ZOO 114-1 Principles of Cell Biology and Genetics 2023/2024 PDF
Document Details
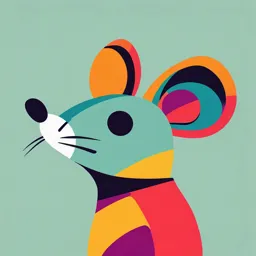
Uploaded by LyricalMoose
University of Ibadan
2024
Dr H.O.Awobode
Tags
Summary
These lecture notes cover the principles of cell biology and genetics, including cell structure and function, eukaryotic and prokaryotic cells, cell theory, cell membranes, organelles, and the cytoskeleton. The 2023-2024 session, with Dr H.O.Awobode as lecturer, is also documented.
Full Transcript
ZOO 111 : Principles of Cell Biology and Genetics 2023/2024 Session Lecturer: Dr H.O.Awobode Overview History of the cell Cell Structure and Function Prokaryotic and Eukaryotic cell Plant and Animal Cell. Texts 1. Biology : The Dynamic science (2008) Russell,...
ZOO 111 : Principles of Cell Biology and Genetics 2023/2024 Session Lecturer: Dr H.O.Awobode Overview History of the cell Cell Structure and Function Prokaryotic and Eukaryotic cell Plant and Animal Cell. Texts 1. Biology : The Dynamic science (2008) Russell, Wolfe, Hertz, starr and McMillan. Thomson Books CA, USA. What is a Cell A cell is the basic unit of structure and function in a living thing. Each cell in the body shares the characteristics of all living things. History of the Cell An English scientist Robert Hooke (1635–1703) was the first to record his observations of cells. In 1663, he took a thin slice of cork and placed it under a microscope that he built himself. Described the cork as” little boxes” looking like a honey comb. It became clear that all living things are made up of cells. The cells ALL have certain common features. The work of Hooke, Leeuwenhoek, Schleiden, Schwann, Virchow, and others led to important theory in life science: The Cell Theory. The theory is based on the concept that cells are the basic unit of life. The cell theory explains the relationship between cells and living things. Cell Theory The ideas of the theory include: Cells form the building blocks of living things. Cells arise only by the division of existing cells. Cells contain genetic (inherited) information which control it’s activities. Physiological processes eg metabolism takes place in the cell. Cells are capable of independent existence under suitable condition. Source :- cpo science.com The Cell Cells are small membrane bound units filled with aqueous solution of chemicals. The cell is made up of organelles Cells create copies of themselves by growing and dividing. All cells share certain common characteristics. All cells are surrounded by a cell membrane. All cells contain organelles. All cells contain cytoplasm. All cells contain genetic material. There are many types, shapes and sizes of cells. A cell consist of 3 major parts: A well defined boundary, The cell membrane. In the middle is a prominent rounded body called The nucleus A transparent substance around the nucleus which fills the cell is Cytoplasm. Types of Cells Prokaryotic cells do not have a nucleus. Most are single celled organisms, though some form strings, clusters or multi- cellular structures. They have a tough protective coat-The cell wall. Under the cell wall is the plasma membrane enclosing the cytoplasm. The cytoplasm contains DNA, RNA, proteins etc. They inhabit a wide variety of environments/ ecological niches. Scientists believe that all life on Earth came from ancient cells of this type. Only bacteria and archae are prokaryotic. Eukaryotic cells have a nucleus and membrane-covered organelles. They are about ten times larger than prokaryotic cells. Higher level of complexity. Animals, plants, fungi, and protists are all made of eukaryotic cells. Many classes form multicellular organisms. Comparing cell types. Source: Cpo.com LOOK IN G IN SIDE THE CELL Cell Membrane The cell membrane is a thin layer that separates the inside of the cell from its outside environment. It is selectively permeable, controlling exchange of material between cell and environment. It keeps the cytoplasm inside while allowing waste products out. Each memb is approx 10mm thick and is made of 3 layers(double layer of phospholipid and protein). Contains about 45% protein, 45% lipids and and 10% carbohydrates. Fluid-Mosaic model of the cell membrane Cell Membrane Most of the lipid is phospholipid. Each consist of a hydrophobic tail of 2 fatty acids and a hydrophilic phosphate head.) Phospholipids arrange themselves as layer of 2 molecules thick (bilayer). Hydrophobic tail point inwards (away from the water) both inside and outside the cell. Phospholipids allow passage of certain lipid soluble substances through the membrane The protein molecules are variable in structure and function. Protein spanning the width of membrane are Intrinsic proteins. Some act as carrier substances transporting molecules across the membrane. Extrinsic proteins are limited to outer or inner surface of membrane. They combine with CHO to form glycoproteins. They act along with glycolipids as chemical receptors on the cell. FLU ID M OSAIC M OD EL Fluid – the plasma membrane is the consistency of olive oil at body temperature, due to unsaturated phospholipids. (cells differ in the amount of unsaturated to saturated fatty acid tails) Most of the lipids and some proteins drift laterally on either side. Phospholipids do not switch from one layer to the next. Cholesterol affects fluidity: at body temperature it lessens fluidity by restraining the movement of phospholipids, at colder temperatures it adds fluidity by not allowing phospholipids to pack close together. Mosaic – membrane proteins form a collage that differs on either side of the membrane with hydrophilic portions facing out and hydrophobic portions facing in. Provides the functions of the membrane Internal membranes Membranes found within the interior of almost all kinds of cells. Enclose organelles within the cell. Similar in appearance to cell membrane under electron microscope. They contain lipid and proteins though different from cell membrane. Differences in composition reflect function of the membrane. They regulate the traffic of molecules and ions in and out of the cell. Functions of Cell membrane Internal membrane Barrier between cell and the Acts as reaction surface. surrounding. Isolate different chemical Mechanical support to cell. reactions. Flexible and allows movement, growth and cell division. Intracellular transport system. It is self–sealing and can divide without bursting. Separate intra cellular Control movement of materials. compartments. Receptor site for hormones and neurotransmitters. Recognition of other cells. Transmission of nerve impulse. Insulation of nerves Mitochondria Present in all types of eukaryotic cells Surrounded by 2 membranes. Smooth outer membrane. Folded inner membrane with layers called cristae. Matrix is within the inner membrane. intermembrane space is located between the two membranes Contain their own DN A. Mitochondria are called the “powerhouses” of cells. They produce much of the energy a cell needs to carry out its functions. Contain oxidative metabolism enzymes for transferring the energy within macromolecules to ATP. Endoplasmic Reticulum A system of flattened membrane –bound sacs. The sacs, are fluid filled. They transport protein in the cell. Some regions are covered with bead-like structures called ribosomes. These are called Rough ER. Smooth ER has no ribosomes. Ribosomes Tiny organelles consisting of two sub-units: one larger than the other. They are site of protein synthesis. They release the proteins into the ER. They may be either attached to endoplasmic reticulum or free in the cytoplasm. Golgi bodies A stack of flattened membrane- bound sacs called cisternae. Stack forms network in animals. A processing and packaging structure- receives material from ER. N ew membrane is continuously added to one end. It buds off at the opposite end to form vessicles. It is involved in the formation of lysosomes Helps cell materials eg. Enzymes to be secreted from the cell in vessicles. Nucleus The largest cell organelle. The nucleus is covered with a membrane called nuclear envelope. The envelope is perforated – has pores that allows materials to pass in and out. It contains chromatin, chromatin contains DN A. Two forms of chromatin: i. euchromatin-stains lightly and contain active DN A. Ii. Heterochromatin-stains deeply and contain inactive DN A. It’s often called the “control center” of the cell because it contains DN A which is the molecule of inheritance. Contain nucleolus which manufacture ribosomes. The nucleolus also acts as a storage area for materials Lysosomes Lysosomes contain enzymes and are involved in autolysis. Lysosomes pick up, food, and old redundant organelles, break them into reusable molecules. They also digest bacteria and content of vacuoles ingested by white blood cells. Cytoskeleton The cytoskeleton is a series of fine fibres made from proteins. They are anchored at one end of plasma membrane or radiate from a site adjacent to the nucleus. It provides structure to the cell and gives it its shape. There are 3 main types: Microfilament. Microtubules Intermediate filament. Microfilaments: Thinnest filament found in all eukaryotic cells. 5-6 nm in diameter Made of protein Actin Large nos form clusters/ networks at various points in the cell. They are associated with movement eg. separation of daughter cells after cell division, muscle contraction etc. Aid cell in cytoplasmic streaming or cell migration. Microtubules Thickest filaments with variable length. Straight, hollow, rigid cylinders of protein. Found in most plant and animal cells. They provide rigidity to parts of cell where they are located. Microtubles called Spindles are important in cell division- they pull duplicated chromosomes in opposite direction and distribute to daughter cells. Used in construction of centrioles, basal bodies, cilia and flagella. Intermediate Filaments Intermediate between microfilaments and microtubules. They provide supporting framework within the cell eg nucleus in cell are held in position by intermediate filaments. Found in all types of muscle cells, axons. ***The three types of filament along with other proteins gives strength, maintains shape and guides movement of cells**** Centrioles Cell wall Paired cylinders composed of Found in plants. a complex arrangement of microtubules. Provide structure and support for the plant. Involved in cell division and locomotion by cilia and Made of cellulose flagella. Vacuoles In plants they are large. Store cell sap Chloroplast An organelle found only in plants and some algae. They are large complex organelles bounded by double membrane. Disc shaped 5-8um diameter X 2-4um thick. Contains a system of internal membrane called stroma. The internal membrane contains phospholipids and proteins. that contains a stack of green pigment called chlorophyll. Chloroplast contains both food mols and oxygen for mitochondrial use. Contains its DN A and reproduce by dividing into 2. ZOO 114 : Principles of Cell Biology and Genetics 2023/2024 Session Lecturer: Dr H.O.Awobode Overview Cellular basis of organization. Cell Tissue Organ Organ Systems. Levels of organization. The Cell Cells are small membrane bound units filled with aqueous solution of chemicals. The cell is made up of organelles Cells create copies of themselves by growing and dividing. All cells share certain common characteristics. All cells are surrounded by a cell membrane. All cells contain organelles. All cells contain cytoplasm. All cells contain genetic material. Simplest forms of life are solitary cells. Single celled (unicellular) organisms are made up of ONE cell. In colonial organisms e.g Volvox, the individual cells are free-living and can survive on their own if separated. Colonial Theory proposes that cooperation among cells of same species led to development of multicellular organisms. Higher organisms are many-celled (multicellular) organisms. Simplest multicellular organisms are made of specialized cells working together. If separated from each other, the individual cells cannot survive by itself. These groups/ communities of cells are derived from a single cell. Individual cells perform specialized functions coordinated by complex communication systems. Cells performing the same function exist as groups G roup of cells carrying out same function are TISSU ES. G roup of tissues form OR G ANS and group of organs form OR G AN SYSTEM S. Tissues A group of connected cells with same structure and function in an organism. They work together to carry out one or more activities e.g absorbing of nutrients from digestion. Organs A group of tissues that have a specific function. Organs can be primitive as in flat worms or complex like mammalian kidneys which integrates several types of tissues into a specialized organ. Organ systems These are group of organs acting together to carry out major functions in a coordinated manner. Each organ of the system carry out specific tasks e.g digestive system beginning from the mouth and ending at the anus. Some organs function in more than one organ system e.g pancreas is part of both endocrine and digestive system. Cellular Environment Cells acquire materials and energy from their surroundings. Acquired materials are transformed by metabolism and end products are returned to the environment. cell are bathed in a surrounding fluid called extracellular fluid (ECF). ECF contains all mols or ions required by the cell. Wastes from the cell are deposited in it. Made up of mainly water in which mol and ions are dissolved. Gases - O2, CO2 are most important. Inorganic ions include Na+, Cl - K+, Ca++ , HCO3-, PO43- are in substancial quantities. Some others required in minute quantities include Cu++ , Zn++ , Mn++ ,Co+ + called trace elements. They are important for enzyme activity. Iodine and flouride are important for teeth, bones and normal growth. Organic compounds such as food and vitamins are found in the extracellular fluid. ECF of multicellular organisms also contain Hormones. ECF remove excretory wastes from cell. The H + (pH) con of the ECF and temperature are important in the welfare of the cell. The 5 ways by which cells exchange materials with the ECFare: diffusion, Osmosis, active transport, endocytosis and exocytosis There are several types of tissues: 1. Epithelial– squamous, cuboidal, columnar 2. Connective – loose, cartilage, bone, adipose, blood 3. Muscle 4. Nervous Types of Tissues 1. Epithelial Tissues Sheet-like layers of cells joined together. They have little extracellular matrix(ECM) between them They cover body surfaces and surfaces of internal organs, cavities and ducts within the body. They protect body surfaces from bacterial and viral invasion. Epithelia are classified as : Simple – formed from single layer of cells. Stratified – formed from multiple cell layers. The shape of the epithelial cells my be i. squamous (mosaic, flattened and spread out). ii. ii. Cuboidal like dice or cubes). iii. iii. Columnar (elongated with long axis perpendicular to the layer) Epithelia usually gives rise to specialized excretory cells Some form structures called glands while others are scattered among non secretory cells within epithelium. Connective Tissue Animal bodies contain connective tissues. Connective tissues support other body tissues, transmit mechanical strength and can act as filters. They consist of cells forming networks or layers around body structures separated by ECM. The mechanical properties of the tissue depend on the type and quantity of its ECM. The ECM ranges from fluid(blood) to hard and crystalline(bone). V ertebrates have six major types of connective tissue: loose connective tissue, fibrous connective tissue, cartilage, bone, adipose tissue and blood. Each has a function related with its structure 1. Loose connective Tissue Sparsely distributed cells surrounded by open network of collagen and glycoprotein fibres. It supports epithelia and forms a network around blood vessels, nerves and some internal organs They reinforce deep layers of the skin They hold abdominal organs place and provide smooth surfaces to prevent abrasion between structures as body moves. 2. Fibrous connective Tissue fibroblasts are among dense masses of collagen and elastin fibres The arrangement provides tensile strength and elasticity e.g in tendons attaching muscles to bones and ligaments. Cornea of the eye is a transparent fibrous connective tissue. Cartilage Consists of cells called chonriocytes. These are sparsely distributed and surrounded by network of collagen fibres embedded in tough elastin matrix of glycoprotein (chondroitin sulfate). Elastin is also present in some form of cartilage. The elasticity of cartilage allows it to resist compression and stay resilient. In humans cartilage supports the larynx, trachea and smaller air passages in the lungs. It forms discs cushioning the vertebrae in the spinal column and the smooth, slippery capsule around the ends of bones in joints e.g hip and knee. Cartilage also serves as precursor to bone during embryonic development e.g sharks and rays have their entire skeleton as cartilage in adults. B one This is the most dense form of connective tissue. Bone forms the skeleton which supports the body, protects softer body structures e.g brain, and contributes to movements of the body. Mature bone consists primarily of cells called ostocytes (osteon-bone), embedded in an ECM containing collagen fibres and glycoprotein impregnated with hydroxyapatite, a calcium phosphate mineral. Collagen fibres gives bone tensile strength and elasticity. The hydroxyapatite resists compression and allows bones to support body weight. Cells, osteoblasts produce collagen and mineral bone. as much as 85% of bone weight is mineral deposits osteoclasts are osteoblasts trapped and surrounded by bone minerals they produce. Osteoclasts remove the mineral and recycle them through the blood stream. B one is not a stable tissue, it is reshaped continuously by bone building osteoblasts and bone degrading osteoclasts. B one appear solid on the surface but are porous structures. They consist of microscopic spaces and canals. The structural unit of bone is osteon which consists of a minute central canal surrounded by osteocytes embedded in a concentric layer of mineral matter. B lood vessels and nerve extensions run through central canal which is connected to spaces cells by fine radiating canals filled with interstitial fluid. The blood vessels supply nutrients to the cell which builds the bone. The nerve cells connect bone cells to the nervous system. Adipose Tissue This connective tissues consists of large densely packed cells called adipocytes. They are specialized for fat storage and has little ECM. It forms a cushion for the body and also acts as an insulating layer under the skin of mammals. Excess carbohydrate are converted to fat stored in adipocytes. These offers weight advantage e.g humans will weigh ~45kg extra if the same chemical energy was stored as CHO instead of fats. Adipose tissue has a rich blood supply of blood vessels which move fats to form adipocytes. B lood It is a connective tissue All it’s cells are suspended in a fluid ECM called plasma. The plasma is a solution of nutrient molecules, ions, and gases. Blood contains two major cell types : erythrocytes (RBC) and leucocytes (WBC). Erythrocytes of mammals are round discs, concave shaped on both sides. They have haemoglobin, a protein that bind and transport oxygen. Their life spans are short e.g about 120 days for human RBC. There are several types of leucocytes : eosinophils, basophils, lymphocytes, monocytes, neutrophils. They all help to protect the body against invading bacteria, viruses and other disease causing agents. Blood plasma contain platelets which are membrane bound fragments of specialized blood cells. They take part in the reactions that seal wounds with blot clots. Blood is the major transport vehicle of the body. It carries oxygen and nutrient to body cells, removes waste and by-products e.g carbon dioxide. Maintains internal fluid environment. Including osmotic balance between cells and interstitial fluid. Blood also transports hormones and other signal molecules. M uscle Tissue Muscle tissue consists of cells with ability to contract. Contraction depends on the interaction of 2 constituents- actin and myosin. It moves body limbs and other structures, pumps blood and squeeze pressure in organs e.g uterus and intestine. There are 3 types of muscle tissue: 1. Skeletal 2. Cardiac 3. Smooth The cells are densely packed with little space for ECM. Skeletal muscle is attached by tendons to skeleton. Elongated and cylindrical in shape, also called muscle fibres. Contain many nuclei, actin, myosin arranged in order giving tissue a band or striated appearance. They lie side by side surrounded by sheets of connective tissue. They contract in response to signals from nerves. The contractions are rapid and help to move body parts and maintain posture. The contractions produce heat which helps in maintaining body temperature in mammals, birds and other vertebrates. Cardiac The contractile tissue of the heart. It has a stripped appearance because it contains actin and myosin molecules arranged as in skeletal muscle. Cardiac muscles are short and branched with each connecting to next cell The joining point between 2 cells is the intercalated disc. Form networks which aid contraction of heart muscle resulting in squeezing or pumping action. The contraction is different from the lengthwise, unidirectional contraction of skeletal muscle. Smooth Present in walls of tubes and body cavities including blood vessels, stomach, intestine, bladder , uterus etc. They are small, spindle shaped cells. The actin and myosin molecules are arranged in a loose network rather than in bundles. Smooth muscle cell are connected by gap junction and enclosed in a mesh of connective tissue. Smooth muscle contracts slowly and for longer periods than skeletal or cardiac muscle. The contractions mix and move stomach and intestinal content, constrict blood vessels and help with parturition. Nervous Tissue Contain nerve cells called neuron. They serve in communication and control between body parts. the brain contains billons of neurons extending through out the body. Nervous tissue also contain glial cells which support and provide nutrients to neurons, provide electrical insulation between them, scavenge cellular debris and foreign matter. A neuron consists of cell body which contains nucleus and organelles with 2 types of cell extension : dendrites and axons. Dendrites receive chemical signals from other neurons or body cells and convert them into electrical signals transmitted to cell body of receiving neuron. Axons convert electrical signal to chemical signals that transmit response to nearby muscle cells, glands cells or other neurons. Axons are usually unbranched except at the terminal All major tissue types: epithelial, connective, nervous, blood and muscle form organs and organ systems. In tissues, organs or organ systems, each cell engages in basic metabolic activities that ensure survival. All vertebrates and most invertebrates have eleven major organ systems: nervous, endocrine, muscular, skeletal, circulatory, lymphatic, respiratory, digestive, excretory, reproductive and integumental. The functions of these organ systems are coordinated and integrated to accomplish a series of activities vital for animals. These include: 1. Acquiring nutrients and other required substances e.g oxygen and distributing them through out the body as well as disposing waste. 2. Synthesizing proteins, CHO, lipids, nucleic acids required for body function. 3. Sensing and responding to changes in the environment e.g temperature, pH, ion conc. 4. Protecting body against injury, attack from other animals and viruses, bacteria. 5. Reproducing, nourishing and protecting offspring Macromolecules of Life Dr Chiaka Anumudu Office B5 [email protected] Chemistry is the logic that underlies biological phenomena Distinctive properties of living systems Organisms are complicated and highly organised Biological structures serve functional purposes Living systems are actively engaged in energy transformations Have a remarkable capacity for self replication Biomolecules: the molecules of life H, O, C and N make up 99+% of the atoms ot the human body. Question: What percentage does each element contribute? Question: What is the rest?? Question: What is the molecular composition of cells? C, H, O and N form strong bonds with each other Covalent bonds are made by sharing a pair of electrons Strongest type of bonds, about 80kcal/mol Fairly stable at room temp Single, c-c, double C=C, triple C=C Typical Covalent Bond Energies Bond Energy kJ/mol H -H 436 C-H 414 C-C 343 C-O 351 Covalent bonds There is free rotation about a single covalent bond,but not about a double or triple bond. Covalent bonds also have a fixed angle. Some covalent bonds involve unequal sharing of electrons. Some atoms hold onto electrons more tightly than other atoms. The tendency to attract electrons is a measure of electronegativity of an atom. Hydrogen bonds attraction betw een a slight positive charge on a hydrogen atom and a slight negative charge on a nearby atom -strength of bond ~ 5 kcal/mol (relatively w eak) -strongest w hen the donor,the hydrogen and the acceptor are about 0.25 nm apart -hydrogen bonds give order and structure to molecules -a single hydrogen bond is w eak,how ever,most molecules are made up of many hydrogen bonds;leads to overall strength of molecule Ionic bonds electrostatic interaction betw een tw o oppositely charged groups in a molecule limiting cause of unequal sharing of electrons;one atom keeps the electron N aCl N a+ + Cl- unequal sharing of electrons,Cl keeps both electrons In solution this group becomes ionized,loses a proton and becomes negatively charged Van der Waal’s interactions nonspecific attractive force that occurs w hen any tw o atoms come in close range -most favourable w hen atoms are 0.2-0.3 nm apart -transient polarity induced betw een atoms a nonpolar bond leads to attraction w ith nearby atoms -very w eak interaction – strength is ~1 kcal/mol. H ow ever the sum of many Van Der W aals interactions leads to increased strength and stability Example:A ligand interacting w ith its receptor is accomplished by many noncovalent interactions such as Van Der W alls interactions. Hydrophobic interactions A molecule forms a particular shape because it likes to adopt the low est energy state (minimize entropy) groups that cannot form hydrogen bonds w ith w ater (the hydrophobic ones)tend to cluster on the inside of the molecule (aw ay from w ater). Hydrophobic: (“w ater hating”)uncharged,nonpolar molecules,don’t interact w ith w ater Hydrophilic: (“w ater loving”):charged or polar molecules;from hydrogen bonds w ith w ater The shape that a biological macromolecule adopts is dependent on a large number of molecular interactions Covalent bonds give a wealth of shapes in 3D And a wealth of functionality DN A C=O and N -H functionalities – H -bond acceptors and donors Covalent bonds make the backbone and the bases (A,C,G ,T) H -bonds dictate the register and direction – A:T – G :C – antiparallel A biomolecular hierarchy Simple molecules are the units for building complex structures Metabolites and macromolecules Organelles Membranes The Cell Properties of biomolecules reflect their fitness for life Chiral monomers make complex biopolymers Back to our weak bonds…. What is the energy (strength) of the weak bonds: hydrogen bond= ionic bond= van der walls = hydrophobic interactions= So weak bonds?? W eak chemical forces mediate biomolecular recognition E.g. Restrict organisms to a narrow range of environmental conditions E.g. Major Macromolecules I The Molecules of Life Within cells, small organic molecules are joined together to form larger molecules Macromolecules are large molecules composed of thousands of covalently connected atoms Most macromolecules are polymers, built from monomers A polymer is a long molecule consisting of many similar building blocks called monomers Three of the four classes of life’s organic molecules are polymers: – Carbohydrates – Proteins – Nucleic acids The Synthesis and Breakdown of Polymers Monomers form larger molecules by condensation reactions called dehydration reactions Polymers are disassembled to monomers by hydrolysis, a reaction that is essentially the reverse of the dehydration reaction Short polymer Unlinked monomer Dehydration removes a water molecule, forming a new bond Longer polymer Dehydration reaction in the synthesis of a polymer Hydrolysis adds a water molecule, breaking a bond Hydrolysis of a polymer The Diversity of Polymers Each cell has thousands of different kinds of macromolecules 1 2 3 H HO Macromolecules vary among cells of an organism, vary more within a species, and vary even more between species An immense variety of polymers can be built from a small set of monomers Carbohydrates serve as fuel and buildingmaterial Carbohydrates include sugars and the polymers of sugars The simplest carbohydrates are monosaccharides, or single sugars Carbohydrate macromolecules are polysaccharides, polymers composed of many sugar building blocks Sugars Monosaccharides have molecular formulas that are usually multiples of CH2O Glucose is the most common monosaccharide Monosaccharides are classified by location of the carbonyl group and by number of carbons in the carbon skeleton Triose sugars Pentose sugars Hexose sugars (C3H6O3) (C5H10O5) (C6H12O6) Aldoses Glyceraldehyde Ribose Glucose Galactose Ketoses Dihydroxyacetone Ribulose Fructose Monosaccharides serve as a major fuel for cells and as raw material for building molecules Though often drawn as a linear skeleton, in aqueous solutions they form rings Linear and Abbreviated ring ring forms structure A disaccharide is formed when a dehydration reaction joins two monosaccharides This covalent bond is called a glycosidic bond Lactose = Glu + Gal Maltose = Glu + Glu Sucrose = Glu + Fru Dehydration reaction in the 1–4 glycosidic synthesis of maltose linkage Glucose Glucose Maltose Dehydration reaction in the 1–2 glycosidic synthesis of sucrose linkage Glucose Fructose Sucrose Polysaccharides Polysaccharides, the polymers of sugars, have storage and structural roles The structure and function of a polysaccharide are determined by its sugar monomers and the positions of glycosidic linkages Starch granules in a potato tuber cell Starch (amylose) Glucose monomer Glycogen granules in muscle tissue Glycogen Cellulose microfibrils in a plant cell wall Cellulose Cellulose Hydrogen bonds molecules between — OH groups (not shown)attached to carbons 3 and 6 Storage Polysaccharides Starch, a storage polysaccharide of plants, consists entirely of glucose monomers Plants store surplus starch as granules within chloroplasts and other plastids Chloroplast Starch 1 µm Amylose Amylopectin Starch:a plant polysaccharide Glycogen is a storage polysaccharide in animals Humans and other vertebrates store glycogen mainly in liver and muscle cells Mitochondria Glycogen granules 0.5 µm Glycogen Glycogen:an animal polysaccharide Structural Polysaccharides Cellulose is a major component of the tough wall of plant cells Like starch, cellulose is a polymer of glucose, but the glycosidic linkages differ The difference is based on two ring forms for glucose: alpha (and beta ( a Glucose b Glucose a and b glucose ring structures Starch:1–4 linkage of a glucose monomers. Cellulose:1–4 linkage of b glucose monomers. Polymers with alpha glucose are helical Polymers with beta glucose are straight In straight structures, H atoms on one strand can bond with OH groups on other strands Parallel cellulose molecules held together this way are grouped into microfibrils, which form strong building materials for plants Cellulose microfibrils in a plant cell wall Cell walls Microfibril 0.5 µm Plant cells Cellulose molecules Glucose monomer Enzymes that digest starch by hydrolyzing alpha linkages can’t hydrolyze beta linkages in cellulose Cellulose in human food passes through the digestive tract as insoluble fiber Some microbes use enzymes to digest cellulose Many herbivores, from cows to termites, have symbiotic relationships with these microbes Chitin, another structural polysaccharide, is found in the exoskeleton of arthropods Chitin also provides structural support for the cell walls of many fungi Chitin can be used as surgical thread Lipids are a diverse group of hydrophobic molecules Lipids are the one class of large biological molecules that do not form polymers The unifying feature of lipids is having little or no affinity for water Lipids are hydrophobic becausethey consist mostly of hydrocarbons, which form nonpolar covalent bonds The most biologically important lipids are fats, phospholipids and steroids Fats Fats are constructed from two types of smaller molecules: glycerol and fatty acids Glycerol is a three-carbon alcohol with a hydroxyl group attached to each carbon A fatty acid consists of a carboxyl group attached to a long carbon skeleton Fatty acid (palmitic acid) Glycerol Dehydration reaction in the synthesis of a fat Fats separate from water because water molecules form hydrogen bonds with each other and exclude the fats In a fat, three fatty acids are joined to glycerol by an ester linkage, creating a triacylglycerol, or triglyceride Ester linkage Fat molecule (triacylglycerol) Fatty acids vary in length (number of carbons)and in the number and locations of double bonds Saturated fatty acids have the maximum number of hydrogen atoms possible and no double bonds Unsaturated fatty acids have one or more double bonds The major function of fats is energy storage (a)Saturated fat (b)Unsaturated fat Structural formula of a saturated fat molecule Structural formula of an unsaturated Space-filling fat molecule model of stearic acid, a saturated fatty acid Space-filling model of oleic acid, an unsaturated fatty acid Double bond causes bending. Fats made from saturated fatty acids are called saturated fats Most animal fats are saturated Saturated fats are solid at room temperature A diet rich in saturated fats may contribute to cardiovascular disease through plaque deposits Stearic acid Saturated fat and fatty acid. Fats made from unsaturated fatty acids are called unsaturated fats Plant fats and fish fats are usually unsaturated Plant fats and fish fats are liquid at room temperature and are called oils Oleic acid cis double bond causes bending Unsaturated fat and fatty acid. Phospholipids In a phospholipid, two fatty acids and a phosphate group are attached to glycerol The two fatty acid tails are hydrophobic, but the phosphate group and its attachments form a hydrophilic head Hydrophilic head Choline Phosphate Glycerol Hydrophobic tails Fatty acids Hydrophilic head Hydrophobic tails (a)Structural formula (b)Space-filling model (c)Phospholipid (d)Phospholipid symbol bilayer When phospholipids are added to water, they self- assemble into a bilayer, with the hydrophobic tails pointing toward the interior The structure of phospholipids results in a bilayer arrangement found in cell membranes Phospholipids are the major component of all cell membranes W ATER Hydrophilic head Hydrophobic tails W ATER Steroids Steroids are lipids characterized by a carbon skeleton consisting of four fused rings Cholesterol, an important steroid, is a component in animal cell membranes Although cholesterol is essential in animals, high levels in the blood may contribute to cardiovascular disease Proteins have many structures, resultingin a wide range of functions Proteins account for more than 50% of the dry mass of most cells Protein functions include structural support, storage, transport, cellular communications, movement, and defense against foreign substances Enzymatic proteins Defensive proteins Function:Selective acceleration of Function:Protection against disease chemical reactions Example:Antibodies inactivate and help Example:Digestive enzymes catalyze the destroy viruses and bacteria. hydrolysis of bonds in food molecules. Antibodies Enzyme Virus Bacterium Storage proteins Transport proteins Function:Storage of amino acids Function:Transport of substances Examples:Casein, the protein of milk, is Examples:Hemoglobin, the iron-containing the major source of amino acids for baby protein of vertebrate blood, transports mammals.Plants have storage proteins oxygen from the lungs to other parts of the in their seeds.Ovalbumin is the protein body.Other proteins transport molecules of egg white, used as an amino acid across cell membranes. source for the developing embryo. Transport protein Ovalbumin Amino acids for embryo Cell membrane Hormonal proteins Receptor proteins Function:Coordination of an organism’ s Function:Response of cell to chemical activities stimuli Example:Insulin, a hormone secreted by Example:Receptors built into the the pancreas, causes other tissues to membrane of a nerve cell detect signaling take up glucose, thus regulating blood molecules released by other nerve cells. sugar concentration. Receptor protein Signaling molecules Insulin High secreted Normal blood sugar blood sugar Structural proteins Contractile and motor proteins Function:Support Function:Movement Examples:Keratin is the protein of hair, Examples:Motor proteins are responsible horns, feathers, and other skin appendages. for the undulations of cilia and flagella. Insects and spiders use silk fibers to make Actin and myosin proteins are their cocoons and webs, respectively. responsible for the contraction of Collagen and elastin proteins provide a muscles. fibrous framework in animal connective tissues. Actin Myosin Collagen Muscle tissue 30 m Connective tissue 60 m Enzymes are a type of protein that acts as a catalyst, speeding up chemical reactions Enzymes can perform their functions repeatedly, functioning as workhorses that carry out the processes of life Substrate (sucrose) Glucose Enzyme (sucrose) Fructose Polypeptides Polypeptides are polymers of amino acids A protein consists of one or more polypeptides Amino Acid Monomers Amino acids are organic molecules with carboxyl and amino groups Amino acids differ in their properties due to differing side chains, called R groups Cells use 20 amino acids to make thousands of proteins carbon Amino Carboxyl group group Nonpolar side chains;hydrophobic Side chain (R group) Glycine Alanine Valine Leucine Isoleucine (Gly or G) (Ala or A) (Val or V) (Leu or L) (le or ) Methionine Phenylalanine Tryptophan Proline (Met or M) (Phe or F) (Trp or W ) (Pro or P) Polar side chains;hydrophilic Serine Threonine Cysteine (Ser or S) (Thr or T) (Cys or C) Tyrosine Asparagine Glutamine (Tyr or Y) (Asn or N) (Gln or Q) Electrically charged side chains;hydrophilic Basic (positively charged) Acidic (negatively charged) Aspartic acid Glutamic acid Lysine Arginine Histidine (Asp or D) (Glu or E) (Lys or K) (Arg or R) (His or H) Learn the 20amino acids, by R group three letter and one letter codes for naming them Amino Acid Polymers Amino acids are linked by peptide bonds A polypeptide is a polymer of amino acids Polypeptides range in length from a few monomers to more than a thousand Each polypeptide has a unique linear sequence of amino acids Each polypeptide has a unique linear sequence of amino acids, with a carboxyl end (C-terminus) and an amino end (N-terminus) NCC-NCC-NCC-NCC Peptide bond New peptide bond forming Side chains Back- bone Amino end Peptide Carboxyl end (N-terminus) bond (C-terminus) Protein Conformation and Function A functional protein consists of one or more polypeptides twisted, folded, and coiled into a unique shape The sequence of amino acids determines a protein’s three-dimensional conformation A protein’s conformation determines its function Ribbon models and space-filling models can depict a protein’s conformation Antibody protein Protein from flu virus SARS-CoV-2Spike Protein (gene S) Schematic 3D structure Mutations Four Levels of Protein Structure The primary structure of a protein is its unique sequence of amino acids Secondary structure, found in most proteins, consists of coils and folds in the polypeptide chain Tertiary structure is determined by interactions among various side chains (R groups) Quaternary structure results when a protein consists of multiple polypeptide chains Diagrammatically….. Secondary Tertiary Quaternary structure structure structure helix pleated sheet Transthyretin Transthyretin polypeptide protein Primary structure, the sequence of amino acids in a protein, is like the order of letters in a long word Primary structure is determined by inherited genetic information Protein sequence can be found on Genbank.org or at UniProtk.db Primary structure Amino acids 1 5 10 Amino end 30 25 20 15 35 40 45 50 Primary structure of transthyretin 55 70 65 60 75 80 85 90 95 115 110 105 100 120 125 Carboxyl end Secondary structure secondary structure – Arrangement of hydrogen bonds – between repeating constituents of the polypeptide backbone Typical secondary structures : – coil alpha helix – folded beta pleated sheet Secondary structure helix Hydrogen bond pleated sheet strand Hydrogen bond Tertiary structure Transthyretin polypeptide Hydrophobic interactions and van der W aals interactions Polypeptide backbone Hydrogen bond Disulfide bridge Ionic bond Quaternary structure Transthyretin protein Quaternary structure results when two or more polypeptide chains form one macromolecule Collagen is a fibrous protein consisting of three polypeptides coiled like a rope Hemoglobin is a globular protein consisting of four polypeptides: two alpha and two beta chains Polypeptide chain Chains Iron Heme Chains Polypeptide chain Collagen Hemoglobin Sickle-Cell Disease: A Simple Change in Primary Structure A slight change in primary structure can affect a protein’s conformation and ability to function Sickle-cell disease, an inherited blood disorder, results from a single amino acid substitution in the protein hemoglobin 10 µm 10 µm Red blood Normal cells are Red blood Fibers of abnormal cell shape full of individual cell shape hemoglobin deform hemoglobin cell into sickle molecules, each shape. carrying oxygen. Figure 3.22 Secondary Primary Quaternary Function Red Blood Cell and Tertiary Structure Structure Shape Structures Normal Molecules do not 1 hemoglobin associate with one 2 another;each carries 3 oxygen. Normal 4 5 subunit 6 7 m Exposed hydro- Sickle-cell Molecules crystallized 1 phobic region hemoglobin into a fiber;capacity to 2 carry oxygen is reduced. Sickle-cell 3 4 5 6 subunit 7 m What Determines Protein Conformation? In addition to primary structure, physical and chemical conditions can affect conformation Alternations in pH, salt concentration, temperature, or other environmental factors can cause a protein to unravel This loss of a protein’s native conformation is called denaturation A denatured protein is biologically inactive Denaturation Normal protein Denatured protein Renaturation The Protein-Folding Problem It is hard to predict a protein’s conformation from its primary structure Most proteins probably go through several states on their way to a stable conformation Chaperonins are protein molecules that assist the proper folding of other proteins Cap Hollow cylinder Chaperonin (fully assembled) Correctly folded Polypeptide protein Steps of Chaperonin The cap attaches, causing The cap comes Action: the cylinder to change off, and the An unfolded poly- shape in such a way that properly folded peptide enters the it creates a hydrophilic protein is released. cylinder from one environment for the end. folding of the polypeptide. Scientists use X-ray crystallography to determine a protein’s conformation Nuclear magnetic resonance (NMR)spectroscopy, which does not require protein crystallization X-ray diffraction pattern Photographic film Diffracted X-rays X-ray source X-ray beam Crystal Nucleic acid Protein X-ray diffraction pattern 3D computer model Experiment Diffracted X-rays X-ray source X-ray beam Crystal Digital detector X-ray diffraction pattern Results RNA DNA RNA polymerase Molecular vsualisation of proteins https://proteopedia.org/wiki/ Search for a protein View the structure, play with it, identify its parts Go and look up the protein on UniProtK.db Explore it Nucleic acids store and transmit hereditary information The amino acid sequence of a polypeptide is programmed by a unit of inheritance called a gene Genes are made of DNA, a nucleic acid RNA controls protein synthesis – tRNA, mRNA, rRNA, non-coding RNAs The Roles of Nucleic Acids There are two types of nucleic acids: – Deoxyribonucleic acid (DNA) – Ribonucleic acid (RNA) DNA provides directions for its own replication DNA directs synthesis of messenger RNA (mRNA) and, through mRNA, controls protein synthesis Protein synthesis occurs in ribosomes DNA Synthesis of mRNA in the nucleus mRNA NUCLEUS CYTOPLASM mRNA Movement of mRNA into cytoplasm via nuclear pore Ribosome Synthesis of protein Amino Polypeptide acids The Structure of Nucleic Acids Nucleic acids are polymers called polynucleotides Each polynucleotide is made of monomers called nucleotides Each nucleotide consists of a nitrogenous base, a pentose sugar and a phosphate group The portion of a nucleotide without the phosphate group is called a nucleoside What is the name of the bond with which 2 nucleotides are joined together? Sugar-phosphate backbone end (on blue background) Nitrogenous bases Pyrimidines C C Nucleoside Nitrogenous Cytosine (C) Thymine Uracil base (T, in DNA) (U, in RNA) Purines Phosphate C group Sugar (pentose) Adenine (A) Guanine (G) C (b)Nucleotide Sugars end (a)Polynucleotide, or nucleic acid Deoxyribose (in DNA) Ribose (in RNA) (c)Nucleoside components 5end Nucleoside Nitrogenous base Phosphate group Pentose sugar Nucleotide 3end Polynucleotide, or nucleic acid Sugar-phosphate backbones Hydrogen bonds Base pair joined by hydrogen bonding Nucleotide Monomers Nucleotide monomers are made up of nucleosides and phosphate groups Nucleoside = nitrogenous base + sugar There are two families of nitrogenous bases: – Pyrimidines have a single six-membered ring – Purines have a six-membered ring fused to a five-membered ring In DNA, the sugar is deoxyribose In RNA, the sugar is ribose Nitrogenous bases Pyrimidines Cytosine Thymine (in DNA)Uracil (in RNA) C T U Purines Adenine Guanine A G Pentose sugars Deoxyribose (in DNA) Ribose (in RNA) Nucleoside components Nucleotide Polymers Nucleotide polymers are linked together, building a polynucleotide Adjacent nucleotides are joined by covalent bonds that form between the –OH group on the 3´carbon of one nucleotide and the phosphate on the 5´carbon on the next These links create a backbone of sugar-phosphate units with nitrogenous bases as appendages The sequence of bases along a DNA or mRNA polymer is unique for each gene The DNA Double Helix A DNA molecule has two polynucleotides spiraling around an imaginary axis, forming a double helix In the DNA double helix, the two backbones run in opposite 5´to 3´directions from each other, an arrangement referred to as antiparallel One DNA molecule includes many genes The nitrogenous bases in DNA form hydrogen bonds in a complementary fashion: A always with T, and G always with C 5end 3end Sugar-phosphate backbone Base pair (joined by What is the hydrogen bonding) implication of the Old strands antiparallel strands Nucleotide for the replication about to be added to a of DNA? new strand 5end New strands 3end 5end 5end 3end DNA and Proteins as Tape Measures of Evolution The linear sequences of nucleotides in DNA molecules are passed from parents to offspring Two closely related species are more similar in DNA than are more distantly related species Molecular biology can be used to assess evolutionary kinship DNA day, Malaria day RNA vs DNA Assignment 1. Go to www.dnaftb.org Using the media files of topics 19-23 -Investigate all the backstory to the discovery of DNA as the genetic material; the people, the context, the sub-plot, the deductions 2.Go to learn.genetics.utah.edu - do the More about RNA -More about DNA and genes: Build a DNA molecule All due Friday before 5pm.The first correct 5 win a prize from me. Animations and Videos Macromolecules – 1 Macromolecules – 2 Polymers Biomolecules – Carbohydrates Carbohydrates Glucose in Water Dehydration and Hydrolysis Animations and Videos Disaccharides Polysaccharides Bozeman – Carbohydrates Fats Lipids Biomolecules – Lipids Bozeman -Lipids Animations and Videos Proteins Protein Structure – 1 Protein Structure – 2 Life Cycle of a Protein Peptide Bond Formation Dehydration Synthesis of Amino Acids Protein Folding -1 Animations and Videos Protein Folding – 2 Protein Organization Protein Denaturation Bozeman – Proteins How Enzymes Work Enzyme Action and the Hydrolysis of Sucrose Enzyme Catalysis -1 Animations and Videos Enzyme Catalysis – 2 Allosteric Regulation of Enzymes Allosteric Enzyme Enzyme Changing Shape Bozeman – Enzymes Bozeman -Nucleic Acids Testing Organic Substances Chapter QuizQuestions – 1 Animations and Videos Chapter QuizQuestions – 2 ZOO 114 Unit 2 Lesson 6 DNA Structure and Function Cracking the Code What is DNA? The genetic material in cells is contained in a molecule called deoxyribonucleic acid, or DNA. Scientists describe DNA as containing a code. A code is a set of rules and symbols used to carry information. To understand how DNA functions, you first need to learn about the structure of the DNA molecule. Copyright © Houghton Mifflin Harcourt Publishing Company Unit 2 Lesson 6 DNA Structure and Function What is the role of DNA and RNA in building proteins? A ribosome is a cell organelle made of ribosomal RNA (rRNA) and protein. As mRNA passes through, transfer RNA (tRNA) delivers amino acids to the ribosomes. The order of the bases codes for which amino acid is attached. The amino acids are joined together to form a protein. Copyright © Houghton Mifflin Harcourt Publishing Company Unit 2 Lesson 6 DNA Structure and Function How was DNA discovered? Many scientists from all over the world contributed to our understanding of DNA. Some scientists discovered the chemicals that make up DNA, and others learned how these chemicals fit together. Still others determined the three-dimensional structure of the DNA molecule. Copyright © Houghton Mifflin Harcourt Publishing Company Unit 2 Lesson 6 DNA Structure and Function Unraveling DNA What does DNA look like? Experiments and imaging techniques have helped scientists to infer the shape of DNA. The structure of DNA is a twisted ladder shape called a double helix. Copyright © Houghton Mifflin Harcourt Publishing Company Unit 2 Lesson 6 DNA Structure and Function What does DNA look like? The two sides of the ladder are made of sugars and phosphate groups. The rungs of the ladder are made of pairs of bases. Copyright © Houghton Mifflin Harcourt Publishing Company Unit 2 Lesson 6 DNA Structure and Function What does DNA look like? A base, a sugar, and a phosphate group make a building block of DNA called a nucleotide. There are four different nucleotides in DNA. Copyright © Houghton Mifflin Harcourt Publishing Company Unit 2 Lesson 6 DNA Structure and Function What does DNA look like? The bases in nucleotides are paired, or complementary. Adenine always pairs with thymine (A-T). Cytosine always pairs with guanine (C-G). The order of the nucleotides in DNA is a code that carries information. Copyright © Houghton Mifflin Harcourt Publishing Company Unit 2 Lesson 6 DNA Structure and Function What does DNA look like? Genes are segments of DNA that relate to a certain trait. The code in the nucleotide order has information about which proteins the cells should build. The types of proteins that your body makes help determine your traits. Copyright © Houghton Mifflin Harcourt Publishing Company Unit 2 Lesson 6 DNA Structure and Function Replication and Mutation How are copies of DNA made? The cell makes copies of DNA molecules through a process known as replication. During replication, the two strands of DNA separate. The bases on each side of the molecule are used as a pattern for a new strand. Copyright © Houghton Mifflin Harcourt Publishing Company Unit 2 Lesson 6 DNA Structure and Function How are copies of DNA made? As bases on the original molecule are exposed, complementary nucleotides are added. When replication is complete, there are two identical DNA molecules. Copyright © Houghton Mifflin Harcourt Publishing Company Unit 2 Lesson 6 DNA Structure and Function How are copies of DNA made? Describe what is happening in the diagram. Copyright © Houghton Mifflin Harcourt Publishing Company Unit 2 Lesson 6 DNA Structure and Function When are copies of DNA made? Before a cell divides, it copies its DNA. Our cells can replicate DNA in just a few hours, because replication begins in many places along a DNA strand. Many groups of proteins are working to replicate your DNA at the same time. Copyright © Houghton Mifflin Harcourt Publishing Company Unit 2 Lesson 6 DNA Structure and Function What are mutations? Mutations are changes in the number, type, or order of bases on a piece of DNA. There are three main kinds of mutations: deletions, insertions, and substitutions. Copyright © Houghton Mifflin Harcourt Publishing Company Unit 2 Lesson 6 DNA Structure and Function What are mutations? In a deletion mutation, a base is left out. In an insertion mutation, an extra base is added. The most common mutation, substitution, happens when one base replaces another. Copyright © Houghton Mifflin Harcourt Publishing Company Unit 2 Lesson 6 DNA Structure and Function What are mutations? Which type of mutation is shown in each row? (The first row is the original sequence.) Copyright © Houghton Mifflin Harcourt Publishing Company Unit 2 Lesson 6 DNA Structure and Function What are mutations? Mutations can happen by random error, and also by damage to the DNA molecule by physical or chemical agents called mutagens. Cells make proteins that can fix errors in DNA, but sometimes the mistake is not corrected. The mistake then becomes part of the genetic code. Copyright © Houghton Mifflin Harcourt Publishing Company Unit 2 Lesson 6 DNA Structure and Function What are mutations? A genetic disorder results from mutations that harm the normal function of the cell. Some genetic disorders are inherited, or passed on from parent to offspring. Other disorders result from mutations during a person’s lifetime. Most cancers fall in this category. Copyright © Houghton Mifflin Harcourt Publishing Company Unit 2 Lesson 6 DNA Structure and Function Protein Factory What is the role of DNA and RNA in building proteins? Some of the information in the DNA is copied to a separate molecule called RNA, or ribonucleic acid. RNA is used to build proteins. Copyright © Houghton Mifflin Harcourt Publishing Company Unit 2 Lesson 6 DNA Structure and Function What is the role of DNA and RNA in building proteins? Like DNA, RNA has a sugar-phosphate backbone and the bases adenine (A), guanine (G), and cytosine (C). Instead of thymine (T), RNA contains uracil (U). Three types of RNA have special roles in making proteins. Copyright © Houghton Mifflin Harcourt Publishing Company Unit 2 Lesson 6 DNA Structure and Function What is the role of DNA and RNA in building proteins? When a cell needs to make a protein, it makes an RNA copy of a section of the DNA. This is called transcription. In transcription, DNA is used as a template to make a complementary strand of messenger RNA (mRNA). The information in the mRNA is then used to build proteins. This is called translation. Copyright © Houghton Mifflin Harcourt Publishing Company Unit 2 Lesson 6 DNA Structure and Function What is the role of DNA and RNA in building proteins? In translation, the mRNA passes through a protein assembly line within a ribosome. Copyright © Houghton Mifflin Harcourt Publishing Company Chromosome & Gene - DNA molecules contain several million nucleotides, while RNA molecules have only a few thousand. - DNA is contained in the chromosomes of the nucleus, each chromosome having a different type of DNA. - Humans have 46 chromosomes (23 pairs), each made up of many genes. - A gene is the portion of the DNA molecule responsible for the synthesis of a single protein (1000 to 2000 nucleotides). Difference between DNA & RNA 1. DNA has four bases: A, G, C, and T. RNA has four bases: A, G, C, and U. 2. In DNA: Sugar is 2-deoxy-D-ribose. In RNA: Sugar is D-ribose. 3. DNA is almost always double-stranded (helical structure). RNA is single strand. 4. RNA is much smaller than DNA. RNA molecules Transmits the genetic information needed to operate the cell. 1. Ribosomal RNA (rRNA) Most abundant RNA – is found in ribosomes: sites for protein synthesis. 2. Messenger RNA (mRNA) Carries genetic information from DNA (in nucleus) to ribosomes (in cytoplasm) for protein synthesis. They are produced in “Transcription” from DNA. 3. Transfer RNA (tRNA) The smallest RNA. Translates the genetic information in mRNA and brings specific Amino acids to the ribosome for protein synthesis. Functions of DNA 1. It reproduces itself when a cell divides (Replication). 2. It supplied the information to make up RNA, proteins, and enzymes. Replication Separation of the two original strands and synthesis of two new daughter strands using the original strands as templates. By breaking H-bonds Replication Replication is bidirectional: takes place at the same speed in both directions. Replication is semiconservative: each daughter molecule has one parental strand and one newly synthesized one. Origin of replication: specific point of DNA where replication begins. Replication fork: specific point of DNA where replication is proceeding. Replication occurs at many places simultaneously along the helix. Replication Leading strand: is synthesized continuously in the 5’ 3’ direction toward the replication fork. Lagging strand: is synthesized discontinuously in the 5’ 3’ direction away from the replication fork. Replication Replisomes: assemblies of “enzyme factories”. Helicases Unwinds the DNA double helix. - Replication of DNA starts with unwinding of the double helix. - Unwinding can occur at either end or in the middle. - Attach themselves to one DNA strand and cause separation of the double helix. Primases Catalyze the synthesis of primers. Primers: are short nucleotides (4 to 15). - They are required to start the synthesis of both daughter strands. - Primases are placed at about every 50 nucleotides in the lagging strand synthesis. DNA Polymerase It catalyzes the formation of the new strands. - It joins the nucleoside triphosphates found in the nucleus. - A new phosphodiester bond is formed between the 5’-phosphate of the nucleoside triphosphate and the 3’-OH group of the new DNA strand. Ligase In formation of lagging strand, small fragments (Okazaki) are join together by ligase enzyme. Protein Synthesis Gene expression: activation of a gene to produce a specific protein. Only a small fraction (1-2%) of the DNA in a chromosome contains genes. Base sequence of the gene carries the information to produce one protein molecule. Change of sequence New protein Gene expression Transcription: synthesis of mRNA (messenger RNA) Translation Reverse transcription Transcription Genetic information is copied from a gene in DNA to make a mRNA. Begins when the section of a DNA that contains the gene to be copied unwinds. Polymerase enzyme identifies a starting point to begin mRNA synthesis. Transcription The DNA splits into two strands: Template strand: it is used to synthesize RNA. Coding Strand (Informational strand): it is not used to synthesize RNA. - Transcription proceeds from the 3’ end to the 5’ end of the template. (informational strand, non-template strand) Direction of transcription - When mRNA is released, the double helix of the DNA re-forms. Transcription C is paired with G, T pairs with A But A pairs with U (not T). Polymerase enzyme moves along the unwound DNA, forming bonds between the bases. RNA Polymerase Section of bases on DNA (template strand): -G–A–A–C–T- Complementary base sequence in mRNA: -C–U–U–G–A- Transcription Sample Problem 22.6 From the template strand of DNA below, write out the mRNA and informational strand of DNA sequences: Template strand: 3’—C T A G G A T A C—5’ mRNA: 5’—G A U C C U A U G—3’ Informational 5’—G A T C C T A T G—3’ strand: Translation mRNA (as a carrier molecule) moves out of the nucleus and goes to ribosomes. tRNA converts the information into amino acids. Amino acids are placed in the proper sequence. Proteins are synthesized. Gene expression Overall function of RAN’s in the cell: facilitate the task of synthesizing protein. Genetic code Genetic code: language that relates the series of nucletides in mRNA to the amino acids specified. The sequence of nucleotides in the mRNA determines the amino acid order for the protein. Every three bases (triplet) along the mRNA makes up a codon. Each codon specifies a particular amino acid. Genetic code Genetic code 64 condons are possible from the triplet combination of A, G, C, and U. Codons are written from the 5’ end to the 3’ end of the mRNA molecule UGA, UAA, and UAG, are stop signals. (code for termination of protein synthesis). AUG has two roles: 1. Signals the start of the proteins synthesis (at the beginning of an mRNA). tRNA (transfer RNA) tRNA translates the codons into specific amino acids. Serine - It contains 70-90 nucleotides. - The 3’ end, called the acceptor stem and always has the nucleotide ACC and a free OH group that binds a specific amino acid. - Anticodon: a sequence of three nucleotides at the bottom of tRNA, which is complementary to three bases in an mRNA and it can identify the needed amino acid. Anticodon loop A G U Codon on mRNA U C A Transcription Translation Protein synthesis mRNA attaches to smaller subunit of a ribosome. tRNA molecules bring specific amino acids to the mRNA. Peptide bonds form between an amino acid and the end of the growing peptide chain. The ribosome moves along mRNA until the end of the codon (translocation). The polypeptide chain is released from the ribosome and becomes an active protein. Sometimes several ribosomes (polysome) translate the same strand of mRNA at the same time to produce several peptide chains. Termination Ribosome encounters a stop condon. No tRNA to complement the termination codon. An enzyme releases the complete polypeptide chain from the ribosome. Amino acids form the three-dimensional structure (active protein). Translation There are 3 stages in translation: 1. Initiation begins with mRNA binding to the ribosome. 2. Elongation proceeds as the next tRNA molecule delivers the next amino acid, and a peptide bond forms between the two amino acids. Translation 3. Termination: Translation continues until a stop codon (UAA, UAG, or UGA) is reached and the completed protein is released. Often the first amino acid (methionine) is not needed and it is removed after protein synthesis is complete. Mutation A heritable change in DNA nucleotide sequence. It changes the sequence of amino acids (structure and function of proteins). Enzyme cannot catalyze. X rays, Overexpose to sun (UV light), Chemicals (mutagens), or Viruses However, some mutations are random events. Cellular Respiration Key Concepts we will cover today... Respiration is the release of energy by combining oxygen with digested food (glucose). Carbon dioxide and water are also produced as byproducts. They are the waste products of respiration. Cellular respiration has two stages. First glucose is broken down to pyruvate during glycolysis, making some ATP. The second stage involving the Krebs cycle is a series of reactions that produce energy-storing molecules during anaerobic respiration. During aerobic respiration, large amounts of ATP are made in an electron transport chain. When oxygen is not present, fermentation follows glycolysis, regenerating NAD+ needed for glycolysis to continue Cellular Energy Most of the foods we eat contain energy stored in proteins, carbohydrates, and fats. Before our cells can use this energy it must be transferred to ATP within the cells. Cells transfer the energy in organic compounds to ATP through a process called cellular respiration. Process cellular respiration Cellular Energy Oxygen in the air you breath makes the production of ATP more efficient. Some ATP is made without this oxygen however. 1. Metabolic processes that require oxygen are called aerobic processes. 2. Metabolic processes that require NO oxygen are called anaerobic processes. Cellular Energy: Respiration The energy-producing process in living things is called respiration. Respiration is the release of energy by combining oxygen with digested food (glucose). Carbon dioxide and water are also produced as byproducts. They are the waste products of respiration. A simple formula to show respiration looks like this: Glucose + oxygen carbon dioxide (waste) + water (waste) + energy Stages of Cellular Respiration Cellular respiration is the process cells use to extract the energy in organic compounds, particularly glucose. Cellular respiration occurs in three major stages Stage 1: glucose is converted to pyruvate producing a small amount of ATP and NADH Stage 2: Aerobic respiration occurs: this is when oxygen is present, pyruvate and NADH make more ATP. Stage 3: In an electron transfer chain, continuing reactions create a large amount of ATP from the materials from stage 2. Stage 1: Breakdown of Glucose The primary fuel for cellular respiration is glucose which is formed when carbohydrates such 1 as starch and sucrose are broken down. In the First stage of cellular respiration, glucose is broken down in the cytoplasm during a process called glycolysis. Glycolysis is an enzyme-assisted anaerobic process that breaks down one six carbon molecule of glucose to two three-carbon pyruvate ions Stage 1: Breakdown of Glucose: Glycolysis Glycolysis can be summarized in 4 steps Step 1: in a series of three reactions, phosphate groups from two ATP molecules are transferred to a glucose molecule Stage 1: Breakdown of Glucose: Glycolysis Glycolysis can be summarized in 4 steps Step 2: In two reactions, the resulting six-carbon molecule is broken down to two three-carbon compounds, each with a phosphate group Stage 1: Breakdown of Glucose: Glycolysis Glycolysis can be summarized in 4 steps Step 3: Two NADH molecules are produced, and one more phosphate group is transferred to each three-carbon compound. Stage 1: Breakdown of Glucose: Glycolysis Glycolysis can be summarized in 4 steps Step 4: In a series of 4 reactions, each three carbon compound is converted to a three-carbon pyruvate, producing 4 ATP molecules in the process Stage 1: Breakdown of Glucose: Glycolysis Summary: In this 4 step process: 1. Glycolysis uses two ATP molecules 2. but produces four ATP molecules in return. 3. Thus, we gain two ATP molecules for a gain ratio of 2 to 1. Glycolysis is followed by another set of reactions that uses the energy temporarily stored in NADH to make more ATP. Stage 2: Production of ATP When oxygen is present, pyruvate produced during glycolysis enters a mitochondrion and is converted to a two-carbon compound. This reaction produces one carbon dioxide molecule, one NADH molecule, and one two- carbon acetyl group The acetyl group is attached to a molecule called coenzyme A forming a compound called acetyl-CoA. Stage 2: Production of ATP Acetyl-CoA enters a series of enzyme-assisted reactions called the Krebs Cycle. The Krebs Cycle has several steps we will be breaking down. Stage 2: Production of ATP Step 1: Acetyl-CoA combines with a 4 carbon compound, forming a six carbon compound and releasing “coenzyme A” Stage 2: Production of ATP Step 2: Carbon Dioxide (CO2) is released from the six- carbon compound, forming a five-carbon compound. Electrons are transferred to NAD+, making a molecule of NADH. Stage 2: Production of ATP Step 3: Carbon dioxide is released from the five-carbon compound, resulting in a four-carbon compound. A molecule of ATP is made, and a molecule of NADH is produced. Stage 2: Production of ATP Step 4: The existing four-carbon compound is then converted to a new four- carbon compound. Electrons are transferred to an electron acceptor called FAD, making a molecule of FADH2. FADH2 is another type of electron carrier. Stage 2: Production of ATP After the Krebs Cycle, NADH and FADH2 now contain much of the energy that was previously stored in glucose and pyruvate. When the Krebs Cycle is completed, the four-carbon compound that began the cycle has been recycled, and acetyl-CoA can enter the cycle again. Electron Transfer Chain In aerobic respiration, electrons donated by NADH and FADH2 pass through an electron transport chain. Step 1: The electron transfer chain pumps hydrogen ions out of the inner compartment Electron Transfer Chain Step 2: At the end of the chain, electrons and hydrogen ions combine with oxygen, forming water (H2O). Electron Transfer Chain Step 3: ATP is produced as hydrogen ions diffuse into the inner compartment through a channel protein. Electron Transfer Chain Hydrogen ions diffuse back into the inner compartment through a carrier protein that adds a phosphate group to ADP, making ATP. At the end of the electron chain, hydrogen ions and spent electrons combine with oxygen molecules (O2) forming water molecules (H20) Respiration in the Absence of Oxygen What happens when there is not enough oxygen for aerobic respiration to occur? When oxygen is not present, NAD+ is recycled in another way Under anaerobic conditions, electrons carried by NADH are transferred to pyruvate produced during glycolysis. This process recycles NAD+ needed to continue making ATP through glycolysis. This recycling of NAD+ using an organic hydrogen acceptor is called fermentation. Respiration in the Absence of Oxygen Two important types of fermentation are lactic acid fermentation and alcoholic fermentation. Lactic acid fermentation by some prokaryotes and fungi is used in the production of foods such as yogurt and some cheeses. Lactic Acid Fermentation Lactate is the ion of an organic acid called lactic acid. For example, during exercise, pyruvate in muscles is converted to lactate when muscles must operate without enough oxygen. Fermentation enables glycolysis to continue producing ATP in muscles as long as the glucose supply lasts. Blood removes excess lactate from muscles. Lactate can build up in muscle cells if it is not removed quickly enough, sometimes causing muscle soreness. Alcoholic Fermentation Alcoholic fermentation is a two- step process. First, pyruvate is converted to a two-carbon compound, releasing carbon dioxide. Second, electrons are transferred from a molecule of NADH to the two-carbon compound, producing ethanol. Alcoholic Fermentation Alcoholic fermentation by yeast, a fungus, has been used in the preparation of many foods and beverages. Wine and beer contain ethanol made during alcoholic fermentation by yeast. Carbon dioxide released by the yeast causes the rising of bread dough and the carbonation of some alcoholic beverages, such as beer. Ethanol is actually toxic to yeast. At a concentration of about 12%, ethanol kills yeast. Thus, naturally fermented wine contains about 12% ethanol. Production of ATP: Summary The total amount of ATP that a cell is able to harvest from each glucose molecule that enters glycolysis depends on the presence or absence of oxygen. Cells use energy most efficiently when oxygen is present. In the first stage of cellular respiration, glucose is broken down to pyruvate during glycolysis. Glycolysis is an anaerobic process (no oxygen required), and it results in a gain of two ATP molecules. In the second stage of cellular respiration, the pyruvate passes through either aerobic respiration (requires oxygen) or fermentation. When oxygen is not present, fermentation occurs instead. Production of ATP: Summary Most of a cell’s ATP is made during aerobic respiration. (requiring oxygen) For each molecule of glucose that is broken down, as many as two ATP molecules are made directly during the Krebs cycle, and up to 34 ATP molecules are produced later by the electron transport chain. Key Concepts: Review... Cellular respiration has two stages. First glucose is broken down to pyruvate during glycolysis, making some ATP. The Krebs cycle is a series of reactions that produce energy- storing molecules during anaerobic respiration During aerobic respiration, large amounts of ATP are made in an electron transport chain. When oxygen is not present, fermentation follows glycolysis, regenerating NAD+ needed for glycolysis to continue What is embryology? The study of developmental events that occur during the prenatal stage. The branch of biology and medicine concerned with the study of embryos and their development. Embryonic period vs. Fetal period Embryonic – first 8 weeks Development of the three primary germ layers give rise to all structures and Basic body plan takes shape Fetal period – remaining 30 weeks. Structures and organs continue to grow and develop. 8 weeks Developmental History of a Human Stages of Development 1. Fertilization 2. Cleavage 3. Gastrulation Embryogenesis 4.Organogenesis 5.Maturation Fertilization The process of fusion or union of the spermatozoon with the mature ovum is known as conception / fertilizaiton/impregnantation. Which produced the fertilized single mono- nucleated cell called the zygote. Embryogenesis: The formation and development of an embryo. 1. Cleavage is a series of rapid mitotic divisions (without cell growth) 2. Gastrulation : is a phase early in the embryonic development of most animals/human being, during which the single-layered blastula is reorganized into a trilaminar ("three-layered") structure known as the gastrula. These three germ layers are known as the ectoderm, mesoderm, and endoderm. 3. Organogenesis: The production and development of the organs of an animal or plant. How fertilization occurs???? Following ovulation, the ovum, which is about 0.15 mm in diameter is picked up by the tubal fimbriae and is moved along by the cilia and by peristaltic movement of the tube. At the time the cervix under the influence of estrogen, secretes a flow of alkaline mucus that deposited in the vagina, only thousands capacitated spermatozoa enter the uterine tube while 300-500 reach the ovum, and remainder are destroyed by the acid medium of the vagina. It takes about 1 hour for sperm to reach the site. The sperm release the enzyme, Hylluronidase which allows penetration of the zona pellucida and the cell membrane surrounding the ovum. Many sperm are needed for this to take place but only one will enter the ovum. After this the membrane is sealed to prevent entry of any further sperm and the nucluei of the two cell fuse. The sperm and ovum contribute half the complement of chromosomes to make a total of 46. The sperm and ovum is known as the male and female gametes and the fertilized ovum as the zygote. Fertilization Normal site for Conception The most common site of conception is the ampullary part (Ampulla ) of the fallopian tube which is the widest part located closed to the ovary Usual Time for Conception Neither sperm nor ovum can survive longer than 2-3 days and the fertilization is most likely to occur when intercourse takes place not more than 48 hours before and 24 hours after ovulation. So the conception will take place about 14 days before the next period is due. The sex of the new individual at the time of conception is determined by sex chromosomes. Every human cell contains 46 chromosomes, which are made up of 44 autosome chromosome and 2 sex chromosomes. The sex chromosome are X and Y. Woman have no Y chromosome and male has Y chromosome (male 44+X+Y) (female 44+X+X). Therefor e, sex of child is always determined by father Morula 1. After fertilization, the Zygote divides into 2 cells (blastomere) (mitosis division)in about 30 hours after fertilization. 2. The blastomeres continue to divide by binary division through 4, 8, and 16 cell stage until a cluster of cells is formed– Morula, resemblibg a mulberry The morula after spending about 3 days(72 hours) in the uterine tube enters the uterine cavity through the narrow uterine ostium (1mm) on the 4th day. Morula Morula Morula 72 hours post- fertilization entering uterus 4. Blastocyst Morula, once entering the uterine cavity, floats freely(next 2 days) and is covered by endometrial fluid and mucus. This fluid is absorbed through the canaliculi of the zona pellucida and Morula begins to accumulate fluid and forms a cavity between its cells. Once cavity appears, it is now called a blastocyst. Fertilization and the Events of the First 6 Days of Development Blastocyst The zona pellucida becomes stretched, thinned and gradually disappear soon prior to implantation. The cell of the outer cell mass forms the wall of the blastocyst and is known as trophoblast. The inner cell mass is concerned with the development of the embryo. Two Distinct Cell Types 1. Trophoblasts – will form the invading placenta 2. Inner cell mass – will form the embryo Trophoblasts Blastocyst Trophoblast Inner cell mass Placenta Chorion Fetus Amnion umbilical cord hCG is produced hCG is human chorionic gonadotropin hormone It is produced by the trophoblasts starting on day 6 hCG causes endometrium of uterus to grow and proliferate hCG prevents the menstrual cycle from occuring This is why a female misses her periods when she is pregnant Implantation (Week 2) Implantation occurs about 6th day after fertilization (approx the 20th day of a regular menstrual cycle). As the zona pellucida surrounding the blastocyst disappear, there is increased adhesiveness of the trophoblastic cells to the endometrium. The trophoblastic cells invade the stromal cells lying between the secretary endometrial glands by histolytic action of the blastocyst bringing about deeper penetration in the decidua. The syncytial cells ( multinucleate cell) ultimately penetrate the endothelial coat of the maternal decidual capillaries establishing communication with the syncytial lacunar system Thus maternal blood circulates through the syncytial lacunae providing nourishment to the blastocyst. Maternal blood vassels Further penetration is arrested by the maternal immunological blocking factor. The point of entry of the blastocyst into the decidual is sealed by a fibrin coat and later by epithelium The process of implantation is completed by the 10th or 11th day of fertilization. This type of deeper penetration of the human blastocyst is called interstitial implantation and the blastocyst is covered on all sides by the endometrium (decidual). Occasionally, there may be increased blood flow into the lacunar space and cause bleeding. It is called implantation bleeding and This corresponds approx to 13th day after fertilization (at about the expected day of period). This may produce confusion in determination of the EDD The outer cell mass of the blastocyst form the trophoblast layer. The inner cell mass on the 8th day differentiates into bilaminar germ disc consisting of a dorsal ectodermal layer of the tall columnar cells and a ventral endodermal layer of flat polyhydral cells. The bilaminar germ disc is connected to the trophoblast by a mesenchymal connecting stalk called the body stalk which later forms the umblical cord. Formation of Amniotic cavity and Yolk sac Two cavities appear on either side of the bilaminar embryonic disc. The dorsal cavity between the ectoderm and trophoblastic layers lined by mesenchyme(connective tissue) is called the amniotic cavity. The cavity on the ventral aspect lined by the primitive mesenchyme on the outside and the endodermal layer of the germ cell disc on the inside is called the yolk sac Week 2 Inner cell mass divides into epiblast and hypoblast 2 fluid filled sacs Amniotic sac from epiblast within which the embryo and later the fetus develop until birth Yolk sac from hypoblast which is one of the structures through which the mother supplies nutrients to the early embryo Bil