMR, which may affect the evaluation of PHV function. Normal echocardiographic parameters of valve function for various prosthetic valve types and sizes in the aortic, mitral, pulmonary, and tricuspid positions are detailed in Appendix Tables A1-A9. B. PHV Dysfunction Prosthetic valve dysfunction can be divided into the following categories: SVD, nonstructural valve dysfunction, endocarditis, and thrombus.13 Regardless of etiology, the hemodynamic consequences 6 Zoghbi et al Journal of the American Society of Echocardiography January 2024 Figure 2 Biological valves: stented (top row) and percutaneous valves with their echocardiographic features and 3D transesophageal echocardiographic images. The self-expanding percutaneous valve is in the middle row, and the balloon-expandable valve is in the bottom row. Mild paravalvular regurgitation is highlighted by the arrows in the middle panels. LA, Left atrium; LV, left ventricle. of dysfunction must be quantified. The following definitions are derived from the Valve Academic Research Consortium 313: i. SVD: intrinsic permanent changes to the prosthetic valve. Examples include wear and tear, leaflet disruption, leaflet fibrosis or calcification, and stent or strut fracture or deformation. Structural failure is more common in bioprosthetic than mechanical prostheses. Valve calcification is the most common cause of bioprosthesis degeneration, seen in 50% of porcine valves at 5 years and in 75% at 8 years.14 Failure rates at 10 to 15 years are 10% to 20% in homografts and 30% in heterografts.15 The leaflets and stents are the primary sites with calcification and leaflet tear or rupture. ii. Nonstructural valve dysfunction: any abnormality of the prosthesis not related to the valve itself but still resulting in valve dysfunction. a. Prosthesis-patient mismatch (PPM) occurs when a normally functioning PHV is small relative to the patient’s size, causing a high gradient and functional stenosis. Outcomes have been related to the severity of PPM. b. Paravalvular leak (PVL) may occur in surgical valves from dehiscence of the sewing ring and for transcatheter valves from malapposition of the stent frame with native tissue. Dehiscence is a serious complication, with 4.9% of aortic PHVs requiring reoperation or catheter-based intervention compared with 2.0% of mitral PHVs. Risk factors for dehiscence include bacterial endocarditis, surgical technique, ascending aortic aneurysm, degenerative regurgitation, and severe calcification of the native valve. Transcatheter PVL is related to multiple factors, including missizing of the device, bulky calcification of leaflet or annulus, underdeployment of the transcatheter valve, or improper implantation depth.13 c. Other nonstructural causes of dysfunction: Other causes of dysfunction include leaflet entrapment or dysfunction from pannus, inappropriate position or sizing, dilatation of the cardiac chambers after implantation (e.g., aortic root dilatation, mitral annular or left atrial) dilatation), and valve embolization. Pannus is fibrous tissue that grows in the periannular region and can cause PHV dysfunction.16 Pannus has a prevalence of 0.2% to 4.5% and occurs equally in mechanical and bioprosthetic valves, with three times higher risk in the mitral position.17 Pannus may coexist with thrombus formation in PHVs. iii. Endocarditis has a prevalence of 1% to 6% and can occur any time after surgery. In mechanical valves, the infection almost always spreads from the sewing ring and results in complications such as PVL, abscess, and extension to adjacent structures. Bioprosthetic valve infections originate in the leaflet cusps and may involve the sewing ring or paravalvular region. Paravalvular abscess is more common in PHVs (56%-100%) than in native valves (10%-40%), especially in the aortic position.18,19 Pseudoaneurysms are commonly seen in the aortic position, with a prevalence of 7% to 25% of prosthetic valve endocarditis.18-20 An infected pseudoaneurysm in relation to a PHV refers to drainage of a paravalvular abscess into an adjacent cardiac chamber. An abnormal communication such as a fistula can occur between two neighboring cavities through a perforation from the infection that extends beyond the valve.18,19 Last, endocarditis after TAVI is an increasingly important consideration in the appropriate clinical setting, given the increasing number of TAVI prostheses implanted.21 iv. Thrombus is seen in 0.3% to 8% of PHVs.2 Mechanical valves are more thrombogenic than bioprosthetic valves, although the risk for thrombus for a mechanical valve with appropriate anticoagulation therapy is similar to that of a bioprosthetic valve. Right-sided valves are more vulnerable to thrombosis than left-sided valves, with the tricuspid valve (TV) affected 12 to 20 times more frequently than left-sided valves.22 Thrombus is seen on echocardiography as a mass on the valve with a soft echodensity that can be associated with intracardiac thrombus16; in bioprosthetic valves, it may appear as valve thickening.23 On CT, thrombus on bioprosthetic valves may manifest as hypoattenuated leaflet thickening, characterized by thickened and hypoattenuating Journal of the American Society of Echocardiography Volume 37 Number 1 Table 1 Essential clinical and echocardiographic parameters in the comprehensive evaluation of prosthetic valve function Parameters Clinical information Date of valve replacement Type and size of the prosthetic valve Height/weight/body surface area Symptoms and related clinical findings Blood pressure and heart rate Echocardiography Opening and closing of leaflets or occluder Presence of leaflet thickening, calcifications, or abnormal echo density(ies) on the various components of the prosthesis or adjacent to prosthesis Valve sewing ring or stent integrity and stability Position of sewing ring or stent frame Doppler echocardiography of the valve Contour of the jet velocity signal Peak velocity and gradient Mean pressure gradient VTI of the jet DVI Acceleration time, acceleration time/ ejection time for AV PHT in MV and TV EOA* Presence, location, and severity of regurgitation† Other echocardiographic data LV and RV size, function, and hypertrophy Left atrial and RA size and function Concomitant valvular disease Estimation of PA pressure Venous inflow pattern (i.e., pulmonary vein for MV and hepatic vein for TV) Previous postoperative study(ies), when available Comparison of above parameters is particularly helpful in suspected prosthetic valvular dysfunction AV, Aortic valve; MV, mitral valve. *EOA using the continuity equation; must be compared with normal Doppler values of the valve type and size. † Transthoracic Doppler is less sensitive for detection of valvular regurgitation in mitral and tricuspid prosthesis; TEE is frequently needed for a more definitive assessment. PHV leaflets and reduced valve motion (hypoattenuation affecting motion). The reported prevalence is 3.6% to 40%.24 C. Evaluation of Prosthetic Valves A comprehensive assessment of prosthetic valve function includes clinical information, echocardiography, and Doppler evaluation. Comparison with a baseline study or serial postoperative studies is key to determining whether valve function has changed (Table 1). i. Clinical information: Study indications, patient symptoms, size and type of valve replacement, and date of surgery should be included in the report when Zoghbi et al 7 available. This allows comparison of the study measurements with the expected normal PHV hemodynamics. Similarly, blood pressure, heart rate, height, weight, and body surface area should be included. Heart rate affects the duration of diastolic filling and therefore mean gradients in the mitral valve and TV; body surface area is helpful in assessing the presence of PPM and chamber size. ii. Echocardiographic imaging: Standardized measurements of cardiac chambers, systolic and diastolic function, aortic root, and ascending aorta per ASE guidelines are recommended in patients with PHVs. Zoom imaging with multiple views should be used to evaluate all components of the prosthetic valve (Table 1). Because of acoustic reverberation by prosthetic material, visualizing the central occluder or leaflets may require off-axis imaging. Biplane imaging allows simultaneous assessment of the valve structure in real time and localization of paravalvular regurgitation with color Doppler. Mild thickening is often the first sign of primary failure of a biologic valve and is a signal to reduce the interval between follow-up studies. Independent or rocking motion of a replacement valve is a sign of dehiscence and may be more diagnostic for valves in the aortic position.25 In the mitral position, normal increased mobility of a valve may be due to annular motion, atrial or annular reconstruction, or location of the sewing ring (i.e., within the left atrium); it needs to be differentiated from dehiscence by the absence of a PVL. Thickening of the aortic root due to hematoma and edema after insertion of a stentless valve usually resolves in 3 to 6 months but can be mistaken for an aortic root abscess. Reviewing the postoperative or intraoperative study is useful to corroborate this finding. Note that careful attention to the possibility of abscess formation is needed at the level of the annulus or sewing ring.25 When using 3D echocardiography, the prosthesis should be assessed via 3D volume data sets, with and without color Doppler, from the imaging view that best visualizes the valve or paravalvular structures. The en face view of prosthetic valves allows easier localization of PVL and guidance of percutaneous interventions. When acquiring 3D data sets, the two-dimensional (2D) multiplanar images should be used to optimize line density and frame rate, allowing an accurate assessment of spatial and temporal changes. This may be achieved with single-beat narrow volumes using live 3D modes or multibeat acquisition using live 3D, zoom, or full-volume modes, preferably with volume rates surpassing 20 Hz. If measurements are performed using 3D volumes, high–volume rate single-beat acquisitions are preferred. However, if 3D color Doppler is required to quantify the vena contracta (VC) area, then a multibeat acquisition may be necessary to improve line density and volume rate. Optimal 3D acquisitions will include surrounding tissue and valvular landmarks so that the location of the lesion may be referenced and displayed in accordance with the ASE and European Association of Echocardiography guidelines.26 iii. Doppler echocardiography: The principles of interrogation and recording flow velocity through prosthetic valves using pulsed-wave (PW), continuous-wave (CW), and color Doppler are similar to those used in assessing native valve function. a. Determination of gradients across prosthetic valves: Velocity across a prosthetic valve is dependent on flow, valve size, and valve type. The simplified Bernoulli equation (DP = 4V2) is key to the noninvasive calculation of pressure gradients. In patients with aortic prostheses and high cardiac output or narrow LVOT in whom the proximal velocity (V1) is >1.5 m/sec, the proximal velocity can no longer be ignored, and estimation of the pressure gradient is DP = 4(V22 ! V12). In bileaflet prostheses and caged-ball valves, however, overestimation of the gradient may occur more than in bioprosthetic valves, particularly with smaller valves and high cardiac output (see ‘‘Pressure Recovery: Hemodynamic Conditions and Clinical Implications’’; Figure 3).27,28 b. Effective orifice area (EOA): The prosthetic valve EOA derived using the continuity equation is a better index of valve function than gradient alone as it is less dependent on flow through the valve: EOA = stroke volume/prosthetic valve velocity-time integral (VTI). For stroke volume calculation using the LVOT, the LVOT diameter measurement and the corresponding position of the PW Doppler sample volume introduce the largest errors in estimating EOA.1,2 The diameter used should always be the largest diameter measured perpendicular to the LVOT direction, not an average determination, as the error is in 8 Zoghbi et al Journal of the American Society of Echocardiography January 2024 Figure 3 Pressure recovery in prosthetic valves. Schematics of changes in velocity and pressure in prosthetic aortic valves. Velocities are lower and systolic arterial pressure (SAP) is higher at the distal aorta than at the level of the VC. The left figure represents changes in velocity and pressure from the LV outflow to the ascending aorta (AA) in a stented bioprosthetic valve. As flow expands into the wider lumen beyond a valve, velocity and kinetic energy decrease and pressure recovers. The magnitude of this phenomenon is small, except in patients with aortas