HSC1007 Anatomy and Physiology 1 Cardiovascular Physiology PDF
Document Details
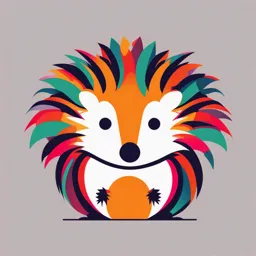
Uploaded by stohhh
Singapore University of Technology and Design
null
Andy Lee (PhD)
Tags
Related
- Cardiovascular Physiology Primer HBY501 PDF
- Cardiovascular Physiology PDF
- Cardiovascular Physiology (Electrical Properties of the Heart) PDF
- Cardiovascular Physiology: Heart as a Pump PDF
- Cardiovascular Physiology - Rhythmical Excitation of the Heart PDF
- Forrester Cardiovascular Physiology Fall 2024 PDF
Summary
These lecture notes cover the basics of cardiovascular physiology, focusing on the electrical basis of the heart and the ECG. The document includes learning outcomes and concepts of homeostasis, presenting information in a structured format for students.
Full Transcript
HSC1007 ANATOMY AND PHYSIOLOGY 1 CARDIOVASCULAR PHYSIOLOGY ELECTRICAL BASIS OF THE HEART AND ECG ANDY LEE (PHD) ASSISTANT PROFESSOR FACULTY HALL #04-17 OFFICE PHONE: 6592 2524 [email protected] LEARNING OUTCOMES At the end of the lesson, you should be able to: Describe the general f...
HSC1007 ANATOMY AND PHYSIOLOGY 1 CARDIOVASCULAR PHYSIOLOGY ELECTRICAL BASIS OF THE HEART AND ECG ANDY LEE (PHD) ASSISTANT PROFESSOR FACULTY HALL #04-17 OFFICE PHONE: 6592 2524 [email protected] LEARNING OUTCOMES At the end of the lesson, you should be able to: Describe the general function of the heart Understand the electrical activity of the heart Explain how the autorhythmic cells is responsible for the function of the heart Understand how cardiac excitation is coordinated for efficient cardiac function Describe how heart rate can be controlled by the nervous system Understand the electrical basis of the ECG Describe how the 12-lead ECG is derived Relate a prototypical normal ECG waveform to events of the cardiac cycle CONCEPT OF HOMEOSTASIS How can a cell in our body, a cardiac myocyte etc, get its nutrients, oxygen and eliminates its metabolic wastes? Internal environment of the body Fluid that surrounds the cells through which life- sustaining exchanges are made Intracellular fluid Extracellular fluid Fig. 1-6, Sherwood SO WHAT IS HOMEOSTASIS? Maintenance of a relatively stable internal environment Dynamic steady state 37.2°C 37.0°C 36.7°C HOMEOSTATIC REGULATION To maintain homeostasis, the body must be able to 1. Detect deviations from normal 2. Integrate this information with other information 3. Make adjustments to restore the factor to normal DETECT INTEGRATE ADJUST Example of a Negative Feedback Deviation out of the set-point triggers a response to restore the factor to normalcy by moving the factor in the opposite direction of the change (REVISION) ANATOMY Systemic circulation (REVISION) ANATOMY Capillary networks of upper body Circulatory system Systemic arteries (to upper body) 1. Heart, 2. Blood Pulmonary Pulmonary circulation circulation Vessels, 3. Blood Systemic veins Aorta Pulmonary Pulmonary artery artery Pulmonary Pulmonary Pulmonary circulation vein vein Capillary network Capillary network of right lung Systemic Systemic of left lung veins arteries (to lower body) Capillary networks of lower body Systemic circulation KEY O2-rich blood Systemic O2-poor blood circulation Fig. 9-1, Sherwood (REVISION) ANATOMY Fig. 9-2, Sherwood FUNCTION OF THE HEART Major function is to service metabolic needs of tissues Achieved by ensuring adequate exchange of fluids at the capillaries Sufficient pressure and output Integrity of vessels ELECTRICAL AND MECHANICAL ACTIVITY OF THE HEART ELECTRICAL ACTIVITY OF THE HEART Intrinsic electrical network of heart ensure coordinated contraction and relaxation Specialized myocardial fibers (not motor nerves) Auto-rhythmicity Contractile cells: 99% of the cardiac muscle cells do the mechanical work of pumping Autorhythmic cells: initiate and conduct the action potentials responsible for contraction of working cells Fig. 20-10, Martini PACEMAKER CELLS Sinoatrial node (SA node) Normal pacemaker of the heart R atria wall near opening of superior vena cava Atrioventricular node (AV node) Junction between atria and ventricles, near opening of the coronary sinus Bundle of His (Atrioventricular bundle) Specialised tract originating from AV node and travels down interventricular septum Purkinje fibers Terminal fibers spreading through the myocardium like tree branches Fig. 20-10, Martini PACEMAKER CELLS Interatrial Interatrial pathway pathway Sinoatrial Atrioventricular (SA) node (AV) node SA node AV node Right atrium Left atrium Right atrium Left atrium Internodal Left branch Internodal Bundle pathway of bundle of pathway of His His Electrically Left ventricle nonconductive Right ventricle Left ventricle fibrous tissue Purkinje Right ventricle Right branch Purkinje of bundle fibers fibers of His (a) Specialized conduction system of the heart (b) Spread of cardiac excitation Fig. 9-8, Sherwood IMPULSE CONDUCTION THROUGH THE HEART Fig. 20-11, Martini PACEMAKER POTENTIAL AND ACTION POTENTIAL IN AUTORHYTHMIC CELL What is an action potential? Pacemaker ability - slowly depolarize until threshold is reached Note that the generation of AP in the heart is slightly different from that in the nervous system Fig. 9-7, Sherwood PACEMAKER ACTIVITY Rate of action potential generation SA node – 70 to 80 AP per min AV node – 40 to 60 AP per min Bundle and Purkinje – 20 to 40 AP per min Fastest is SA node, hence it sets the pace Others (AV, bundle and purkinje) could not assume their naturally slower rates Activated by AP from SA node before they can reach threshold at their slower rhythm Once an AP occurs in any cardiac muscle cell, it propagates throughout the myocardium AP ---> contraction PACEMAKER ACTIVITY Fig. 9-9, Sherwood EFFICIENT CARDIAC FUNCTION 1. Atrial excitation and contraction should be complete before ventricular contraction onset Prime main pumps (ventricles) by fully filling them before they pump Slight delay at AV node 2. Excitation of cardiac muscle fibers should be coordinated to ensure each heart chamber contracts as a unit to pump efficiently 3. The pair of atria and pair of ventricles should be functionally coordinated so that both members of the pair contract simultaneously CONTROL OF HEART RATE Cardiovascular control center Pain, chemoreceptors, respiratory center, baroreceptors etc Epinephrine (adrenaline), temperature etc Fig. 20-21, Martini CONTROL OF HEART RATE Autonomic nervous system Sympathetic (S) Parasympathetic (P) Control SA node Resting conditions: S and P both active but P predominates Epinephrine (adrenaline), temperature etc, act directly on SA node independent of ANS Pain, chemoreceptors, respiratory center, baroreceptors etc, act on the cardiovascular control center CONTROL OF HEART RATE HR + ─ Sympathetic Parasympathetic ↑ ↑ ↑ HR ↓ HR Sympathetic Parasympathetic Sympathetic Parasympathetic ↑ ↓ ↓ ↑ ELECTROCARDIOGRAM (ECG) ELECTROCARDIOGRAM (ECG) ELECTRICAL BASIS OF THE ECG Record of electrical events in heart from the surface of the body Provide information on the cardiac rate, rhythm, pattern of depolarization, and presence of cardiac ischemia or infarction etc. Note: Not a direct recording of the actual electrical activity of the heart! Overall spread of electrical activity throughout the heart during depolarization and repolarization Sum of electrical activity Differential recording – potential difference between 2 electrodes 12 LEAD ECG Fig. 9-13, Sherwood 12 LEAD ECG 12 LEAD ECG Each lead forms an axis in vertical or horizontal plane Each lead look at the electrical events in the heart from a unique vantage point (spatial location) Lead II directly at apex of the left ventricle V2, V3, V4 horizontal looks at anterior part of left ventricle 12 LEAD ECG Can help to localized infarction from the 12 lead ECG readout as each looks at the heart from a different spatial location Relate the parts of heart and then to the coronary artery Anatomical relationship of leads example Inferior wall—Leads II, III, and aVF Anterior wall—Leads V1 to V4 Lateral wall—Leads I, aVL, V5, and V6 TYPICAL LEAD II ECG WAVEFORM Fig. 11-1, Guyton mV over time Higher muscle mass depolarizing – higher voltage Normal rate at which ECG strip is run = 25mm/s TYPICAL LEAD II ECG WAVEFORM Fig. 9-14, Sherwood ELECTRICAL BASIS OF THE ECG Where is the SA node firing? Firing of SA node does not generate sufficient electrical activity to reach body surface, so not recorded. The P wave is the first wave to be sensed, when the impulse spreads through the atria Where is the wave for atrial repolarisation? Same time as ventricular depolarization, masked by QRS Why P wave smaller? Atria have smaller muscle mass than ventricles What are the 3 occasions where there is no electrical activity? AV node delay; PR Ventricles completely depolarised, and cardiac cells undergoing plateau phase (ventricles contracting); ST Heart muscle at rest, ventricles filling AV NODE DELAY PR interval: 0.12s – 0.20s (~0.16s), AV node delay Time for signal to travel from SA node to AV node, AV node delay, then travel out to bundle of his. Bulk of time is the AV Fig. 11-1, Guyton node delay, hence taken as AV node delay RR interval: time for 1 cardiac cycle ABNORMAL RATE AND RHYTHM Fig. 9-15, Sherwood ABNORMAL RATE AND RHYTHM Fig. 9-15, Sherwood CALCIUM AND CARDIAC MUSCLE CONTRACTION The action potential of cardiac contractile cells shows a characteristic plateau Plateau phase: membrane potential is maintained close to this peak positive level for several hundred milliseconds Opening of the L-type Ca2+ channels results in a slow, inward diffusion of Ca2+. This continued influx of positively charged Ca2+ prolongs the positivity inside the cell and is primarily responsible for the plateau part of the action potential. Fig. 9-10, Sherwood CALCIUM AND CARDIAC MUSCLE CONTRACTION Calcium entry from the ECF Induces a much larger Ca2+ release from the sarcoplasmic reticulum Extra supply of Ca2+ release with slow Ca2+ removal is responsible for the long period of cardiac contraction Increased contraction ensures adequate time to eject the blood fully Fig. 9-11, Sherwood REFERENCES Chapter 9 Sherwood, L. (2016) Human Physiology: From Cells to Systems. 9th edition. Cengage Chapter 20 Martini, F. H., Nath, J.L., & Bartholomew, E. F. (2018). Fundamentals of Anatomy and Physiology. 11th Global Edition. Pearson. HSC1007 ANATOMY AND PHYSIOLOGY 1 CARDIOVASCULAR PHYSIOLOGY CARDIAC CYCLE AND OUTPUT ANDY LEE (PHD) ASSISTANT PROFESSOR FACULTY HALL #04-17 OFFICE PHONE: 6592 2524 [email protected] LEARNING OUTCOMES At the end of the lesson, you should be able to: Describe the sequence of events that occur during one cardiac cycle Explain how pressures and volumes within the heart chambers change Explain the ECG in terms of the cardiac cycle. Describe and explain when sounds are heard during the cardiac cycle Define cardiac output and describe how it is affected by cardiac rate and stroke volume Explain how autonomic nerves regulate the cardiac rate and the strength of ventricular contraction Explain the intrinsic regulation of stroke volume (the Frank-Starling law of the heart) REVISION AP in Autorhythmic cells (Pacemaker) AP in Cardiac Contractile cells (Pumping) Responsible for autogeneration of AP Responsible for contraction upon (impulses) receiving impulses Related to rate and rhythm of heartbeat Related to the contraction All ions (Na+, K+, Ca2+) are important for All ions (Na+, K+, Ca2+) are important for this to occur this to occur Ca2+ is unique to autorhythmic cells for AP in contractile cells is different from self induction of AP as compared to autorhythmic cells due to the plateau nervous system phase from the slow influx of Ca2+ Cytosolic Ca2+ directly involved in mechanism of contraction Autogeneration Contraction of impulses Impulse spread Autogeneration of REVISION impulses in autorhythmic cells (pacemaker) Impulse spread Action potential in cardiac contractile cells Increase in cytosolic Ca2+ in cardiac Contraction contractile cells REVISION Anatomy of the heart and how it relates to function Electrical activity of the heart Pacemaker What is an ECG How different leads can give you an insight into different regions of the heart Importance of calcium in generation of an action potential in cardiac autorhythmic cells and in the strength of cardiac contraction MECHANICAL EVENTS OF THE CARDIAC CYCLE Systole – Contraction Diastole – Relaxation Fig. 20-16, Martini CARDIAC CYCLE Atrial contraction AV valves open Forces small amount of blood into relaxed ventricles to fill it CARDIAC CYCLE Atria begin to relax after contraction Ventricle begin to contract Forces AV valves to close CARDIAC CYCLE Aortic semilunar valves open Blood ejected CARDIAC CYCLE Ventricles relaxes Semilunar valves close AV valves open Blood flows from atria to ventricles passively 19 19 volume elaxation c ventricular con (mL) 24 Reduced Ventricular Ventricular Isovolumetric Left ventricular 10 filling pressure 40 23 systole diastole (mm Hg) End- 20 Rapid 65 systolic filling 8 volume 15 20 QRS 9 No sound 1st 2nd 1st 2nd 16 16 OVERVIEW OF THE Left atrial pressure 1 4 22 Heart sounds (a) (b) (c) Electrocardiogram (d) (e) (a) (b) (c) (d) 3 P (e) T 25 0 CARDIAC CYCLE (mm Hg) 5 21 Ejection End- Ventricular filling 120 phase 7 11 135 diastolic phase 13 13 volume Left Left ventricular 2 6 14 atrium 18 volume Right 100 17 (mL) atrium 24 Reduced Aortic pressure filling 12 (mm Hg) 23 80 Rapid ventricular relaxation Isovolumetric End- Right 20 65 systolic ventricle filling volume 15 Left ventricular contraction ventricle 60 No sound 1st Passive filling2nd (a) during 1st (b) Atrial 2nd (c) Isovolumetric (d) Ventricular (e) Isovolumetric 19 19 Heart sounds ventricular and atrial diastole contraction ventricular contraction ejection ventricular relaxation Isovolumetric Left ventricular 10 (a) (b) (c) (d) Ventricular (e) (a) filling (b) (c) (d) (All Ventricular emptying 40 (e)valves pressure (All valves (AV valves open; semilunar valves closed) closed) (mm(Semilunar Hg) valves open; closed) AV valves closed) 20 9 Left Left atrial 4 1 (a) Passive filling; atrium pressure (mm Hg) 0 5 22 t 21 m (b) Atrial contraction; End- 7 11 Ventricular filling diastolic phase (c) Isovolumetric 135 volume Left ventricular 2 6 14 ght contraction; volume entricle (mL) 24 Reduced (d) Ventricular Left ventricle ejection; 23 filling (a) (e) PassiveIsovolumetric filling during (b) Atrial (c) Isovolumetric (d) Ventricular (e) Isovolumetric End- 20 Rapid 65 systolic filling ventricular and atrial diastole contraction ventricular contraction ejection ventricular relaxation ventricular relaxation volume 15 Ventricular filling (All valves Ventricular emptying (All valves No sound 1st 2nd 1st 2nd (AV valves open; semilunar valves closed) closed) (Semilunar valves open; closed) Heart sounds Fig. 9-16, Sherwood AV valves closed) (a) (b) (c) (d) (e) (a) (b) (c) (d) (e) 8 QRS 16 16 3 P T PASSIVE FILLING AND ATRIAL CONTRACTION Electrocardiogram Ejection 120 phase 1: Atrial pressure higher than ventricular 13 13 18 pressure, blood flow passively into ventricles Aortic pressure 100 17 12 (mm Hg) 2: Ventricular volume rising 80 ventricular contraction 3: SA node fire and impulse spread through atria 60 1 (P wave) Isovolumetric Left ventricular 10 pressure 40 (mm Hg) 4: Atrial pressure increase 20 9 5,6: Volume in ventricles increase leading to an Left atrial pressure 0 1 4 22 increase in ventricular pressure (mm Hg) 5 21 End- 7 11 V 135 diastolic p 7: Atrial contraction complete. End diastolic Left ventricular volume volume 2 6 14 volume (EDV) ~ 135ml (mL) End- 20 65 systolic volume 15 No sound 1st 2nd Heart sounds VentricularVentric Ventricular systole systole diasto 8 8 QRS QRS VENTRICULAR CONTRACTION 16 16 16 16 3 3 P P T T Electrocardiogram Electrocardiogram Impulse passes through AV node Ejection Ejection phase phase 120 120 8: QRS – ventricular excitation 13 13 13 13 18 18 100 100 9: Sharp increase in ventricular pressure Aortic pressure Aortic pressure 12 12 17 17 (mm Hg)(mm Hg) 1st heart sound: AV valves close 80 80 ventricular relaxation 9-12: Isovolumetric ventricular contraction – All valves close, ventricular contraction ventricular contraction 60 60 no change in volume or muscle length, pressure continue to 19 19 Isovolumetric Isovolumetric Left ventricular Left ventricular increase 10 10 pressurepressure 40 40 (mm Hg)(mm Hg) 12: Ventricular pressure > aortic pressure (aortic valves open 20 20 9 9 and blood ejected) Left atrial Left atrial 1 4 1 4 pressurepressure 0 0 22 22 13: Aortic pressure increase as blood is pumped into aorta (mm Hg)(mm Hg) 5 5 21 2 faster than blood can drain off smaller vessels at the other end End- End- 7 11 7 11 Ventricu 135 diastolic 135 diastolic phase volume volume Left ventricular Left ventricular 14: Ventricular volume decrease 2 2 6 14 6 14 volume volume (mL) (mL) 24 23 End- End- 20 Rap 20 65 65 systolic systolic fillin 15 volume volume 15 No soundNo sound 1st 1st 2nd 2nd Heart sounds Heart sounds Ventricular Ventricular Ventricular Ventricu systole diastole systole diastole 8 8 QRS QRS VENTRICULAR REPOLARIZATION AND DIASTOLE 16 16 16 16 3 3 25 P T P T Electrocardiogram Electrocardiogram 15: End systolic volume (ESV) – blood not Ejection Ejection 120 120 phase phase pumped out totally 13 13 13 13 18 18 100 100 17 17 16: T wave – ventricular repolarisation Aortic pressure Aortic pressure 12 12 (mm Hg) (mm Hg) 80 80 ventricular relaxation Isovolumetric ventricular relaxation Isovolumetric 17: Ventricles relax, pressure drops ventricular contraction ventricular contraction 60 60 19 19 19 19 18 & 2nd heart sound: ventricular pressure Left < ventricular Isovolumetric Isovolumetric Left ventricular 10 10 pressure 40 pressure 40 (mm Hg) (mm Hg) aortic pressure, aortic valve close. Creates a 20 20 9 9 notch on aortic pressure curve (dicrotic notch) Left atrial pressure Left atrial 1 pressure 4 1 4 0 0 22 22 (mm Hg) (mm Hg) 5 5 21 21 19: All valves closed again 135 End- diastolic 7 11 135 End- diastolic 7 Ventricular 11 phase filling Ventricula phase volume volume Left ventricular Left ventricular 2 142 20: Chamber volume remains constant 6 6 14 volume volume (mL) (mL) 24 Reduced 24 R filling f 23 23 End- 20 Rapid End- 20 Rapid 65 systolic 65 filling systolic filling volume 15 volume 15 No sound 1st 2nd No sound 1st 2nd 1st Heart sounds Heart sounds Ventricular Ventricular Ventricular Ventricu systole systole diastole diastol 8 8 QRS QRS VENTRICULAR FILLING 16 16 16 16 3 3 25 P T P T Electrocardiogram Electrocardiogram 21: Ventricular pressure < atrial pressure (AV Ejection Ejection 120 phase 120 phase valves open) 13 13 13 13 18 18 100 100 17 17 22: Atria pressure increasing due to constant Aortic pressure Aortic pressure 12 12 (mm Hg) (mm Hg) 80 80 ventricular relaxation Isovolumetric ventricular relaxation Isovolumetric flow of blood from veins ventricular contraction ventricular contraction 60 60 23: Pool of blood in atria rush into ventricles 19 19 19 19 Isovolumetric Isovolumetric Left ventricular Left ventricular 10 10 pressure 40 pressure 40 when AV valves open (rapid filling) (mm Hg) (mm Hg) 20 20 9 9 24: Filling of blood slow down Left atrial Left atrial 1 4 1 4 pressure pressure 0 0 22 22 5 (mm Hg) (mm Hg) 21 5 21 25: SA node fire and impulse spread through 135 End- diastolic 7 11 135 End- Ventricular 7 11 filling diastolicphase Ventricula phase atria again (P wave). Cardiac cycle repeats. volume volume Left ventricular Left ventricular 2 6 14 2 6 14 volume volume (mL) (mL) 24 Reduced 24 R filling 23 23 End- 20 RapidEnd- 20 Rapid 65 systolic 65 filling systolic filling volume 15 volume 15 No sound 1st No2nd sound 1st 1st 2nd 2nd Heart sounds Heart sounds CARDIAC CYCLE Key points: When valves open and close Correlation with heart sounds Correlation to ECG Closure of AV valves signal start of ventricular systole Closure of aortic valves signal end of ventricular systole and start of diastole HEART SOUNDS S1 – 1st heart sound (Lub) S2 – 2nd heart sound (Dub) S3 – 3rd heart sound Passive filling sound of the ventricles, cannot be heard normally except in hyperdynamic circulation or pathologically in cardiac failure S4 – 4th heart sound At atrial systole, ventricular hypertrophy, difficulty in filling up ventricles during atrial contraction Cardiac murmurs Produced by turbulent flow of blood across incompetent (regurgitating) or stenotic (narrowed) valves CARDIAC OUTPUT (CO) Volume of blood pumped out by each ventricle per min Depends on heart rate and stroke volume Heart rate (HR) – beats per min Stroke volume (SV) – volume of blood pumped per beat or stroke CO = HR X SV = 70 beats/min X 70 ml/beat = 4900 ml/min ≈ 5L/min EFFECT OF ANS ON HEART RATE Heart rate Sympathetic Parasympathetic activity activity (and epinephrine) Fig 9-19, Sherwood STROKE VOLUME Stroke volume is determined by the extent of venous return and by sympathetic activity Intrinsic control: related to the extent of venous return Extrinsic control: related to the extent of sympathetic stimulation of the heart Fig 9-20, Sherwood CONTROL OF STROKE VOLUME Intrinsic control of stroke volume Increased end-diastolic volume (EDV) results in increased stroke volume Frank-Starling Law of the Heart Intrinsic relationship between EDV and SV “the heart normally pumps out during systole, the volume of blood return to it during diastole” CONTROL OF STROKE VOLUME Frank-Starling Law of the Heart ↑ diastolic filling - ↑ EDV - ↑ stretching of the heart - ↑ length of cardiac muscle fibers before contraction - ↑ force on subsequent cardiac contraction - ↑ SV Fig 9-21, Sherwood CONTROL OF STROKE VOLUME Effect of sympathetic stimulation on stroke volume (Extrinsic control) Fig 9-22, Sherwood CONTROL OF STROKE VOLUME Sympathetic stimulation increases the contractility of the heart A change in contractility is defined as change in the work performed by the heart not brought about by change in initial fiber length Fig 9-23, Sherwood STROKE VOLUME SV = EDV – ESV Ejection fraction (EF) Fraction of blood ejected from a ventricle with each heartbeat Measure of cardiac contractility EF= SV/EDV ×100% Normal = 55 – 75% Measured by echocardiography Definitive measurement by cardiac catherisation STROKE VOLUME AND CARDIAC OUTPUT Strength of cardiac muscle contraction Varying the initial length of muscle fiber which depends on ventricular filling (intrinsic control) Varying the extend of sympathetic stimulation (extrinsic control) Sympathetic stimulation increases cardiac output by increasing both HR and SV Fig 9-24, Sherwood CARDIAC OUTPUT (CO) Volume of blood pumped out by each ventricle per min Factors affecting cardiac output Preload (volume of filling), heart (contractility, heartrate) and afterload (peripheral resistance) Cardiac failure occurs when cardiac output is insufficient to service the metabolic requirements of the body’s tissues CARDIAC FAILURE (HEART FAILURE) Cardiac failure occurs when cardiac output is insufficient to service the metabolic requirements of the body’s tissues Compensatory measures for systolic heart failure Sympathetic stimulation Retention of salt and water by kidneys to expand blood volume Major effect of cardiac failure are caused by congestion in pulmonary and/or systemic circulations BLOOD SUPPLY TO THE HEART The heart receives most of its blood supply through the coronary circulation during diastole Matching of coronary blood flow to heart muscle’s O2 needs Coronary blood flow is adjusted in response to changes in the heart’s O2 requirements Fig 9-26, Sherwood BLOOD SUPPLY TO THE HEART Fig 3.65, Drake Fig 9-29 Sherwood CORONARY ARTERY DISEASE (CAD) Angina pectoris Sensation of chest pain (epigastric region, neck, shoulder etc) as a result of myocardial ischemia Common presenting symptom of CAD Acute Myocardial Infarction (MI) “heart attack” Irreversible death (necrosis) of part of heart muscle secondary to ischemia CORONARY ARTERY DISEASE (CAD) Causes? Atherosclerosis - progressive, degenerative arterial disease Leads to occlusion of affected vessels, reducing blood flow through them Fig 9-27 Sherwood Fig 9-28 Sherwood Causes? CORONARY Thrombus – abnormal clot in the vessel wall ARTERY DISEASE Embolus – abnormal particle floating in the blood vessels (CAD) Thromboembolism – obstruction of blood vessel by a blood clot dislodged from another site Examples of some surgical treatment / intervention CORONARY ARTERY Percutaneous transluminal coronary angioplasty (PTCA) DISEASE (CAD) Coronary artery bypass grafting (CABG) CORONARY ARTERY DISEASE (CAD) REFERENCES Chapter 9 Sherwood, L. (2016) Human Physiology: From Cells to Systems. 9th edition. Cengage Chapter 20 Martini, F. H., Nath, J.L., & Bartholomew, E. F. (2018). Fundamentals of Anatomy and Physiology. 11th Global Edition. Pearson. HSC1007 ANATOMY AND PHYSIOLOGY 1 CARDIOVASCULAR PHYSIOLOGY CIRCULATORY SYSTEM AND BLOOD PRESSURE ANDY LEE (PHD) ASSISTANT PROFESSOR FACULTY HALL #04-17 OFFICE PHONE: 6592 2524 [email protected] LEARNING OUTCOMES At the end of the lesson, you should be able to: Describe the main features of the different blood vessels Explain how arteries function as a pressure reservoir Explain how the sounds of Korotkoff are used to measure blood pressure Distinguish between pulse pressure, arterial blood pressure and mean arterial blood pressure Describe the mechanisms by which blood flow to different organs/tissues can be regulated Describe the factors regulating venous return Describe the factors that affect mean arterial blood pressure Describe both short and long term control of blood pressure INSIDE THE HUMAN BODY: BLOOD CIRCULATION ORGANIZATION OF CVS Fig 10-4, Sherwood FEATURES OF BLOOD VESSELS Table 10-1, Sherwood Endothelium Elastin fibers Smooth muscle Collagen fibers RECONDITIONING BLOOD Blood is transported to all parts of the body through a system of vessels Brings fresh supplies to the vicinity of all cells while removing their wastes Reconditioning organs receive more blood than what they need to perform homeostatic adjustments to blood Example: Digestive tract (20% of CO; to pick up nutrient supplies) Kidneys (20% of CO, to eliminate waste, adjust water and electrolytes) PRESSURE VS FLOW RATE Flow rate Volume of blood passing through per unit time Proportional to pressure gradient and inversely proportional to resistance Blood pressure Force exerted by the blood against a vessel Pressure gradient Difference in pressure between the beginning and end of a vessel Resistance Fig 10-2a, Sherwood Friction between the blood and vascular wall ARTERIES Arteries serve as rapid- transit passageways to the organs and as a pressure reservoir Heart contracts to pump blood into arteries and relaxes to refill with blood from veins BLOOD PRESSURE Force exerted by blood against a vessel wall Depends on volume of blood within the vessel; and Distensibility of the vessel walls Systolic pressure Maximum pressure when blood is ejected into the arteries Diastolic pressure Minimum pressure when blood is draining into the rest of the vessel during diastole Indicated as systolic/diastolic (120/80 mmHg) BLOOD PRESSURE Fig 10-7, Sherwood Korotkoff sounds BLOOD PRESSURE https://www.youtube.com/watch?v=bHXvhOQ0hYc PULSE PRESSURE Pulse pressure = systolic – diastolic Pulse wave can be FELT over major arteries Wave results from difference in systolic and diastolic pressure Fig 10-7, Sherwood Strong pulse wave is just strong difference between systolic and diastolic pressure Pulsating in nature (pulsatile) Elastic properties of arteries help convert pulsatile flow of blood from heart into more continuous flow in the capillaries MEAN ARTERIAL PRESSURE (MAP) Main driving force for blood flow Average pressure driving blood forward Is the pressure that is monitored and regulated by body’s blood pressure reflexes (homeostasis) MAP = Diastolic pressure + 1/3 pulse pressure; or MAP = 2/3 diastolic + 1/3 systolic Example: if car drives 80km/h for 40min and 120km/h for 20min. Average speed is 93km/h and not 100km/h. BLOOD PRESSURE In arteries, little resistance, little pressure lost, so pressure is around the same throughout arterial tree. Pressure drops from arteries to veins – increasingly non- pulsatile Fig 10-8, Sherwood CAPILLARIES Ideally suited to serve as site of exchange Very thin walled, extensive branching and proximity of almost every cell to a capillary RBC in a single file Blood velocity in capillaries is very slow as compared to arteries Total flow rate (~5L/min) is the same throughout circulatory tree (due to increased surface area) CAPILLARIES Fig 10-17, Sherwood CAPILLARIES Fig 10-15, Sherwood PRE-CAPILLARY SPHINCTERS Tissues that are metabolic active have more capillaries Many capillaries are not open under resting conditions Capillaries have no smooth muscles Thus, pre-capillary sphincters serve to control blood flow Sensitive to local metabolic activity changes ↑ metabolic activity → sphincter relax → more open capillaries → increased blood flow to active tissues Fig 10-18, Sherwood REGULATION OF BLOOD FLOW AND DISTRIBUTION Local control of arteriolar (arterioles) Local vasoregulation of radius is important in determining arterioles the distribution of cardiac output Vasoconstriction The fraction of the total CO delivered - to each organ varies depending on Vasodilation demands for blood - Differences in flow to organs are Vascular tone determined by differences in state of partial constriction of vascularization and differences in arteriolar smooth muscle resistance offered by arterioles Establishes a baseline of arteriolar supplying each organ resistance VASOREGULATION OF ARTERIOLES Fig 10-9, Sherwood EXTRINSIC CONTROL OF ARTERIOLAR RADIUS Extrinsic control of arteriolar radius is important in regulating blood pressure Influence of total peripheral resistance (TPR) on mean arterial pressure Sympathetic fibers supply arteriolar smooth muscle everywhere (except in brain) Increased sympathetic activity – generalized vasoconstriction Decreased sympathetic activity – generalized vasodilation EXTRINSIC CONTROL OF ARTERIOLAR RADIUS Local controls overriding sympathetic vasoconstriction Riding bicycle (exercise) → increased sympathetic activity → generalized vasoconstriction → local metabolic activity in leg muscle induces vasodilation (override) → more blood to leg muscle → lesser blood to arm muscles and viscera etc No parasympathetic innervation to arterioles (except in the penis and clitoris) EXTRINSIC CONTROL OF ARTERIOLAR RADIUS Cardiovascular control center Control the sympathetic output Adrenal hormones Norepinephrine produces generalized vasoconstriction (Epinephrine too, but weaker) Epinephrine reinforces local vasodilatory mechanisms in tissues (mostly in skeletal muscles and heart) Vasopression and angiotensin II (potent vasoconstrictors) Vasopressin maintains water balance Angiotensin II regulates salt balance DURING EXERCISE Cardiac output ↑ Vasodilation in skeletal muscle and heart More blood diverted to these organs Body heat ↑ Vasodilation of the skin also (flushing of skin) DURING EXERCISE Sherwood, Chapter 10, Closer look at Exercise Physiology BLOOD PRESSURE Affected by cardiac output and total peripheral resistance Blood Pressure Needs to be closely regulated High enough (adequate pressure) to maintain blood flow and tissue Cardiac TPR perfusion (fluid exchange) Output Not too high to overwork heart and cause vascular damage and Arteriolar HR radius rupture of small vessels Blood SV viscosity VENOUS RETURN Blood returning to the heart Veins Low resistance, less elastic recoil, less smooth muscle Slow transit time through it (act as a blood reservoir) Storage as moving not as quickly to the heart to be pumped out Venous capacity (amount of blood veins can hold) Depends on distensibility of vessel walls and other external pressures such as skeletal muscles squeezing it During exercise Stored blood needed – increase in venous return REGULATION OF VENOUS RETURN Sympathetic innervation Veins have low tone, but high sympathetic innervation: vasoconstriction, ↑ venous pressure Note: Vasoconstriction in veins: ↑ flow (from ↓ capacity, squeezing out more blood already present); while arteriole vasoconstriction ↓ flow (from ↑ resistance) Skeletal muscle activity Many large veins lie between skeletal muscles in arms/legs When muscle contracts, veins compressed (skeletal muscle pump) ↓ venous capacity, ↑ venous pressure (extra way that blood returns to heart during exercise) REGULATION OF VENOUS RETURN Gravity Lying down, equal Standing up- vessels below heart subject to gravity (pressure caused by weight of blood from heart to vessel) Distensible veins yield under hydrostatic pressure- ↑ capacity In leg, post-capillary blood pools in extended veins, ↓ VR, ↓CO Fig 10-26, Sherwood EFFECT OF GRAVITY Lie down- stand up compensations: Triggers sympathetic venous vasoconstriction, driving some of the pooled blood forward Skeletal muscle pump “interrupts” column of blood Sympathetic venous vasoconstriction cannot completely compensate without skeletal pump Scenario 1. Person stands still for long time, blood flow to brain reduced (↓ in circulating volume, despite reflexes for maintaining MAP), leads to fainting- horizontal positioning 2. Postural hypotension EFFECT OF GRAVITY Valves stop blood from going backwards: One-way valves spaced 2-4 cm away Also help counteract gravity (minimize back flow) Varicose veins Incompetent venous valves (can no longer support the column of blood above it and collapse) Aggravated by frequent, prolonged standing OTHER FACTORS Respiratory activity Pressure within chest cavity is 5 mmHg less than atmospheric pressure External pressure gradient between the lower veins (atmospheric pressure) and chest veins (less than atmospheric pressure) Cardiac suction Ventricular contraction, AV valves closed but drawn downward, enlarging atrial cavity so atrial pressure transiently drops lower than 0mmHg – sucking more blood into atria Ventricular relaxation, AV valves open, rapid expansion of ventricles creates a suction effect sucking blood from atrium and veins FACTORS FACILITATING VENOUS RETURN Fig 10-25, Sherwood SUMMARY? Fig 10-29, Sherwood SUMMARY OF NERVOUS INPUT ON BP Fig 10-32, Sherwood SHORT TERM REGULATION OF BP (BARORECEPTOR REFLEX) Any change in MAP will trigger an automatic baroreceptor reflex in attempt to restore BP to norm BP ↓ - detected by baroreceptor – Cardiovascular center - ↑ sympathetic and ↓ parasympathetic – ↑ HR, ↑ SV, arteriole vasoconstriction (↑ total peripheral resistance) and venous vasoconstriction (↑ CO) - ↑ BP Fig 10-30, Sherwood LONG TERM REGULATION OF BLOOD PRESSURE Regulating blood volume ↓ blood volume results in ↓ arterial BP Major compensation is reabsorption of fluid by kidney (salt and water balance) Renin-Angiotensin system of the kidney HYPERTENSION Hypertension is a national public-health problem, but its causes are largely unknown Types of hypertension: primary and secondary (secondary to other known problem/disease) Baroreceptor adaptation during hypertension: adapt to operate at a higher level Causes? (Too much dietary salt? Disturbance of renin-angiotensin system leading to increase blood volume?) Complications of hypertension: left ventricular hypertrophy, stroke, heart attack, kidney failure, and progressive vision loss HYPERTENSION MOH Clinical Practice Guidelines on Hypertension, 2017 American College of Cardiology, American Heart Association, … Guidelines, 2017 REFERENCES Chapter 10 Sherwood, L. (2016) Human Physiology: From Cells to Systems. 9th edition. Cengage