Cardiovascular Physiology PDF
Document Details
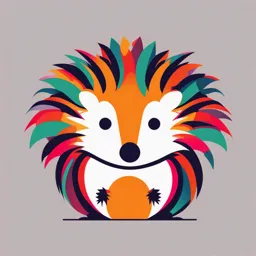
Uploaded by SelfSatisfactionPrairieDog8417
Superior University
Tags
Related
Summary
This textbook chapter details the anatomy of the heart, its electrical and mechanical functions, and the circulatory system. It explains how the heart acts as a dual pump, nourishing heart muscle, and the important role of coronary circulation. Key concepts like cardiac output control and coronary artery disease are also covered.
Full Transcript
CH AP TE R Cardiac Physiology CONTENTS AT A GLANCE Anatomy of the Heart Location of the heart Anatomy of the Heart The heart as a dual pump From just a matter of days following...
CH AP TE R Cardiac Physiology CONTENTS AT A GLANCE Anatomy of the Heart Location of the heart Anatomy of the Heart The heart as a dual pump From just a matter of days following conception until death, the Heart valves beat goes on. In fact, throughout an average human life span, Heart walls; cardiac muscle the heart contracts about 3 billion times, never stopping except Pericardial sac for a fraction of a second to ll between beats. Within about Electrical Activity of the Heart three weeks a er conception, the heart of the developing em- bryo starts to function. It is the rst organ to become func- Pacemaker activity tional. At this time the human embryo is only a few millimeters Spread of cardiac excitation long, about the size of a capital letter on this page. Action potential of cardiac contractile cells Why does the heart develop so early, and why is it so crucial Cardiac refractory period throughout life? It is this important because the circulatory Electrocardiography system is the body’s transport system. A human embryo, having Mechanical Events of the Cardiac Cycle very little yolk available as food, depends on promptly establish- Electrical, pressure, and volume relationships during ing a circulatory system that can interact with the mother’s diastole and systole circulation to pick up and distribute to the developing tissues Heart sounds the supplies so critical for survival and growth. us begins the Cardiac Output and Its Control story of the circulatory system, which continues throughout life Determinants of cardiac output to be a vital pipeline for transporting materials on which the cells of the body absolutely depend. Control of heart rate e circulatory system has three basic components: Control of stroke volume Nourishing the Heart Muscle 1. e heart serves as the pump that imparts pressure to the Coronary circulation blood to establish the pressure gradient needed for blood to ow to the tissues. Like all liquids, blood ows down a pressure Coronary artery disease gradient from an area of higher pressure to an area of lower pressure. is chapter focuses on cardiac physiology (cardia means “heart”). 2. e blood vessels serve as the passageways through which blood is directed and distributed from the heart to all parts of the body and subsequently returned to the heart. e smallest of the blood vessels are designed for rapid exchange of materials between the surrounding tissues and the blood within the ves- sels (see Chapter 10). 3. Blood is the transport medium within which materials be- ing transported long distances in the body (such as O2, CO2, nutrients, wastes, electrolytes, and hormones) are dissolved or suspended (see Chapter 11). Blood travels continuously through the circulatory system Log on to CengageNOW at http://www to and from the heart through two separate vascular (blood.cengage.com/sso/ for an opportunity to explore a learning vessel) loops, both originating and terminating at the heart module that illustrates diffi cult concepts with self-study ( Figure 9-1). e pulmonary circulation consists of a closed tutorials, animations, and interactive quizzes to help you learn, loop of vessels carrying blood between the heart and lungs review, and master physiology concepts. 303 Systemic lung and the le lung; the systemic circulation circulation simultaneously loops through the upper half and the lower half of the body. The heart is positioned in the middle of the thoracic cavity. Capillary networks of e heart is a hollow, muscular organ about the upper body size of a clenched st. It lies in the thoracic (chest) cavity about midline between the ster- num (breastbone) anteriorly and the vertebrae Systemic arteries (backbone) posteriorly. Place your hand over your (to upper body) heart. People usually put their hand on the le side of the chest, even though the heart is actually Pulmonary Pulmonary circulation circulation in the middle of the chest. e heart has a broad Systemic base at the top and tapers to a pointed tip, the veins Aorta apex, at the bottom. It is situated at an angle un- Pulmonary Pulmonary artery artery der the sternum so that its base lies predomi- nantly to the right and the apex to the le of the sternum. When the heart beats forcefully, the apex actually thumps against the inside of the Pulmonary chest wall on the le side. Because we become Pulmonary vein vein aware of the beating heart through the apex beat on the le side of the chest, we tend to think— erroneously—that the entire heart is on the le. Capillary network Capillary network e heart’s position between two bony of right lung Systemic Systemic of left lung structures, the sternum and vertebrae, veins arteries makes it possible to manually drive blood (to lower body) out of the heart when it is not pumping e ectively, by rhythmically depressing the sternum. is ma- neuver compresses the heart between the sternum Capillary and vertebrae so that blood is squeezed out into the networks of blood vessels, maintaining blood ow to the tis- lower body sues. O en this external cardiac compression, which is part of cardiopulmonary resuscitation (CPR), serves as a lifesaving measure until appropriate therapy can restore the heart to normal function. The heart is a dual pump. Systemic Even though anatomically the heart is a single circulation organ, the right and le sides of the heart func- KEY tion as two separate pumps. e heart is divided into right and le halves and has four chambers, = O2-rich blood = O2-poor blood an upper and a lower chamber within each half ( Figure 9-2a). e upper chambers, the atria FIGURE 9-1 Pulmonary and systemic circulation in relation to the heart. The circulatory system consists of two separate vascular loops: the pulmo- (singular, atrium), receive blood returning to the nary circulation, which carries blood between the heart and lungs; and the sys- heart and transfer it to the lower chambers, the temic circulation, which carries blood between the heart and organ systems. Each ventricles, which pump blood from the heart. of these loops forms a figure “8,” with the pulmonary circulation simultaneously e vessels that return blood from the tissues to supplying the right and left lungs, and the systemic circulation simultaneously sup- the atria are veins, and those that carry blood plying the upper body and lower body. away from the ventricles to the tissues are arter- ies. e two halves of the heart are separated by the septum, a continuous muscular partition that (pulmo means “lung”). e systemic circulation is a circuit of prevents mixture of blood from the two sides of the heart. is vessels carrying blood between the heart and other body sys- separation is extremely important, because the right side of the tems. Each of these vascular loops forms a gure “8.” e pul- heart is receiving and pumping O2-poor blood, whereas the le monary circulation simultaneously loops through the right side of the heart receives and pumps O2-rich blood. 304 Chapter 9 To systemic circulation (upper body) Aorta Superior vena cava (returns blood from Right and left head, upper limbs) pulmonary arteries (to lungs) Right Left Right pulmonary ventricular ventricular veins (return blood Left pulmonary veins (return blood wall wall from right lung) from left lung) Pulmonary semilunar valve (shown open) Left atrium Aortic semilunar Right atrium valve (shown open) Right atrioventricular Left atrioventricular valve (shown open) valve (shown open) Right ventricle Left ventricle Inferior vena cava Septum (returns blood from trunk, legs) KEY O2-rich blood To systemic circulation O2-poor blood (lower body) (a) Blood flow through the heart (c) Thickness of right and left ventricles Right atrium Right ventricle Venae cavae Pulmonary artery Other Digestive Systemic Pulmonary systemic Brain Kidneys Muscles Lungs tract circulation circulation organs Aorta Pulmonary veins Left ventricle Left atrium (b) Dual pump action of the heart FIGURE 9-2 Blood flow through and pump action of the heart. (a) The arrows indicate the direction of blood flow. To illustrate the direction of blood flow through the heart, all of the heart valves are shown open, which is never the case. The right side of the heart receives O2-poor blood from the systemic circulation and pumps it into the pulmonary circulation. The left side of the heart receives O2-rich blood from the pulmonary circulation and pumps it into the systemic circulation. (b) Note the parallel pathways of blood flow through the systemic organs. (The rela- tive volume of blood flowing through each organ is not drawn to scale.) (c) Note that the left ventricular wall is much thicker than the right wall. Cardiac Physiology 305 THE COMPLETE CIRCUIT OF BLOOD FLOW Let us look at how the heart functions as a dual pump by tracing a drop of blood When pressure is greater through one complete circuit ( Figure 9-2a and b). Blood re- behind the valve, it opens. turning from the systemic circulation enters the right atrium via two large veins, the venae cavae, one returning blood from above and the other returning blood from below heart level. Valve opened e drop of blood entering the right atrium has returned from When pressure is greater in the body tissues, where O2 has been taken from it and CO2 has front of the valve, it closes. been added to it. is partially deoxygenated blood ows from Note that when pressure is the right atrium into the right ventricle, which pumps it out greater in front of the valve, it does not open in the opposite through the pulmonary artery, which immediately forms two direction; that is, it is a branches, one going to each of the two lungs. us, the right side one-way valve. of the heart receives blood from the systemic circulation and Valve closed; does not open in opposite direction pumps it into the pulmonary circulation. Within the lungs, the drop of blood loses its extra CO2 and FIGURE 9-3 Mechanism of valve action. picks up a fresh supply of O2 before being returned to the le atrium via the pulmonary veins coming from both lungs. is O2-rich blood returning to the le atrium subsequently ows muscle on the right side, making the le side a stronger pump into the le ventricle, the pumping chamber that propels the ( Figure 9-2c). blood to all body systems except the lungs; that is, the le side of the heart receives blood from the pulmonary circulation and Pressure-operated heart valves pumps it into the systemic circulation. e single large artery carrying blood away from the le ventricle is the aorta. Major ensure that blood flows in the right arteries branch from the aorta to supply the various organs of direction through the heart. the body. Blood ows through the heart in one xed direction from veins In contrast to the pulmonary circulation, in which all the to atria to ventricles to arteries. e presence of four one-way blood ows through the lungs, the systemic circulation may be heart valves ensures this unidirectional ow of blood. e valves viewed as a series of parallel pathways. Part of the blood are positioned so that they open and close passively because of pumped out by the le ventricle goes to the muscles, part to the pressure di erences, similar to a one-way door ( Figure 9-3). A kidneys, part to the brain, and so on ( Figure 9-2b). us, the forward pressure gradient (that is, a greater pressure behind the output of the le ventricle is distributed so that each part of the valve) forces the valve open, much as you open a door by push- body receives a fresh blood supply; the same arterial blood does ing on one side of it, whereas a backward pressure gradient (that not pass from organ to organ. Accordingly, the drop of blood is, a greater pressure in front of the valve) forces the valve closed, we are tracing goes to only one of the systemic organs. Tissue just as you apply pressure to the opposite side of the door to cells within the organ take O2 from the blood and use it to oxi- close it. Note that a backward gradient can force the valve closed dize nutrients for energy production; in the process, the tissue but cannot force it to swing open in the opposite direction; that cells form CO2 as a waste product that is added to the blood (see is, heart valves are not like swinging, saloon-type doors. pp. 2 and 37). e drop of blood, now partially depleted of O2 content and increased in CO2 content, returns to the right side AV VALVES BETWEEN THE ATRIA AND VENTRICLES Two of the of the heart, which once again will pump it to the lungs. One heart valves, the right and le atrioventricular (AV) valves, circuit is complete. are positioned between the atrium and the ventricle on the right and le sides, respectively ( Figure 9-4a). ese valves let COMPARISON OF THE RIGHT AND LEFT PUMPS Both sides of blood ow from the atria into the ventricles during ventricular the heart simultaneously pump equal amounts of blood. e lling (when atrial pressure exceeds ventricular pressure) but volume of O2-poor blood being pumped to the lungs by the prevent the back ow of blood from the ventricles into the atria right side of the heart soon becomes the same volume of O2- during ventricular emptying (when ventricular pressure greatly rich blood being delivered to the tissues by the le side of the exceeds atrial pressure). If the rising ventricular pressure did heart. e pulmonary circulation is a low-pressure, low- not force the AV valves to close as the ventricles contracted to resistance system, whereas the systemic circulation is a high- empty, much of the blood would ine ciently be forced back pressure, high-resistance system. Pressure is the force exerted into the atria and veins instead of being pumped into the arter- on the vessel walls by the blood pumped into the vessels by the ies. e right AV valve is also called the tricuspid valve (tri heart. Resistance is the opposition to blood ow, largely means “three”) because it consists of three cusps or lea ets caused by friction between the owing blood and the vessel ( Figure 9-4b). Likewise, the le AV valve, which has two wall. Even though the right and le sides of the heart pump cusps, is o en called the bicuspid valve (bi means “two”) or, the same amount of blood, the le side performs more work, alternatively, the mitral valve (because of its physical resem- because it pumps an equal volume of blood at a higher pres- blance to a mitre, or bishop’s traditional hat). sure into a higher-resistance and longer system. Accordingly, e edges of the AV valve lea ets are fastened by tough, the heart muscle on the le side is much thicker than the thin, brous cords of tendinous-type tissue, the chordae ten- 306 Chapter 9 dineae, which prevent the valves from being everted. at is, the Aorta chordae tendineae prevent the AV valve from being forced by the high ventricular pressure to open in the Pulmonary artery Superior vena cava opposite direction into the atria. ese cords extend from the edges Pulmonary valve of each cusp and attach to small, Pulmonary veins nipple-shaped papillary muscles, which protrude from the inner sur- Left atrium face of the ventricular walls (papilla Pulmonary veins means “nipple”). When the ventri- Left AV valve cles contract, the papillary muscles Right atrium Aortic valve also contract, pulling downward on the chordae tendineae. is pulling Right AV valve Chordae tendineae exerts tension on the closed AV Papillary muscle valve cusps to hold them in posi- tion, much like tethering ropes hold Left ventricle down a hot-air balloon. is action helps keep the valve tightly sealed in Right ventricle Interventricular septum the face of a strong backward pres- Inferior vena cava sure gradient ( Figure 9-4c). (a) Location of the heart valves in a longitudinal section of the heart SEMILUNAR VALVES BETWEEN THE VENTRICLES AND MAJOR ARTERIES e two remaining heart valves, the aortic and pulmonary valves, lie at the juncture where the major arter- ies leave the ventricles ( Figure 9-4a). ey are known as semilunar valves because they have three Right AV valve Left AV valve Aortic or pulmonary valve cusps, each resembling a shallow (b) Heart valves in closed position, viewed from above half-moon-shaped pocket (semi means “half ”; lunar means “moon”) ( Figure 9-4b). ese valves are Right atrium Direction of backflow of blood forced open when the le and right Right AV valve Chordae tendineae Aorta ventricular pressures exceed the Direction of Aortic Leakproo pressure in the aorta and pulmo- backflow of valve blood “seam” Septum nary artery, respectively, during ventricular contraction and empty- Right ventricle ing. Closure results when the ven- tricles relax and ventricular pres- Papillary muscle sures fall below the aortic and pulmonary artery pressures. e (c) Prevention of eversion of AV valves (d) Prevention of eversion of semilunar valve closed valves prevent blood from FIGURE 9-4 Heart valves. Eversion of the AV valves is prevented by tension on the valve leaf- owing from the arteries back into lets exerted by the chordae tendineae when the papillary muscles contract. When the semilunar the ventricles from which it has just valves are swept closed, their upturned edges fit together in a deep, leakproof seam that prevents been pumped. valve eversion. e semilunar valves are pre- vented from everting by the ana- tomic structure and positioning of the cusps. When on ven- the atria into the veins usually is not a signi cant problem, for tricular relaxation a backward pressure gradient is created, the two reasons: (1) atrial pressures usually are not much higher than back surge of blood lls the pocketlike cusps and sweeps them venous pressures, and (2) the sites where the venae cavae enter into a closed position, with their unattached upturned edges the atria are partially compressed during atrial contraction. tting together in a deep, leakproof seam ( Figure 9-4d). FIBROUS SKELETON OF THE VALVES Four interconnecting NO VALVES BETWEEN THE ATRIA AND VEINS Even though there rings of dense connective tissue provide a rm base for attach- are no valves between the atria and veins, back ow of blood from ment of the four heart valves ( Figure 9-5). is brous Cardiac Physiology 307 (Rear) heart’s complex twisting during development. As a result of this Fibrous arrangement, when the ventricular muscle contracts and short- rings ens, the diameter of the ventricular chambers is reduced while Right AV valve Left AV valve the apex is simultaneously pulled upward toward the top of the heart in a rotating manner. is exerts a “wringing” e ect, e - ciently exerting pressure on the blood within the enclosed chambers and directing it upward toward the openings of the major arteries that exit at the base of the ventricles. To support their rhythmic, lifelong contractile activity (re- member heart muscle cells cannot divide, so at least most of them live as long as you do), they have an abundance of energy- Aortic valve generating mitochondria, and they receive a rich blood supply, about one capillary for each myocardial ber. Ventricular Pulmonary semilunar myocardium valve Cardiac muscle fibers are interconnected by (Front) intercalated discs and form functional syncytia. FIGURE 9-5 Fibrous skeleton of the heart. A view of the e individual cardiac muscle cells are interconnected to form heart from above, with the atria and major vessels removed to show the heart valves and fibrous rings. Note that the inlet and branching bers, with adjacent cells joined end to end at spe- outlet valves to the ventricle all lie on the same plane through the cialized structures known as intercalated discs. Two types of heart. membrane junctions are present within an intercalated disc: desmosomes and gap junctions ( Figure 9-6b and c). A des- mosome, a type of adhering junction that mechanically holds skeleton, which separates the atria from the ventricles, also cells together, is particularly abundant in tissues such as the provides a fairly rigid structure for attachment of the cardiac heart that are subject to considerable mechanical stress (see muscle. e atrial muscle mass is anchored above the rings, p. 58). At intervals along the intercalated disc, the opposing and the ventricular muscle mass is attached to the bottom of membranes approach each other very closely to form gap junc- the rings. tions, which are areas of low electrical resistance that allow It might seem rather surprising that the inlet valves to the action potentials to spread from one cardiac cell to adjacent ventricles (the AV valves) and the outlet valves from the ven- cells (see p. 59). Some cardiac muscle cells can generate action tricles (the semilunar valves) all lie on the same plane through potentials without any nervous stimulation. When one of the the heart, as delineated by the brous skeleton. is relation- cardiac cells spontaneously undergoes an action potential, the ship comes about because the heart forms from a single tube electrical impulse spreads to all the other cells that are joined that bends on itself and twists on its axis during embryonic by gap junctions in the surrounding muscle mass, so that they development. Although this turning and twisting make study- become excited and contract as a single, functional syncytium ing the structural relationships of the heart more di cult, the (see p. 295). e atria and the ventricles each form a functional twisted structure has functional importance in that it helps the syncytium and contract as separate units. e synchronous heart pump more e ciently. We will see how by turning our contraction of the muscle cells that make up the walls of each attention to the portion of the heart that actually generates the of these chambers produces the force needed to eject the en- forces responsible for blood ow, the cardiac muscle within the closed blood. heart walls. No gap junctions join the atrial and ventricular contractile cells, and furthermore, the atria and ventricles are separated by the electrically nonconductive brous skeleton that surrounds The heart walls are composed primarily and supports the valves. However, an important, specialized of spirally arranged cardiac muscle fibers. conduction system facilitates and coordinates transmission of e heart wall has three distinct layers: electrical excitation from the atria to the ventricles to ensure synchronization between atrial and ventricular pumping. A thin inner layer, the endothelium, a unique type of epi- Because of both the syncytial nature of cardiac muscle and thelial tissue that lines the entire circulatory system the conduction system between the atria and ventricles, an im- A middle layer, the myocardium, which is composed of pulse spontaneously generated in one part of the heart spreads cardiac muscle and constitutes the bulk of the heart wall (myo throughout the entire heart. erefore, unlike skeletal muscle, means “muscle”; cardia means “heart”) where graded contractions can be produced by varying the A thin external layer, the epicardium, that covers the heart number of muscle cells contracting within the muscle (recruit- (epi means “on”) ment of motor units), either all the cardiac muscle bers con- tract or none do. A “hal earted” contraction is not possible. e myocardium consists of interlacing bundles of cardiac Cardiac contraction is graded by varying the strength of con- muscle bers arranged spirally around the circumference of the traction of all the cardiac muscle cells. You will learn more heart ( Figure 9-6a). e spiral arrangement is the result of the about this process in a later section. 308 Chapter 9 The heart is enclosed by the pericardial sac. e heart is enclosed in the double-walled, membranous peri- cardial sac (peri means “around”). e sac consists of two layers—a tough, brous covering and a secretory lining. e outer brous covering of the sac attaches to the connective tissue partition that separates the lungs. is attachment anchors the heart so that it remains properly positioned within the chest. e sac’s secretory lining secretes a thin pericardial uid, which provides lubrication to prevent friction between the pericardial layers as they glide over each other with every beat of the heart. Pericarditis, an in ammation of the pericardial sac that (a) Bundles of cardiac muscle results in a painful friction rub between the two peri- are arranged spirally around the cardial layers, occurs occasionally because of viral or ventricle. When they contract, they bacterial infection. “wring” blood from the apex to the base where the major arteries exit. Building on this foundation of heart structure, we now ex- plain how action potentials are initiated and spread throughout the heart, followed by a discussion of how this electrical activity Intercalated discs © Dr. John Cunningham/Visuals Unlimited brings about coordinated pumping of the heart. Electrical Activity of the Heart Contraction of cardiac muscle cells to eject blood is triggered by action potentials sweeping across the muscle cell membranes. e heart contracts, or beats, rhythmically as a result of action potentials that it generates by itself, a property called auto- rhythmicity (auto means “self ”). ere are two specialized types of cardiac muscle cells: (b) Cardiac muscle fibers branch and are interconnected by 1. Contractile cells, which are 99% of the cardiac muscle cells, intercalated discs. do the mechanical work of pumping. ese working cells nor- mally do not initiate their own action potentials. 2. In contrast, the small but extremely important remainder of Plasma membranes of Desmosome adjacent cardiac muscle fibers the cardiac cells, the autorhythmic cells, do not contract but instead are specialized for initiating and conducting the action potentials responsible for contraction of the working cells. Cardiac autorhythmic cells display pacemaker activity. In contrast to nerve and skeletal muscle cells, in which the membrane remains at constant resting potential unless the cell is stimulated, the cardiac autorhythmic cells do not have a rest- ing potential. Instead, they display pacemaker activity; that is, their membrane potential slowly depolarizes, or dri s, be- tween action potentials until threshold is reached, at which time the membrane res or has an action potential. An auto- Gap junction Action potential rhythmic cell membrane’s slow dri to threshold is called the Intercalated disc pacemaker potential ( Figure 9-7; see also p. 295). rough (c) Intercalated discs contain two types of membrane repeated cycles of dri and re, these autorhythmic cells cycli- junctions: mechanically important desmosomes cally initiate action potentials, which then spread throughout and electrically important gap junctions. the heart to trigger rhythmic beating without any nervous FIGURE 9-6 Organization of cardiac muscle fibers. Bun- stimulation. dles of cardiac muscle fibers are arranged spirally around the ventri- cle. Adjacent cardiac muscle cells are joined end to end by interca- PACEMAKER POTENTIAL AND ACTION POTENTIAL IN AUTO lated discs, which contain two types of specialized junctions: RHYTHMIC CELLS Complex interactions of several di erent desmosomes, which act as spot rivets mechanically holding the cells ionic mechanisms are responsible for the pacemaker potential. together; and gap junctions, which permit action potentials to e most important changes in ion movement that give rise to spread from one cell to adjacent cells. Cardiac Physiology 309 occurs through the open If channels, further contributing to the 20 dri toward threshold. e third ionic contribution to pacemaker potential is in- 10 PK+; PCa2+, L Self-induced action potential creased Ca2 entry. In the second half of the pacemaker poten- Membrane potential (mV) 0 tial, the If channels close and transient Ca2 channels (T-type 10 Ca2 channels), one of two types of voltage-gated Ca2 chan- Ca 2+ in K o + ut 20 nels, open before the membrane reaches threshold. (“T” stands Threshold 30 PCa2+, L; potential for transient.) e resultant brief in ux of Ca2 further depolar- PCa2+,T 2+ izes the membrane, bringing it to threshold, at which time the 40 Ca n + i transient Ca2 channels close. 50 PCa2+, T; Na Once threshold is reached, the rising phase of the action po- PNa+, f in Slow 60 depolarization tential occurs in response to activation of a long-lasting, voltage- PNa+, f; gated Ca2 channel (L-type Ca2 channel; “L” standing for long- PK+ (pacemaker potential) lasting) and a subsequently large in ux of Ca2. e Ca2 -induced Time (msec) rising phase of a cardiac pacemaker cell di ers from that in nerve and skeletal muscle cells, where Na in ux rather than Ca2 in- KEY ux swings the potential in the positive direction. f = Funny channels e falling phase is the result, as usual, of the K e ux that T = Transient-type channels occurs when K permeability increases on activation of voltage- L = Long-lasting channels gated K channels, coupled with closure of the L-type Ca2 channels. A er the action potential is over, slow closure of these FIGURE 9-7 Pacemaker activity of cardiac autorhythmic cells. The first half of the pacemaker potential is the result of si- K channels contributes to the next slow depolarization to multaneous opening of unique funny channels, which permits in- threshold. ward Na current, and closure of K channels, which reduces out- ward K current. The second half of the pacemaker potential is the result of opening of T-type Ca2 channels. Once threshold is The sinoatrial node is the normal reached, the rising phase of the action potential is the result of pacemaker of the heart. opening of L-type Ca2 channels, whereas the falling phase is the e specialized noncontractile cardiac cells capable of auto- result of opening of K channels. rhythmicity lie in the following speci c sites ( Figure 9-8): 1. e sinoatrial node (SA node), a small, specialized region in the right atrial wall near the opening of the superior vena cava. 2. e atrioventricular node (AV node), a small bundle of the pacemaker potential are (1) an increased inward Na cur- specialized cardiac muscle cells located at the base of the right rent, (2) a decreased outward K current, and (3) an increased atrium near the septum, just above the junction of the atria and inward Ca2 current. ventricles. To elaborate, the initial phase of the slow depolarization to 3. e bundle of His (atrioventricular bundle), a tract of threshold is caused by net Na entry through a type of voltage- specialized cells that originates at the AV node and enters the gated channels found only in cardiac pacemaker cells. Typically interventricular septum. Here, it divides to form the right and voltage-gated channels open when the membrane becomes less le bundle branches that travel down the septum, curve around negative (depolarizes), but these unique channels open when the tip of the ventricular chambers, and travel back toward the the potential becomes more negative (hyperpolarizes) at the atria along the outer walls. end of repolarization from the previous action potential. Be- 4. Purkinje bers, small terminal bers that extend from the cause of their unusual behavior, they are called funny, or If, bundle of His and spread throughout the ventricular myocar- channels. When one action potential ends and the If channels dium much like small twigs of a tree branch. open, the resultant depolarizing net inward Na current through these open channels starts immediately moving the pacemaker cell’s membrane potential toward threshold once again. NORMAL PACEMAKER ACTIVITY Because these various auto- e second ionic mechanism that contributes to this pace- rhythmic cells have di erent rates of slow depolarization to maker potential is a progressive reduction in the passive out- threshold, the rates at which they are normally capable of gen- ward ux of K. In cardiac autorhythmic cells, permeability to erating action potentials also di er (▲ Table 9-1). e heart K does not remain constant between action potentials as it cells with the fastest rate of action potential initiation are local- does in nerve and skeletal muscle cells. e K channels that ized in the SA node. Once an action potential occurs in any opened during the falling phase of the preceding action poten- cardiac muscle cell, it is propagated throughout the rest of the tial slowly close at negative potentials. is slow closure gradu- myocardium via gap junctions and the specialized conduction ally diminishes the out ow of positive potassium ions down system. erefore, the SA node, which normally has the fastest their concentration gradient. As a result, the rate of K e ux rate of autorhythmicity, at 70 to 80 action potentials per minute, slowly declines at the same time the slow inward leak of Na drives the rest of the heart at this rate and thus is known as the 310 Chapter 9 Interatrial Interatrial pathway pathway Sinoatrial Atrioventricular (SA) node (AV) node SA node AV node Right atrium Left atrium Right atrium Left atrium Internodal Left branch Internodal Bundle pathway of bundle pathway of His of His Electrically Right ventricle Left ventricle nonconductive Left ventricle fibrous tissue Right branch Purkinje Right ventricle Purkinje of bundle of His fibers fibers (a) Specialized conduction system of the heart (b) Spread of cardiac excitation FIGURE 9-8 Specialized conduction system of the heart and spread of cardiac excitation. An action potential initiated at the SA node first spreads throughout both atria. Its spread is facilitated by two specialized atrial conduction pathways, the interatrial and internodal path- ways. The AV node is the only point where an action potential can spread from the atria to the ven- tricles. From the AV node, the action potential spreads rapidly throughout the ventricles, hastened by a specialized ventricular conduction system consisting of the bundle of His and Purkinje fibers. pacemaker of the heart. at is, the entire heart becomes ex- Purkinje bers) at 30 mph. If all these cars are joined, the en- cited, triggering the contractile cells to contract and the heart to gine that can travel at 70 mph will pull the rest of the cars at that beat at the pace or rate set by SA node autorhythmicity, nor- speed. e engines that can travel at lower speeds on their own mally at 70 to 80 beats per minute. e other autorhythmic will be pulled at a faster speed by the fastest engine and there- tissues cannot assume their own naturally slower rates, because fore cannot assume their own slower rate as long as they are they are activated by action potentials originating in the SA being driven by a faster engine. e other 97 cars (nonauto- node before they can reach threshold at their own, slower rhythmic, contractile cells), being unable to move on their own, rhythm. will likewise travel at whatever speed the fastest engine pulls e following analogy shows how the SA node drives the them. rest of the heart at its own pace. Suppose a train has 100 cars, 3 of which are engines capable of moving on their own; the ABNORMAL PACEMAKER ACTIVITY If for some reason other 97 cars must be pulled ( Figure 9-9a). One engine (the the fastest engine breaks down (SA node damage), the SA node) can travel at 70 miles/hour (mph) on its own, another next-fastest engine (AV node) takes over and the entire engine (the AV node) at 50 mph, and the last engine (the train travels at 50 mph; that is, if the SA node becomes non- functional, the AV node assumes pacemaker activity ( Figure 9-9b). e non–SA nodal autorhythmic tissues are latent pace- makers that can take over, although at a lower rate, if the nor- mal pacemaker fails. ▲ TABLE - Normal Rate of Action If impulse conduction becomes blocked between the atria Potential Discharge and the ventricles, the atria continue at the typical rate of in Autorhythmic Tissues 70 beats per minute, and the ventricular tissue, not being driven by the faster SA nodal rate, assumes its own much slower auto- of the Heart rhythmic rate of about 30 beats per minute, initiated by the ventricular autorhythmic cells (Purkinje bers). is situation Action Potentials is like a breakdown of the second engine (AV node) so that the Tissue per Minute* lead engine (SA node) becomes disconnected from the slow SA node (normal pacemaker) 70–80 third engine (Purkinje bers) and the rest of the cars ( Figure AV node 40–60 9-9c). e lead engine (and cars connected directly to it; that is, Bundle of His and Purkinje fibers 20–40 the atrial cells) continues at 70 mph while the rest of the train proceeds at 30 mph. is complete heart block occurs when *In the presence of parasympathetic tone; see p. 240 and p. 327. the conducting tissue between the atria and ventricles is dam- aged, as for example during a heart attack, and becomes non- Cardiac Physiology 311 70 mph SA node AV node Purkinje fiber Contractile (“working”) cells 70 mph 50 mph 30 mph (a) Normal pacemaker activity: Whole train will go 70 mph (heart rate set by SA node, the fastest autorhythmic tissue). 50 mph AV node Purkinje fiber 50 mph 30 mph de A no h S mp 70 SA node “derailed” (b) Takeover of pacemaker activity by AV node when the SA node is nonfunctional: Train will go 50 mph (the next fastest autorhythmic tissue, the AV node, will set the heart rate). 70 mph SA node 30 mph Purkinje fiber 70 mph 30 mph de no h AV mp AV node “derailed” 50 (c) Takeover of ventricular rate by the slower ventricular autorhythmic tissue in complete heart block: First part of train will go 70 mph; last part will go 30 mph (atria will be driven by SA node; ventricles will assume own, much slower rhythm). Ectopic focus 140 mph SA node AV node Purkinje fiber 70 mph 50 mph 140 mph (d) Takeover of pacemaker activity by an ectopic focus: Train will be driven by ectopic focus, which is now going faster than the SA node (the whole heart will be driven more rapidly by an abnormal pacemaker). FIGURE 9-9 Analogy of pacemaker activity. In complete heart block (c), when ventricular rate is taken over by the slower ventricular autorhythmic tissue, the atrial rate (not shown) is still driven by the SA node. functional. A ventricular rate of 30 beats per minute will sup- that spreads throughout the rest of the heart before the SA port only a very sedentary existence; in fact, the patient usually node can initiate a normal action potential (ectopic means becomes comatose. “out of place”). An occasional abnormal impulse from a ven- When a person has an abnormally low heart rate, as in SA tricular ectopic focus produces a premature ventricular node failure or heart block, an arti cial pacemaker can be contraction (PVC). If the ectopic focus continues to dis- used. Such an implanted device rhythmically generates im- charge at its more rapid rate, pacemaker activity shi s from pulses that spread throughout the heart to drive both the atria the SA node to the ectopic focus. e heart rate abruptly be- and ventricles at the typical rate of 70 beats per minute. comes greatly accelerated and continues this rapid rate for a Occasionally an area of the heart, such as a Purkinje ber, variable time period until the ectopic focus returns to nor- becomes overly excitable and depolarizes more rapidly than mal. Such overly irritable areas may be associated with or- the SA node. ( e slow engine suddenly goes faster than the ganic heart disease, but more frequently they occur in re- lead engine; see Figure 9-9d). is abnormally excitable sponse to anxiety, lack of sleep, or excess ca eine, nicotine, or area, an ectopic focus, initiates a premature action potential alcohol consumption. 312 Chapter 9 We now turn attention to how an action potential, once 3. e pair of atria and pair of ventricles should be functionally initiated, is conducted throughout the heart. coordinated so that both members of the pair contract simultane- ously. is coordination permits synchronized pumping of blood into the pulmonary and systemic circulation. The spread of cardiac excitation is coordinated to ensure efficient pumping. e normal spread of cardiac excitation is carefully orches- trated to ensure that these criteria are met and the heart func- Once initiated in the SA node, an action potential spreads tions e ciently, as follows (see Figure 9-8b). throughout the rest of the heart. For e cient cardiac function, the spread of excitation should satisfy three criteria: ATRIAL EXCITATION An action potential originating in the SA 1. Atrial excitation and contraction should be complete before node rst spreads throughout both atria, primarily from cell to the onset of ventricular contraction. Complete ventricular lling cell via gap junctions. In addition, several poorly delineated, requires that atrial contraction precede ventricular contraction. specialized conduction pathways speed up conduction of the During cardiac relaxation, the AV valves are open, so venous impulse through the atria. blood entering the atria continues to ow directly into the ven- e interatrial pathway extends from the SA node within tricles. Almost 80% of ventricular lling occurs by this means the right atrium to the le atrium. Because this pathway rapidly before atrial contraction. When the atria do contract, more transmits the action potential from the SA node to the path- blood is squeezed into the ventricles to complete ventricular way’s termination in the le atrium, a wave of excitation can lling. Ventricular contraction then occurs to eject blood from spread across the gap junctions throughout the le atrium at the heart into the arteries. the same time as excitation is similarly spreading throughout If the atria and ventricles were to contract simultaneously, the right atrium. is ensures that both atria become depolar- the AV valves would close immediately because ventricular ized to contract simultaneously. pressures would greatly exceed atrial pressures. e ventricles e internodal pathway extends from the SA node to the have much thicker walls and, accordingly, can generate more AV node. e AV node is the only point of electrical contact pressure. Atrial contraction would be unproductive because the between the atria and ventricles; in other words, because the atria could not squeeze blood into the ventricles through closed atria and ventricles are structurally connected by electrically valves. erefore, to ensure complete lling of the ventricles— nonconductive brous tissue, the only way an action potential in to obtain the remaining 20% of ventricular lling that occurs the atria can spread to the ventricles is by passing through the during atrial contraction—the atria must become excited and AV node. e internodal conduction pathway directs the spread contract before ventricular excitation and contraction. During of an action potential originating at the SA node to the AV node a normal heartbeat, atrial contraction occurs about 160 msec to ensure sequential contraction of the ventricles following atrial before ventricular contraction. contraction. Hastened by this pathway, the action potential ar- 2. Excitation of cardiac muscle bers should be coordinated to rives at the AV node within 30 sec of SA node ring. ensure that each heart chamber contracts as a unit to pump e - 0.09 sec ciently. If the muscle bers in a heart chamber became excited CONDUCTION BETWEEN THE ATRIA AND THE VENTRICLES e and contracted randomly rather than contracting simultane- action potential is conducted relatively slowly through the AV ously in a coordinated fashion, they would be unable to eject node. is slowness is advantageous because it allows time blood. A smooth, uniform ventricular contraction is essential for complete ventricular lling. e impulse is delayed about to squeeze out the blood. As an analogy, assume you have a 100 msec (the AV nodal delay), which enables the atria to be- basting syringe full of water. If you merely poke a nger here or come completely depolarized and to contract, emptying their there into the rubber bulb of the syringe, you will not eject contents into the ventricles, before ventricular depolarization much water. However, if you compress the bulb in a smooth, and contraction occur. coordinated fashion, you can squeeze out the water. In a similar manner, contraction of isolated cardiac VENTRICULAR EXCITATION A er the AV nodal delay, the im- muscle bers is not successful in pumping blood. Such pulse travels rapidly down the septum via the right and le random, uncoordinated excitation and contraction of branches of the bundle of His and throughout the ventricular the cardiac cells is known as brillation. Fibrillation of the myocardium via the Purkinje bers. e network of bers in ventricles is much more serious than atrial brillation. Ven- this ventricular conduction system is specialized for rapid tricular brillation rapidly causes death, because the heart can- propagation of action potentials. Its presence hastens and coor- not pump blood into the arteries. is condition can o en be dinates the spread of ventricular excitation to ensure that the corrected by electrical de brillation, in which a very strong ventricles contract as a unit. e action potential is transmitted electrical current is applied on the chest wall. When this current through the entire Purkinje ber system within 30 msec. reaches the heart, it stimulates (depolarizes) all parts of the Although this system carries the action potential rapidly heart simultaneously. Usually the rst part of the heart to re- to a large number of cardiac muscle cells, it does not terminate cover is the SA node, which takes over pacemaker activity, once on every cell. e impulse quickly spreads from the excited again initiating impulses that trigger the synchronized contrac- cells to the rest of the ventricular muscle cells by means of gap tion of the rest of the heart. junctions. Cardiac Physiology 313 e ventricular conduction system is more highly orga- nized and more important than the interatrial and internodal PNa+; PK+ (transient) conduction pathways. Because the ventricular mass is so much K 30 + ut PCa2+, L; PK+ (both transient and leaky) larger than the atrial mass, a rapid conduction system is crucial o fas to hasten the spread of excitation in the ventricles. Purkinje t Ca2+ in slow bers can transmit an action potential six times faster than the Plateau PCa2+, L; PK+ Membrane potential (mV) ventricular syncytium of contractile cells could. If the entire 0 phase of (ordinary ventricular depolarization process depended on cell-to-cell action voltage-gated) spread of the impulse via gap junctions, the ventricular tissue potential immediately next to the AV node would become excited and K+ out fast Na+ in fast contract before the impulse had even passed to the heart apex. is, of course, would not allow e cient pumping. Rapid con- duction of the action potential down the bundle of His and its swi , di use distribution throughout the Purkinje network Threshold lead to almost simultaneous activation of the ventricular myo- PNa+ potential 70 cardial cells in both ventricular chambers, which ensures a PK+ (ordinary single, smooth, coordinated contraction that can e ciently voltage-gated); PK+ (leaky) eject blood into both the systemic and pulmonary circulations 90 250 at the same time. Time (msec) The action potential of cardiac contractile FIGURE 9-10 Action potential in contractile cardiac mus- cells shows a characteristic plateau. cle cells. The action potential in cardiac contractile cells differs considerably from the action potential in cardiac autorhythmic cells e action potential in cardiac contractile cells, although initi- (compare with Figure 9-7). The rapid rising phase of the action po- ated by the nodal pacemaker cells, varies considerably in ionic tential in contractile cells is the result of Na entry on opening of mechanisms and shape from the SA node potential (compare fast Na channels at threshold. The early, brief repolarization after Figures 9-7 and 9-10). Unlike the membrane of autorhythmic the potential reaches its peak is because of limited K efflux on cells, the membrane of contractile cells remains essentially at opening of transient K channels, coupled with inactivation of the rest at about –90 mV until excited by electrical activity propa- Na channels. The prolonged plateau phase is the result of slow gated from the pacemaker. Myocardial contractile cells have Ca2 entry on opening of L-type Ca2 channels, coupled with re- several subclasses of K channels. At resting potential, the type duced K efflux on closure of several types of K channels. The of K channel that is open is especially leaky.1 e resultant rapid falling phase is the result of K efflux on opening of ordinary voltage-gated K channels, as in other excitable cells. Resting po- outward movement of K through these channels keeps the tential is maintained by opening of leaky K channels. resting potential close to the K equilibrium potential at 90 mV. Once the membrane of a ventricular myocardial con- tractile cell is depolarized to threshold via current ow through gap junctions, an action potential is generated by a complicated 3. Unique to these cardiac muscle cells, the membrane poten- interplay of permeability changes and membrane potential tial is maintained at this near peak positive level for several changes as follows ( Figure 9-10): hundred milliseconds, producing a plateau phase of the action potential. In contrast, the short action potential of neurons and 1. During the rising phase of the action potential, the mem- skeletal muscle cells lasts 1 to 2 msec. Whereas the rising phase brane potential rapidly reverses to a positive value of of the action potential is brought about by activation of com- about 20 mV to 30 mV (depending on the myocardial cell) paratively “fast” Na channels and the early, brief repolarization as a result of activation of voltage-gated Na channels and Na arises from activation of fast, transient K channels, this plateau subsequently rapidly entering the cell, as it does in other excit- is maintained by two voltage-dependent permeability changes: able cells undergoing an action potential (see p. 92). ese are activation of “slow” L-type Ca2 channels and a marked de- the same type of double-gated Na channels found in nerve and crease in K permeability in the cardiac contractile cell mem- skeletal muscle cells. At peak potential, the Na permeability brane. ese permeability changes occur in response to the then rapidly plummets to its low resting value. sudden change in voltage during the rising phase of the action 2. At peak potential, another subclass of K channels tran- potential. Opening of the L-type Ca2 channels results in a slow, siently opens. e resultant limited e ux of K through these inward di usion of Ca2 because Ca2 is in greater concentra- transient channels brings about a brief, small repolarization as tion in the ECF. is continued in ux of positively charged the membrane becomes slightly less positive. Ca2 prolongs the positivity inside the cell and is primarily re- sponsible for the plateau portion of the action potential. is e ect is enhanced by the concomitant decrease in K permea- 1 For reasons beyond the scope of this book, this channel type is o - bility on closure of both the brie y opened transient K chan- cially known as an inward recti er K channel, and the “ordinary” nels and the leaky K channels open at resting potential. e K channel discussed shortly is called a delayed recti er K channel. resultant reduction in outward movement of positively charged 314 Chapter 9 K prevents rapid repolarization of the membrane and thus Action potential contributes to prolongation of the plateau phase. in cardiac mV 4. e rapid falling phase of the action potential results from contractile cell inactivation of the Ca2 channels and delayed activation of “or- Time dinary” voltage-gated K channels, yet another subclass of K channels identical to the ones responsible for repolarization in Travels down neurons and skeletal muscle cells. e decrease in Ca2 perme- T tubules ability diminishes the slow, inward movement of positive Ca2 , whereas the sudden increase in K permeability simultaneously promotes rapid outward di usion of positive K. us, as in other excitable cells, the cell returns to resting potential as K Entry of small Release of large amount leaves the cell. At resting potential, the ordinary voltage-gated amount of Ca2+ Ca2+ of Ca2+ from sarcoplasmic K channels close and the leaky K channels open once again. from ECF through 2+ reticulum through ryanodine L-type Ca2+ channels Ca2+-induced Ca2+-release channels Let’s now see how this action potential brings about con- Ca release traction. Ca2+ Ca2+ Ca2+ Ca2+ Ca2+ Ca entry from the ECF induces a much larger Ca2+ Cytosolic Ca2+ Ca release from the sarcoplasmic reticulum. In cardiac contractile cells, the L-type Ca2 channels lie primarily Ca2+ in the T tubules. As you just learned, these voltage-gated channels open during a local action potential. us, unlike in skeletal Troponin–tropomyosin muscle, Ca2 di uses into the cytosol from the ECF across the complex in thin T tubule membrane during a cardiac action potential. is enter- filaments pulled aside ing Ca2 triggers the opening of nearby ryanodine Ca2 -release channels in the adjacent lateral sacs of the sarcoplasmic reticu- lum (see p. 264). By means of this so-called Ca2 -induced Ca2 Cross-bridge cycling release, Ca2 entering the cytosol from the ECF induces a much between thick and larger release of Ca2 into the cytosol from the intracellular stores thin filaments ( Figure 9-11). e resultant local bursts of Ca2 release, known as Ca2 sparks, from the sarcoplasmic reticulum collectively in- crease the cytosolic Ca2 pool su ciently to turn on the contrac- Thin filaments slide tile machinery. Ninety percent of the Ca2 needed for muscle inward between thick contraction comes from the sarcoplasmic reticulum. is extra filaments supply of Ca2 , coupled with slow Ca2 removal processes, is responsible for the long period of cardiac contraction, which lasts about three times longer than the contraction of a single skeletal Contraction muscle ber (300 msec compared to 100 msec). is increased contractile time ensures adequate time to eject the blood. FIGURE 9-11 Excitation–contraction coupling in cardiac contractile cells. ROLE OF CYTOSOLIC Ca IN EXCITATION CONTRACTION COU PLING As in skeletal muscle, the role of Ca2 within the cytosol is to bind with the troponin–tropomyosin complex and physi- heart. Abnormal levels of K are most important clinically, fol- cally pull it aside to allow cross-bridge cycling and contraction lowed to a lesser extent by Ca2 imbalances. By changing the ( Figure 9-11) (see p. 260). However, unlike skeletal muscle, in concentration gradient between the ICF and ECF, changes in which su cient Ca2 is always released to turn on all the cross the ECF K concentration (either above or below normal) can bridges, in cardiac muscle the extent of cross-bridge activity var- lead to abnormalities in cardiac excitation by changing the rest- ies with the amount of cytosolic Ca2. As we will show, various ing potential (see p. 535). regulatory factors can alter the amount of cytosolic Ca2. An elevated ECF Ca2 concentration augments the strength Removal of Ca2 from the cytosol by energy-dependent of cardiac contraction by prolonging the plateau phase of the mechanisms in both the plasma membrane and sarcoplasmic action potential and by increasing the cytosolic concentration reticulum restores the blocking action of troponin and tropo- of Ca2. Contractions tend to be of longer duration, with little myosin, so contraction ceases and the heart muscle relaxes. time to rest between contractions. Some drugs alter cardiac function by in uencing Ca2 movement across the myocardial INFLUENCE OF ALTERED ECF K AND Ca CONCENTRA cell membranes. For example, Ca2 -channel blocking agents, TIONS Not surprisingly, changes in the ECF concentra- such as verapamil, block Ca2 in ux through the L-type Ca2 tion of K and Ca2 can have profound e ects on the channels during an action potential, reducing the force of car- Cardiac Physiology 315 channels that were activated during the initial Na in ux of the Action Contractile potential response rising phase; that is, the double-gated Na channels are in their closed and not capable of opening conformation (see p. 92). Not 30 until the membrane recovers from this inactivation process (when the membrane has already repolarized to resting), can Relative cardiac muscle fiber tension the Na channels be activated once again to begin another ac- tion potential. 0 Membrane potential (mV) The ECG is a record of the overall spread of electrical activity through the heart. e electrical currents generated by cardiac muscle during de- Refractory polarization and repolarization spread into the tissues around period the heart and are conducted through the body uids. A small part of this electrical activity reaches the body surface, where it can be detected using recording electrodes. e record pro- 70 duced is an electrocardiogram, or ECG. (Alternatively, the abbreviation EKG is o en used, from the ancient Greek word 90 “kardia” instead of the Latin “cardia” for “heart.”) Remember three important points when considering what 0 100 200 300 an ECG represents: Time (msec) 1. An ECG is a recording of that part of the electrical activity FIGURE 9-12 Relationship of an action potential and the present in body uids from the cardiac impulse that reaches the refractory period to the duration of the contractile re- body surface, not a direct recording of the actual electrical ac- sponse in cardiac muscle. tivity of the heart. 2. e ECG is a complex recording representing the overall spread of activity throughout the heart during depolarization diac contraction. Other drugs, such as digitalis, increase cardiac and repolarization. It is not a recording of a single action poten- contractility by inducing an accumulation of cytosolic Ca2. tial in a single cell at a single point in time. e record at any given time represents the sum of electrical activity in all the A long refractory period prevents cardiac muscle cells, some of which may be undergoing action potentials while others may not yet be activated. For example, tetanus of cardiac muscle. immediately a er the SA node res, the atrial cells are undergo- Like other excitable tissues, cardiac muscle has a refractory pe- ing action potentials while the ventricular cells are still at rest- riod. During the refractory period, a second action potential ing potential. At a later point, the electrical activity will have cannot be triggered until an excitable membrane has recovered spread to the ventricular cells while the atrial cells will be repo- from the preceding action potential. In skeletal muscle, the re- larizing. erefore, the overall pattern of cardiac electrical ac- fractory period is very short compared with the duration of the tivity varies with time as the impulse passes throughout the resulting contraction, so the ber can be restimulated before heart. the rst contraction is complete to produce summation of con- 3. e recording represents comparisons in voltage detected tractions. Rapidly repetitive stimulation that does not let the by electrodes at two di erent points on the body surface, not muscle ber relax between stimulations results in a sustained, the actual potential. For example, the ECG does not record a maximal contraction known as tetanus (see Figure 8-18, potential at all when the ventricular muscle is either completely p. 271). depolarized or completely repolarized; both electrodes are In contrast, cardiac muscle has a long refractory period that “viewing” the same potential, so no di erence in potential be- lasts about 250 msec because of the prolonged plateau phase of tween the two electrodes is recorded. the action potential. is is almost as long as the period of con- traction initiated by the action potential; a cardiac muscle ber e exact pattern of electrical activity recorded from the contraction averages about 300 msec ( Figure 9-12). Conse- body surface depends on the orientation of the recording quently, cardiac muscle cannot be restimulated until contrac- electrodes. Electrodes may be loosely thought of as “eyes” tion is almost over, precluding summation of contractions and that “see” electrical activity and translate it into a visible re- tetanus of cardiac muscle. is is a valuable protective mecha- cording, the ECG record. Whether an upward de ection or nism, because the pumping of blood requires alternate periods downward de ection is recorded is determined by the way of contraction (emptying) and relaxation ( lling). A prolonged the electrodes are oriented with respect to the current ow in tetanic contraction would prove fatal. e heart chambers the heart. For example, the spread of excitation across the could not be lled and emptied again. heart is “seen” di erently from the right arm, from the le e chief factor responsible for the long refractory period is leg, or from a recording directly over the heart. Even though inactivation, during the prolonged plateau phase, of the Na the same electrical events are occurring in the heart, di erent 316 Chapter 9 Lead I: Right arm to left arm aVR: right arm aVL: left arm Lead II: Lead III: Right arm to Left arm to left leg left leg aVF: left leg V1 V2 V6 V3 V V5 4 Ground electrode (a) Limb leads