CH 4 PDF.pdf
Document Details
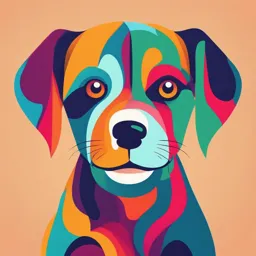
Uploaded by UltraCrispIntellect1022
2019
Tags
Full Transcript
SOME QUESTIONS WE WILL CONSIDER ◗ Is it possible to focus attention on just one thing, even when there are many other things going on at the same time? (95) ◗ Under what conditions can we pay attention to more than one thing at a time? (110) ◗ What does attention research tell us about the effect of...
SOME QUESTIONS WE WILL CONSIDER ◗ Is it possible to focus attention on just one thing, even when there are many other things going on at the same time? (95) ◗ Under what conditions can we pay attention to more than one thing at a time? (110) ◗ What does attention research tell us about the effect of talking on cell phones while driving a car? (112) ◗ Is it true that we are not paying attention to a large fraction of the things happening in our environment? (115) R oger, sitting in the library, is attempting to do his math homework when some people at the next table start talking. He is annoyed because people aren’t supposed to talk in the library, but he is so focused on the math problems that it doesn’t distract him (Figure 4.1a). However, a little later, when he decides to take a break from his math homework and play an easy game on his cell phone, he does find their conversation distracting (Figure 4.1b). “Interesting,” he thinks. “Their talking didn’t bother me when I was doing the math problems.” Deciding to stop resisting his listening to the conversation, Roger begins to consciously eavesdrop while continuing to play his cell phone game (Figure 4.1c). But just as he is beginning to figure out what the couple is talking about, his attention is captured by a loud noise and commotion from across the room, where it appears a book cart has overturned, scattering books on the floor. As he notices that one person seems upset and others are gathering up the books, he looks from one person to another and decides he doesn’t know any of them (Figure 4.1d). ll blah,blah blah,blah Doing math problems, not distracted (a) Selective attention Playing game, distracted (b) Distraction blah,blah Playing game and listening (c) Divided attention 94 Commotion across room (d) Attentional capture and visual scanning ➤ Figure 4.1 Roger’s adventures with attention. (a) Selective attention: doing math problems while not being distracted by people talking. (b) Distraction: playing a game but being distracted by the people talking. (c) Divided attention: playing the game while listening in on the conversation. (d) Attentional capture and scanning: a noise attracts his attention, and he scans the scene to figure out what is happening. Copyright 2019 Cengage Learning. All Rights Reserved. May not be copied, scanned, or duplicated, in whole or in part. WCN 02-200-202 08271_ch04_ptg01.indd 94 4/18/18 4:20 PM Attention as Information Processing 95 Roger’s experiences illustrate different aspects of attention—the ability to focus on specific stimuli or locations. His attempt to focus on his math homework while ignoring the people talking is an example of selective attention—attending to one thing while ignoring others. The way the conversation in the library interfered with his cell phone game is an example of distraction—one stimulus interfering with the processing of another stimulus. When Roger decides to listen in on the conversation while simultaneously playing the game, he is displaying divided attention—paying attention to more than one thing at a time. Later, his eavesdropping is interrupted by the noise of the overturned book cart, an example of attentional capture—a rapid shifting of attention usually caused by a stimulus such as a loud noise, bright light, or sudden movement. Finally, Roger’s attempt to identify the people across the room, looking from one person’s face to another, is an example of visual scanning—movements of the eyes from one location or object to another. With all of these different aspects of attention in mind, let’s return to William James’s (1890) definition of attention, which we introduced in Chapter 1: Millions of items . . . are present to my senses which never properly enter my experience. Why? Because they have no interest for me. My experience is what I agree to attend to. . . . Everyone knows what attention is. It is the taking possession by the mind, in clear and vivid form, of one out of what seem several simultaneously possible objects or trains of thought. . . . It implies withdrawal from some things in order to deal effectively with others. Although this definition is considered a classic, and certainly does capture a central characteristic of attention—withdrawal from some things in order to deal effectively with others—we can now see that it doesn’t capture the diversity of phenomena that are associated with attention. Attention, as it turns out, is not one thing. There are many different aspects of attention, which have been studied using different approaches. This chapter, therefore, consists of a number of sections, each of which is about a different aspect of attention. We begin with a little history, because early research on attention helped establish the information processing approach to cognition, which became the central focus of the new field of cognitive psychology. Attention as Information Processing Modern research on attention began in the 1950s with the introduction of Broadbent’s filter model of attention. Broadbent’s Filter Model of Attention Broadbent’s filter model of attention, which we introduced in Chapter 1 (page 14), was designed to explain the results of an experiment done by Colin Cherry (1953). Cherry studied attention using a technique called dichotic listening, where dichotic refers to presenting different stimuli to the left and right ears. The participant’s task in this experiment is to focus on the message in one ear, called the attended ear, and to repeat what he or she is hearing out loud. This procedure of repeating the words as they are heard is called shadowing (Figure 4.2). Cherry found that although his participants could easily shadow a spoken message presented to the attended ear, and they could report whether the unattended message was spoken by a male or female, they couldn’t report what was being said in the unattended ear. Other dichotic listening experiments confirmed that people are not aware of most of the information being presented to the unattended ear. For example, Neville Moray (1959) showed that participants were unaware of a word that had The meaning of life is... The yellow dog chased... The yellow dog chased... ➤ Figure 4.2 In the shadowing procedure, which involves dichotic listening, a person repeats out loud the words that they have just heard. This ensures that participants are focusing their attention on the attended message. Copyright 2019 Cengage Learning. All Rights Reserved. May not be copied, scanned, or duplicated, in whole or in part. WCN 02-200-202 08271_ch04_ptg01.indd 95 4/18/18 4:20 PM 96 CHAPTER 4 Attention Messages Sensory memory Filter Detector To memory Attended message ➤ Figure 4.3 Flow diagram of Broadbent’s filter model of attention. been repeated 35 times in the unattended ear. The ability to focus on one stimulus while filtering out other stimuli has been called the cocktail party effect, because at noisy parties people are able to focus on what one person is saying even if there are many conversations happening at the same time. Based on results such as these, Donald Broadbent (1958) created a model of attention designed to explain how it is possible to focus on one message and why information isn’t taken in from the other message. This model, which introduced the flow diagram to cognitive psychology (see page 14), proposed that information passes through the following stages (Figure 4.3): Sensory memory holds all of the incoming information for a fraction of a second and then transfers all of it to the filter. (We will discuss sensory memory in Chapter 5.) ➤ The filter identifies the message that is being attended to based on its physical characteristics—things like the speaker’s tone of voice, pitch, speed of talking, and accent—and lets only this attended message pass through to the detector in the next stage. All of the other messages are filtered out. ➤ The detector processes the information from the attended message to determine higher-level characteristics of the message, such as its meaning. Because only the important, attended information has been let through the filter, the detector processes all of the information that enters it. ➤ The output of the detector is sent to short-term memory, which holds information for 10–15 seconds and also transfers information into long-term memory, which can hold information indefinitely. We will describe short- and long-term memory in Chapters 5–8. ➤ Broadbent’s model is called an early selection model because the filter eliminates the unattended information right at the beginning of the flow of information. Modifying Broadbent’s Model: More Early Selection Models The beauty of Broadbent’s filter model of attention was that it provided testable predictions about selective attention, which stimulated further research. One prediction is that since all of the unattended messages are filtered out, we should not be conscious of information in the unattended messages. To test this idea, Neville Moray (1959) did a dichotic listening experiment in which his participants were instructed to shadow the message presented to one ear and to ignore the message presented to the other ear. But when Moray presented the listener’s name to the unattended ear, about a third of the participants detected it (also see Wood & Cowan, 1995). Moray’s participants had recognized their names even though, according to Broadbent’s theory, the filter is supposed to let through only one message, based on its physical characteristics. Clearly, the person’s name had not been filtered out, and, most important, it had been analyzed enough to determine its meaning. You may have had an experience Copyright 2019 Cengage Learning. All Rights Reserved. May not be copied, scanned, or duplicated, in whole or in part. WCN 02-200-202 08271_ch04_ptg01.indd 96 4/18/18 4:20 PM Attention as Information Processing 97 similar to Moray’s laboratory demonstration if, as you were talking to someone in a noisy room, you suddenly heard someone else say your name. Following Moray’s lead, other experimenters showed that information pre9 Dear sented to the unattended ear is processed enough to provide the listener with Left ear some awareness of its meaning. For example, J. A. Gray and A. I. Wedderburn (1960), while undergraduates at the University of Oxford, did the following experiment, sometimes called the “Dear Aunt Jane” experiment. As in Cherry’s dichotic listening experiment, the participants were told to shadow the message presented to one ear. As you can see in Figure 4.4, the attended (shadowed) ear received the message “Dear 7 Jane,” and the unattended ear received the message “9 Aunt 6.” However, rather than reporting the “Dear 7 Jane” message that was presented to the attended ear, participants reported hearing “Dear Aunt Jane.” Aunt 7 Switching to the unattended channel to say “Aunt” means that the participant’s attention had jumped from one ear to the other and then back again. This occurred because they were taking the meaning of the words into account. (An example of top-down processing! See page 67.) Because of results such as these, Anne Treisman (1964) proposed a modification of Broadbent’s model. Treisman proposed that selection occurs in two stages, and she replaced Broadbent’s filter with an attenuator (Figure 4.5). The attenuator analyzes the incoming message in terms of (1) its physical characteristics— whether it is high-pitched or low-pitched, fast or slow; (2) its language—how the message groups into syllables or words; and (3) its meaning—how sequences 6 Jane of words create meaningful phrases. Note that the attenuator represents a process and is not identified with a specific brain structure. Treisman’s idea that the information in the channel is selected is similar to what Broadbent proposed, but in Treisman’s attenuation model of attention, language and meaning can also be used to separate the messages. However, Treisman proposed that the analysis of the message proceeds only as far as is necessary to identify the attended message. For example, if there are two messages, one in a male voice and one in a female voice, then analysis at the physical level (which Instructions: Broadbent emphasized) is adequate to separate the low-pitched male voice from Shadow this side the higher-pitched female voice. If, however, the voices are similar, then it might be necessary to use meaning to separate the two messages. ➤ Figure 4.4 In Gray and Wedderburn’s (1960) “Dear Aunt Jane” experiment, participants were According to Treisman’s model, once the attended and unattended mestold to shadow the message presented to the left sages have been identified, both messages pass through the attenuator, but the ear. But they reported hearing the message “Dear attended message emerges at full strength and the unattended messages are Aunt Jane,” which starts in the left ear, jumps to attenuated—they are still present but are weaker than the attended message. the right ear, and then goes back to the left ear. Because at least some of the unattended message gets through the attenuator, Unless otherwise noted all items © Cengage Treisman’s model has been called a “leaky filter” model. The final output of the system is determined in the second stage, when the message is analyzed by the dictionary unit. The dictionary unit conAttended message tains words, stored in memory, each of which has a threshold for being activated (Figure 4.6). A threshDictionary old is the smallest signal strength that can barely be Attenuator To memory Messages unit detected. Thus, a word with a low threshold might be detected even when it is presented softly or is obscured by other words. Unattended According to Treisman, words that are commessages mon or especially important, such as the listener’s name, have low thresholds, so even a weak signal in ➤ Figure 4.5 Flow diagram for Treisman’s attenuation model of selective the unattended channel can activate that word, and attention. Copyright 2019 Cengage Learning. All Rights Reserved. May not be copied, scanned, or duplicated, in whole or in part. WCN 02-200-202 08271_ch04_ptg01.indd 97 4/18/18 4:20 PM 98 CHAPTER 4 Attention Signal strength needed to activate High Low Own name Rutabaga Boat ➤ Figure 4.6 The dictionary unit of Treisman’s attenuation model of selective attention contains words, each of which has a threshold for being detected. This graph shows the thresholds that might exist for three words. The person’s name has a low threshold, so it will be easily detected. The thresholds for the words rutabaga and boat are higher, because they are used less or are less important to this particular listener. we hear our name from across the room. Uncommon words or words that are unimportant to the listener have higher thresholds, so it takes the strong signal of the attended message to activate these words. Thus, according to Treisman, the attended message gets through, plus some parts of the weaker, unattended messages. The research we have been describing so far was extremely important, not only because it defined some of the basic phenomena of attention but also because it demonstrated how an aspect of cognition could be conceptualized as a problem of information processing, in which information from the environment passes through various stages of processing. Like Broadbent’s model, Treisman’s is called an early selection model because it proposes a filter that operates at an early stage in the flow of information. Other models propose that selection can occur later. A Late Selection Model Other theories were proposed to take into account the results of experiments showing that messages can be selected at a later stage of processing, based primarily on their meaning. For example, in an experiment by Donald MacKay (1973), a participant listened to an ambiguous sentence, such as “They were throwing stones at the bank,” that could be interpreted in more than one way. (In this example, “bank” can refer to a riverbank or to a financial institution.) These ambiguous sentences were presented to the attended ear while biasing words were presented to the other, unattended ear. For example, as the participant was shadowing “They were throwing stones at the bank,” either the word “river” or the word “money” was presented to the unattended ear. After hearing a number of ambiguous sentences, the participants were presented with pairs of sentences, such as “They threw stones toward the side of the river yesterday” and “They threw stones at the savings and loan association yesterday,” and asked to indicate which of these two sentences was closest in meaning to one of the sentences they had heard previously. MacKay found that the meaning of the biasing word affected the participants’ choice. For example, if the biasing word was “money,” participants were more likely to pick the second sentence. This occurred even though participants reported that they were unaware of the biasing words that had been presented to the unattended ear. MacKay proposed that because the meaning of the word river or money was affecting the participants’ judgments, the word must have been processed to the level of meaning even though it was unattended. Results such as this led MacKay and other theorists to develop late selection models of attention, which proposed that most of the incoming information is processed to the level of meaning before the message to be further processed is selected (Deutsch & Deutsch, 1963; Norman, 1968). The attention research we have been describing has focused on when selective attention occurs (early or late) and what types of information are used for the selection (physical characteristics or meaning). But as research in selective attention progressed, researchers realized that there is no one answer to what has been called the “early–late” controversy. Early selection can be demonstrated under some conditions and later selection under others, depending on the observer’s task and the type of stimuli presented. Thus, researchers began focusing instead on understanding the many different factors that control attention. This brings us back to Roger’s experience in the library. Remember that he was able to ignore the people talking when he was doing his math homework but became distracted by the talking when he was playing the easy cell phone game. The idea that the ability to selectively attend to a task can depend both on the distracting stimulus and on the nature of the task has been studied by Nilli Lavie (2010), who introduced the concepts of processing capacity and perceptual load. Copyright 2019 Cengage Learning. All Rights Reserved. May not be copied, scanned, or duplicated, in whole or in part. WCN 02-200-202 08271_ch04_ptg01.indd 98 4/18/18 4:20 PM Processing Capacity and Perceptual Load 99 Processing Capacity and Perceptual Load How do people ignore distracting stimuli when they are trying to focus their attention on a task? Lavie answers this question by considering two factors: (1) processing capacity, which refers to the amount of information people can handle and sets a limit on their ability to process incoming information; and (2) perceptual load, which is related to the difficulty of a task. Some tasks, especially easy, well-practiced ones, have low perceptual loads; these low-load tasks use up only a small amount of the person’s processing capacity. Tasks that are difficult and perhaps not as well practiced are high-load tasks and use more of a person’s processing capacity. Sophie Forster and Lavie (2008) studied the role of processing capacity and perceptual load in determining distraction by presenting displays like the one in Figure 4.7a. The participants’ task was to respond as quickly as possible when they identified a target, either X or N. Participants pressed one key if they saw the X and another key if they saw the N. This task is easy for displays like the one on the left in Figure 4.7a, in which the target is surrounded by just one type of letter, like the small o’s. However, the task becomes harder when the target is surrounded by different letters, as in the display on the right. This difference is reflected in the reaction times, with the hard task resulting in longer reaction times than the easy task. However, when a task-irrelevant stimulus—like the unrelated cartoon character shown in Figure 4.7b— is flashed below the display, responding slows for the easy task more than for the hard task. Lavie explains results such as the ones in Figure 4.7b in terms of her load theory of attention, diagrammed in Figure 4.8, in which the circle represents the person’s processing capacity and the shading represents the portion that is used up by a task. Figure 4.8a shows that with the low-load task, there is still processing capacity left. This means that resources are available to process the task-irrelevant stimulus (like the cartoon character), and even o N o o H Z W X 800 800 700 700 RT (msec) RT (msec) o M 600 500 400 N o M Z o o H X o W 600 500 400 0 0 Easy (a) K o K o Hard Easy Hard (b) ➤ Figure 4.7 The task in Forster and Lavie’s (2008) experiment was to indicate the identity of a target (X or N) as quickly as possible in displays like the ones shown here. (a) The reaction time for the easy condition like the display on the left, in which the target is accompanied by small o’s, is faster than the reaction time for the hard condition, in which the target is accompanied by other letters. (b) Flashing a distracting cartoon character near the display increases the reaction time for the easy task more than it does for the hard task. The increase for each task is indicated by the gray extensions of the bars. (Source: Adapted from S. Forster & N. Lavie, Failures to ignore entirely irrelevant distractors: The role of load, Journal of Experimental Psychology: Applied, 14 , 73–83, 2008.) Copyright 2019 Cengage Learning. All Rights Reserved. May not be copied, scanned, or duplicated, in whole or in part. WCN 02-200-202 08271_ch04_ptg01.indd 99 4/18/18 4:20 PM 100 CHAPTER 4 Attention Remaining perceptual capacity No perceptual capacity remains Resources used by low-load primary task Resources used by high-load primary task (a) (b) ➤ Figure 4.8 The load theory of attention: (a) Low-load tasks that use few cognitive resources may leave resources available for processing unattended task-irrelevant stimuli, whereas (b) high-load tasks that use all of a person’s cognitive resources don’t leave any resources to process unattended taskirrelevant stimuli. though the person was told not to pay attention to the task-irrelevant stimulus, it gets processed and slows down responding. Figure 4.8b shows a situation in which all of a person’s processing capacity is being used for a high-load task, such as the hard task in the experiment. When this occurs, no resources remain to process other stimuli, so irrelevant stimuli can’t be processed and they have little effect on performance of the task. Thus, if you are carrying out a hard, high-load task, no processing capacity remains, and you are less likely to be distracted (as Roger found when he was focusing on the hard math problems). However, if you are carrying out an easy, low-load task, the processing capacity that remains is available to process task-irrelevant stimuli (as Roger found out when he was distracted from his easy cell phone game). The ability to ignore task-irrelevant stimuli is a function not only of the load of the task you are trying to do but also of how powerful the task-irrelevant stimulus is. For example, while Roger was able to ignore the conversation in the library while he was focused on the difficult math problems, a loud siren, indicating fire, would probably attract his attention. An example of a situation in which task-irrelevant stimuli are difficult to ignore is provided by the Stroop effect, described in the following demonstration. D E M O N S T R AT I O N The Stroop Effect Look at Figure 4.9. Your task is to name, as quickly as possible, the color of ink used to print each of the shapes. For example, starting in the upper-left corner and going across, you would say, “red, blue, . . .” and so on. Time yourself (or a friend you have enlisted to do this task), and determine how many seconds it takes to report the colors of all the shapes. Then repeat the same task for Figure 4.10, remembering that your task is to specify the color of the ink, not the color name that is spelled out. ➤ Figure 4.9 Name the color of the ink used to print these shapes. Copyright 2019 Cengage Learning. All Rights Reserved. May not be copied, scanned, or duplicated, in whole or in part. WCN 02-200-202 08271_ch04_ptg01.indd 100 4/18/18 4:20 PM Processing Capacity and Perceptual Load YELLOW RED BLUE PURPLE GREEN ORANGE YELLOW GREEN BLUE RED GREEN PURPLE ORANGE RED BLUE 101 ➤ Figure 4.10 Name the color of the ink used to print these words. If you found it harder to name the colors of the words than the colors of the shapes, then you were experiencing the Stroop effect, which was first described by J. R. Stroop in 1935. This effect occurs because the names of the words cause a competing response and therefore slow responding to the target—the color of the ink. In the Stroop effect, the task-irrelevant stimuli are extremely powerful, because reading words is highly practiced and has become so automatic that it is difficult not to read them (Stroop, 1935). The approaches to attention we have described so far—early information processing models and Lavie’s load approach—are concerned with the ability to focus attention on a particular image or task. But in everyday experience you often shift your attention from place to place, either by moving your eyes or by shifting attention “in your mind” without moving your eyes. We discuss such shifts in attention next. T E ST YOUR SELF 4.1 1. Give examples of situations that illustrate the following: selective attention, distraction, divided attention, attentional capture, and scanning. 2. How was the dichotic listening procedure used to determine how well people can focus on the attended message and how much information can be taken in from the unattended message? What is the cocktail party effect, and what does it demonstrate? 3. Describe Broadbent’s model of selective attention. Why is it called an early selection model? 4. What were the results of experiments by Moray (words in the unattended ear) and Gray and Wedderburn (“Dear Aunt Jane”)? Why are the results of these experiments difficult to explain based on Broadbent’s filter model of attention? 5. Describe Treisman’s attenuation model. First indicate why she proposed the theory, then how she modified Broadbent’s model to explain some results that Broadbent’s model couldn’t explain. 6. Describe MacKay’s “bank” experiment. Why does his result provide evidence for late selection? 7. Describe the Forster and Lavie experiment on how processing capacity and perceptual load determine distraction. What is the load theory of attention? 8. What is the Stroop effect? What does it illustrate about task-irrelevant stimuli? Copyright 2019 Cengage Learning. All Rights Reserved. May not be copied, scanned, or duplicated, in whole or in part. WCN 02-200-202 08271_ch04_ptg01.indd 101 4/18/18 4:20 PM 102 CHAPTER 4 Attention Directing Attention by Scanning a Scene Attention, according William James, involves “withdrawing from some things in order to effectively deal with others.” Think about what this means when applied to everyday situations. There are lots of “things” out there that are potential objects of our attention, but we attend to some things and ignore others. How do we accomplish this, and how does directing our attention affect our experience? We begin by considering how we can direct our attention by moving our eyes to look at one place after another. Scanning a Scene With Eye Movements Kevin Mazur/WireImage/Getty Images See how many people you can identify in Figure 4.11 in a minute. Go! As you did this task, you probably noticed that you had to scan the scene, checking each face in turn, in order to identify it. Scanning is necessary because good detail vision occurs only for things you are looking at directly. Another way to experience the fact that we have to look directly at things we want to see in detail is to look at the word at the end of this line and, without moving your eyes, see how many words you can read to the left. If you do this without cheating (resist the urge to look to the left!), you will find that although you can read the word you are looking at, you can read only a few of the words that are farther off to the side. Both of these tasks illustrate the difference between central vision and peripheral vision. Central vision is the area you are looking at. Peripheral vision is everything off to the side. Because of the way the retina is constructed, objects in central vision fall on a small area called the fovea, which has much better detail vision than the peripheral retina, on ➤ Figure 4.11 How many people can you identify in this photo in 1 minute? Copyright 2019 Cengage Learning. All Rights Reserved. May not be copied, scanned, or duplicated, in whole or in part. WCN 02-200-202 08271_ch04_ptg01.indd 102 4/18/18 4:20 PM Directing Attention by Scanning a Scene 103 Maria Wachala/Getty Images (Scanning measurements by James Brockmole) which the rest of the scene falls. Thus, as you scanned the scene in Figure 4.11, you were aiming your fovea at one face after another. Each time you briefly paused on one face, you were making a fixation. When you moved your eye to observe another face, you were making a saccadic eye movement—a rapid, jerky movement from one fixation to the next. It isn’t surprising that you were moving your eyes from one place to another, because you were trying to identify as many people as possible. But it may surprise you to know that even when you are freely viewing an object or scene without searching for anything in particular, you move your eyes about three times per second. This rapid scanning is ➤ Figure 4.12 Scan path of a person freely viewing a picture. Fixations are indicated by shown in Figure 4.12, which is a pattern the yellow dots and eye movements by the red lines. Notice that this person looked of fixations (dots) separated by saccadic preferentially at areas of the picture such as the statues but ignored areas such as the eye movements (lines) that occurred as water, rocks, and buildings. a participant viewed the picture of the fountain. Shifting attention from one place to another by moving the eyes is called overt attention because we can see attentional shifts by observing where the eyes are looking. We will now consider two factors that determine how people shift their attention by moving their eyes: bottom-up, based primarily on physical characteristics of the stimulus; and top-down, based on cognitive factors such as the observer’s knowledge about scenes and past experiences with specific stimuli. Attention can be influenced by stimulus salience—the physical properties of the stimulus, such as color, contrast, or movement. Capturing attention by stimulus salience is a bottom-up process because it depends solely on the pattern of light and dark, color and contrast in a stimulus (Ptak, 2012). For example, the task of finding the people with blonde hair in Figure 4.11 would involve bottom-up processing because it involves responding to the physical property of color, without considering the meaning of the image (Parkhurst et al., 2002). Determining how salience influences the way we scan a scene typically involves analyzing characteristics such as color, orientation, and intensity at each location in the scene and then combining these values to create a saliency map of the scene (Itti & Koch, 2000; Parkhurst et al., 2002; Torralba et al., 2006). For example, the person in red in Figure 4.13 would get high marks for salience, both for the brightness of the color and because it contrasts with the expanse of white, which has lower salience because it is homogeneous. Experiments in which people’s eyes were tracked as they observed pictures have found that the first few fixations are more likely on high-salience areas. But after the first few fixations, scanning begins to be influenced by top-down, or cognitive, processes Ales Fevzer/Corbis Documentary/Getty Images Scanning Based on Stimulus Salience ➤ Figure 4.13 The red shirt is visually salient because it is bright and contrasts with its surroundings. Copyright 2019 Cengage Learning. All Rights Reserved. May not be copied, scanned, or duplicated, in whole or in part. WCN 02-200-202 08271_ch04_ptg01.indd 103 4/18/18 4:20 PM 104 CHAPTER 4 Attention that depend on things such as the observers’ goals and expectations determined by their past experiences in observing the environment (Parkhurst et al., 2002). Scanning Based on Cognitive Factors (a) One way to show that where we look isn’t determined only by stimulus salience is by checking the eye movements of the participant looking at the scene in Figure 4.12. Notice that the person never looks at the bright blue water even though it is salient due to its brightness, color, and position near the front of the scene. The person also ignored the rocks and columns and several other prominent architectural features. Instead, the person focused on aspects of the fountain that might be more interesting, such as the statues. It is important to note, however, that just because this person looked at the statues doesn’t mean everyone would. Just as there are large variations between people, there are variations in how people scan scenes (Castelhano & Henderson, 2008; Noton & Stark, 1971). Thus, another person, who might be interested in the architecture of the buildings, might look less at the statues and more at the building’s windows and columns. This example illustrates top-down processing, because scanning is influenced by preferences a person brings to the situation. Top-down processing also comes into play when scanning is influenced by scene schemas—an observer’s knowledge about what is contained in typical scenes (see regularities of the environment, page 74). Thus, when Melissa Võ and John Henderson (2009) showed pictures like the ones in Figure 4.14, observers looked longer at the printer in Figure 4.14b than the pot in Figure 4.14a because a printer is less likely to be found in a kitchen. People look longer at things that seem out of place in a scene because their attention is affected by their knowledge of what is usually found in the scene. Another example of how cognitive factors based on knowledge of the environment influences scanning is an experiment by Hiroyuki Shinoda and coworkers (2001) in which they measured observers’ fixations and tested their ability to detect traffic signs as they drove through a computergenerated environment in a driving simulator. They found that the observers were more likely to detect stop signs positioned at intersections than those positioned in the middle of a block, and that 45 percent of the observers’ fixations occurred close to intersections. In this example, the observers are using learning about regularities in the environment (stop signs are usually at corners) to determine when and where to look for stop signs. Scanning Based on Task Demands (b) ➤ Figure 4.14 Stimuli used by Võ and Henderson (2009). Observers spent more time looking at the printer in (b) than at the pot in (a), shown inside the yellow rectangles (which were not visible to the observers). (Source: M.L.-H.Vo, & J. M. Henderson, Does gravity matter? Effects of semantic and syntactic inconsistencies on the allocation of attention during scene perception, Journal of Vision, 9, 3:24, 1–15, Figure 1) The examples in the last section demonstrate that knowledge of various characteristics of the environment can influence how people direct their attention. However, the last example, in which participants drove through a computer-generated environment, was different from the rest. The difference is that instead of looking at pictures of stationary scenes, participants were interacting with the environment. This kind of situation, in which people are shifting their attention from one place to another as they are doing things, occurs when people are moving through the environment, as in the driving example, and when people are carrying out specific tasks. Because many tasks require attention to different places as the task unfolds, it isn’t surprising that the timing of when people look at specific places is determined by the sequence of actions involved in the task. Consider, for example, the pattern of eye movements in Figure 4.15, which were measured as a person was making a peanut butter sandwich. The process of making Copyright 2019 Cengage Learning. All Rights Reserved. May not be copied, scanned, or duplicated, in whole or in part. WCN 02-200-202 08271_ch04_ptg01.indd 104 4/18/18 4:20 PM Outcomes of Attention 105 the sandwich begins with the movement of a slice of bread from the bag (A) to the plate (B). Notice that this operation C is accompanied by an eye movement from the bag to the plate. The observer then looks at the peanut butter jar just before it is lifted and looks at the top just before it is removed (C). A Attention then shifts to the knife, which is picked up and used B to scoop the peanut butter and spread it on the bread (Land & Hayhoe, 2001). The key finding of these measurements, and also of another experiment in which eye movements were measured as a C person prepared tea (Land et al., 1999), is that the person’s eye movements were determined primarily by the task. The person fixated on few objects or areas that were irrelevant to the task, and eye movements and fixations were closely linked to the action the ➤ Figure 4.15 Sequence of fixations of a person making a peanut person was about to take. Furthermore, the eye movement usually butter sandwich. The first fixation is on the loaf of bread. preceded a motor action by a fraction of a second, as when the (Source: Adapted from M. F. Land, N. Mennie, & J. Rusted, The roles of vision and eye movements in the control of activities of daily living, Perception, 28, 11, person first fixated on the peanut butter jar and then reached over 1311–1328. Copyright © 1999 by Pion Ltd, London. Reproduced by permission. to pick it up. This is an example of the “just in time” strategy— www.pion.co.uk and www.envplan.com.) eye movements occur just before we need the information they will provide (Hayhoe & Ballard, 2005; Tatler et al., 2011). The examples we have described in connection with scanning based on cognitive factors and task demands have something in common: They all provide evidence that scanning is influenced by people’s predictions (Henderson, 2017). Scanning anticipates what a person is going to do next as they make a peanut butter and jelly sandwich; scanning anticipates that stop signs are most likely to be located at intersections; and pausing scanning to look longer at an unexpected object occurs when a person’s expectations are violated, as when a printer unexpectedly appears in a kitchen. Outcomes of Attention What do we gain by attending? Based on the last section, which described overt attention that is associated with eye movements, we might answer that question by stating that shifting attention by moving our eyes enables us to see places of interest more clearly. This is extremely important, because it places the things we’re interested in front-and-center where they are easy to see. But some researchers have approached attention not by measuring factors that influence eye movements, but by considering what happens when we shift our attention without making eye movements. Shifting attention while keeping the eyes still is called covert attention, because the attentional shift can’t be seen by observing the person. This type of attending involves shifting attention “with the mind” as you might do when you are paying attention to something off to the side while still looking straight ahead. (This has also been described as “looking out of the corner of your eye.”) One reason some researchers have studied covert attention is that it is a way of studying what is happening in the mind without the interference of eye movements. We will now consider research on covert attention, which shows how shifting attention “in the mind” can affect how quickly we can respond to locations and to objects, and how we perceive objects. Attention Improves Our Ability to Respond to a Location In a classic series of studies, Michael Posner and coworkers (1978) asked whether paying attention to a location improves a person’s ability to respond to stimuli presented there. To answer this question, Posner used a procedure called precueing. Copyright 2019 Cengage Learning. All Rights Reserved. May not be copied, scanned, or duplicated, in whole or in part. WCN 02-200-202 08271_ch04_ptg01.indd 105 4/18/18 4:20 PM 106 CHAPTER 4 Attention METHOD Precueing The general principle behind a precueing experiment is to determine whether presenting a cue indicating where a test stimulus will appear enhances the processing of the target stimulus. The participants in Posner and coworkers’ (1978) experiment kept their eyes stationary throughout the experiment, always looking at the 1 in the display in Figure 4.16, so Posner was measuring covert attention. Participants first saw an arrow cue (as shown in the left panel) indicating on which side of the display they should focus their attention. In Figure 4.16a, the arrow cue indicates that they should focus their attention to the right (while looking steadily at the 1). Their task was to press a key as rapidly as possible when a target square was presented off to the side (as shown in the right panel). The trial shown in Figure 4.16a is a valid trial because the target square appears on the side indicated by the cue arrow. On 80 percent of the trials, the cue arrow directed participants’ attention to the side where the target square appeared. However, on 20 percent of the trials, the arrow directed the participant’s attention away from where the target was to appear (Figure 4.16b). These were the invalid trials. On both the valid and invalid trials, the participant’s task was the same—to press the key as quickly as possible when the target square appeared. See cue Respond to target + + + + (a) Valid trial (b) Invalid trial Reaction time (ms) 325 300 275 250 225 200 0 (c) Results Valid Invalid ➤ Figure 4.16 Procedure for (a) valid trials and (b) invalid trials in Posner et al.’s (1978) precueing experiment; (c) the results of the experiment. The average reaction time was 245 ms for valid trials but 305 ms for invalid trials. (Source: M. I. Posner, M. J. Nissen, & W. C. Ogden, Modes of perceiving and processing information. Copyright © 1978 by Taylor & Francis Group LLC–Books.) The results of this experiment, shown in Figure 4.16c, indicate that participants reacted to the square more rapidly when their attention was focused on the location where the signal was to appear. Posner interpreted this result as showing that information processing is more effective at the place where attention is directed. This result and others like it gave rise to the idea that attention is like a spotlight or zoom lens that improves processing when directed toward a particular location (Marino & Scholl, 2005). Attention Improves Our Ability to Respond to Objects In addition to covertly attending to locations, as in Posner’s experiment, we can also covertly attend to specific objects. We will now consider some experiments that show that (1) attention can enhance our response to objects and (2) when attention is directed to one place on an object, the enhancing effect of that attention spreads to other places on the object. Consider, for example, the experiment diagrammed in Figure 4.17 (Egly et al., 1994). As participants kept their eyes on the +, one end of the rectangle was briefly highlighted (Figure 4.17a). This was the cue signal that indicated where a target, a dark square (Figure 4.17b), would probably appear. In this example, the cue indicates that the target is likely to appear in position A, at the upper part of the right rectangle, and the target is, in fact, presented at A. (The letters used to illustrate positions in our description did not appear in the actual experiment.) The participants’ task was to press a button when the target was presented anywhere on the display. The numbers indicate the reaction times, in milliseconds, for three target locations when the cue signal had been presented at A. Not surprisingly, participants responded most rapidly when the target was presented at A, where the cue had been presented. However, the most interesting result is that participants responded more rapidly when the target was presented Copyright 2019 Cengage Learning. All Rights Reserved. May not be copied, scanned, or duplicated, in whole or in part. WCN 02-200-202 08271_ch04_ptg01.indd 106 4/18/18 4:20 PM Outcomes of Attention at B (reaction time 5 358 ms) than when the target was presented at C (reaction time 5 374 ms). Why does this occur? It can’t be because B is closer to A than C, because B and C are exactly the same distance from A. Rather, B’s advantage occurs because it is located within the object that was receiving the participant’s attention. Attending at A, where the cue was presented, causes the maximum effect at A, but the effect of this attention spreads throughout the object so some enhancement occurs at B as well. The faster responding that occurs when enhancement spreads within an object is called the same-object advantage (Marino & Scholl, 2005; also see Driver & Baylis, 1989, 1998; Katzner et al., 2009; Lavie & Driver, 1996; and Malcolm & Shomstein, 2015 for more demonstrations of how attention spreads throughout objects). Attention Affects Perception 107 Cue A C 374 ms C + D A 324 ms B 358 ms + B D Present cue...................Cue off...................Present target (a) (b) ➤ Figure 4.17 In Egly and coworkers’ (1994) experiment, (a) a cue signal appears at one place on the display, then the cue is turned off and (b) a target is flashed at one of four possible locations, A, B, C, or D. The participants’ task was to press a button when the target was presented anywhere on the display. Numbers are reaction times in ms for positions A, B, and C when the cue signal appeared at position A. Returning to the quote by James at the beginning of the chapter, let’s focus on his description of attention to objects as “taking possession by the mind in clear and vivid form.” The phrase in clear and vivid form suggests that attending to an object makes it more clear and vivid—that is, attention affects perception. More than 100 years after James’s suggestion, many experiments have shown that attended objects are perceived to be bigger and faster, and to be more richly colored and have better contrast than non attended objects (Anton-Erxleben et al., 2009; Carrasco et al., 2004; Fuller & Carrasco, 2006; Turatto et al., 2007). Attention therefore not only causes us to respond faster to locations and objects but affects how we perceive the object (Carrasco, 2011). Attention Affects Physiological Responding Attention has a number of different effects on the brain. One effect is to increase activity in areas of the brain that represent the attended location. Attention to Locations Increases Activity in Specific Areas of the Brain What happens in the brain when people shift their attention to different locations while keeping their eyes stationary? Ritobrato Datta and Edgar DeYoe (2009) answered this question by measuring brain activity using fMRI as participants kept their eyes fixed on the center of the display in Figure 4.18 and shifted their attention to different locations in the display. The colors in the circles in Figure 4.18b indicate the area of brain that was activated when the participant directed his or her attention to different locations indicated by the letters on the stimulus in Figure 4.18a. Notice that the yellow “hot spot,” which is the place of greatest activation, moves out from the center and also becomes larger as attention Stimulus disc A Attention to one area A B B C C (a) Always looking at center of disc (b) ➤ Figure 4.18 (a) Participants in Datta and DeYoe’s (2009) experiment directed their attention to different areas of this circular display while keeping their eyes fixed on the center of the display. (b) Activation of the brain that occurred when participants attended to the areas indicated by the letters on the stimulus disc. The center of each circle is the place on the brain that corresponds to the center of the stimulus. The yellow “hot spot” is the area of the brain that is maximally activated by attention. (Source: From R. Datta & E. A. DeYoe, I know where you are secretly attending! The topography of human visual attention revealed with fMRI, Vision Research, 49, 1037–1044, 2009.) Copyright 2019 Cengage Learning. All Rights Reserved. May not be copied, scanned, or duplicated, in whole or in part. WCN 02-200-202 08271_ch04_ptg01.indd 107 4/18/18 4:20 PM 108 CHAPTER 4 Attention is directed farther from the center. By collecting brain activation data for all of the locations on the stimulus, Datta and DeYoe created “attention maps” that show how directing attention to a specific area of space activates a specific area of the brain. What makes this experiment even more interesting is that after attention maps were determined for a particular participant, that participant was told to direct his or her attention to a “secret” place, unknown to the experimenters. Based on the location of the resulting yellow “hot spot” in the brain, the experimenters were able to predict, with 100 percent accuracy, the “secret” place where the participant was attending. Attention Changes the Representation of Objects Across the Cortex Datta and DeYoe’s “hot spot” experiment is an elegant demonstration of how attention directed to a specific location results in enhanced activity at one place in the cortex. But what about a situation in which people might be directing their attention to numerous different locations as they search for something in a naturalistic environment? Tolga Cukur and coworkers (2013) considered this question by determining how attention affects the way different types of objects are represented across the brain as a whole. The starting point for Cukur’s experiment was Alex Huth’s (2012) brain map that we described in Chapter 2 (see Figure 2.20). Huth’s map illustrates how different categories of objects and actions are represented by activity that is distributed across a large area of the brain. Huth determined this map by having participants view movies in a scanner, and using fMRI to determine brain activity when different things were happening on the screen (see Figure 2.19). Cukur did the same thing as Huth (they were working in the same laboratory and were involved in both papers), but instead of having his observers passively view the movies, he gave them a task that involved searching for either “humans” or “vehicles.” A third group passively viewed the films, as in Huth’s experiment. Figure 4.19 shows what happened by plotting how a single voxel in the brain (see page 41 to review voxel) responded to different types of stimuli under two different search conditions. Notice in (a) that when the observer is searching for “humans” in the movie, the voxel responds well to “person,” slightly to “animal,” and hardly at all to “building” and “vehicle.” However, in (b), when the observer is searching for “vehicle,” the voxel’s tuning shifts so it now responds well to “vehicle,” slightly to “building,” but not to “person” or “animal.” By analyzing the data from tens of thousands of voxels across the brain, Cukur created the whole brain maps shown in Figure 4.20. The colors indicate tuning to different categories. The most obvious difference between the search-for-people brain and the search-for-vehicles brain occurs at the top of the brain in this view. Notice that in the person condition there are more yellows and greens, which represent people or things related to people like body parts, animals, groups, and talking. However, in the vehicles condition, Natural movies Single voxel tuning Animal Person … Building Vehicle Animal Person … Building Vehicle Search for “vehicles” moodboard/Alamy Stock Photo Search for “humans” ➤ Figure 4.19 How tuning of a single voxel is affected by attention to (a) humans and (b) vehicles. Copyright 2019 Cengage Learning. All Rights Reserved. May not be copied, scanned, or duplicated, in whole or in part. WCN 02-200-202 08271_ch04_ptg01.indd 108 4/18/18 4:20 PM Outcomes of Attention (b) Search for people Key: Colors on the brain associated with specific objects Body parts, plants Text, graphs Body parts Building, furniture Body parts, humans Devices, artifacts Humans, talking Vehicles (c) Search for vehicles Geography, roads Movement Carnivores, mammals Animals Huth and Gallant (a) Passive view 109 ➤ Figure 4.20 The map of categories on the brain changes for viewing a film. Colors indicate activation caused by different categories of stimuli. (a) Passive view indicates activation when not searching for anything. (b) Search for people causes activation indicated by yellow and green, which stand for people and things related to people. (c) Search for vehicles causes activation indicated by reds, which stand for vehicles and things related to vehicles. colors shift to reds, which represent vehicles or things related to vehicles such as movement, road, and devices. An important feature of these brain maps is that looking for a particular category shifts responding to the category and to additional things related to that category, so looking for people also affects responding to groups and clothing. Cukur calls this effect attentional warping—the map of categories on the brain changes so more space is allotted to categories that are being searched for, and this effect occurs even when the attended category isn’t present in the movie. For example, when a person is on the lookout for vehicles, the brain becomes “warped” or “tuned” so that large areas respond best to vehicles and things related to vehicles. Then, when a vehicle, a road, or movement appears in a scene, a large response occurs. Other things, which the person is not looking for at the moment, would cause smaller responses. T E ST YOUR SELF 4.2 1. What is the difference between central vision and peripheral vision? How is this difference related to overt attention, fixations, and eye movements? 2. What is stimulus salience? How is it related to attention? 3. Describe some examples of how attention is determined by cognitive factors. What is the role of scene schemas? 4. Describe the peanut butter experiment. What does the result tell us about the relation between task demands and attention? 5. What is covert attention? Describe the precueing procedure used by Posner. What does the result of Posner’s experiment indicate about the effect of attention on information processing? 6. Describe the Egly precueing experiment. What is the same-object advantage, and how was it demonstrated by Egly’s experiment? 7. What are three behaviorally measured outcomes of attention? 8. Describe how Data and DeYoe showed that attention to a location affects activity in the brain. 9. Describe Cukur’s experiment, which showed how attention changes the representation of objects across the cortex. Copyright 2019 Cengage Learning. All Rights Reserved. May not be copied, scanned, or duplicated, in whole or in part. WCN 02-200-202 08271_ch04_ptg01.indd 109 4/18/18 4:20 PM 110 CHAPTER 4 Attention Divided Attention: Can We Attend to More Than One Thing at a Time? Our emphasis so far has been on attention as a mechanism for focusing on one thing at a time. We have seen that sometimes we take in information from a task-irrelevant stimulus, even when we are trying ignore irrelevant stimuli, as in Forster and Lavie’s experiment and the Stroop task. But what if you want to purposely distribute your attention among a few tasks? Is it possible to pay attention to more than one thing at a time? Although you might be tempted to answer “no,” based on the difficulty of listening to two conversations at once, there are many situations in which divided attention—the distribution of attention among two or more tasks—can occur, as when Roger was able to play his cell phone game and listen in on the nearby conversation. Also, people can simultaneously drive, have conversations, listen to music, and think about what they’re going to be doing later that day (although this may not hold for difficult driving conditions). As we will see, the ability to divide attention depends on a number of factors, including practice and the difficulty of the task. Divided Attention Can Be Achieved With Practice: Automatic Processing Experiments by Walter Schneider and Richard Shiffrin (1977) involved divided attention because they required the participant to carry out two tasks simultaneously: (1) holding information about target stimuli in memory and (2) paying attention to a series of “distractor” stimuli to determine whether one of the target stimuli is present among these distractor stimuli. Figure 4.21 illustrates the procedure. The participant was shown a memory set like the one in Figure 4.21a, consisting of one to four characters called target stimuli. The memory set was followed by rapid presentation of 20 “test frames,” each of which contained distractors (Figure 4.21b). On half of the trials, one of the frames contained a target stimulus from the memory set. A new memory set was presented on each trial, so the targets changed from trial to trial, followed by new test frames. In this example, there is one target stimulus in the memory set, there are four stimuli in each frame, 3 and the target stimulus 3 appears in one of the frames. At the beginning of the experiment, the participants’ per(a) Present target stimulus in memory set formance was only 55 percent correct; it took 900 trials for performance to reach 90 percent (Figure 4.22). Participants reported that for the first 600 trials, they had to keep repeating the target items in each memory set in order to remember them. K R C T 3 H ...... (Although targets were always numbers and distractors letters, M G V L F J remember that the actual targets and distractors changed from trial to trial.) However, participants reported that after about (b) Present series of 20 test frames (fast!) 600 trials, the task had become automatic: The frames appeared and participants responded without consciously thinking about it. They would do this even when as many as four targets had (c)