Ch 25 Lecture Outline PDF
Document Details
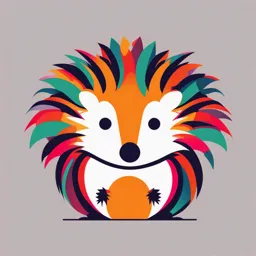
Uploaded by MajesticNovaculite
Tags
Summary
This document is a lecture outline covering the topic of body fluids, fluid balance, and electrolyte regulation in the body. It details the components of fluid balance, including intake, output, and the role of hormones in the regulation of fluid. It is suitable for an undergraduate level course in biology, physiology, or related fields.
Full Transcript
1 Chapter 25 Lecture Outline © 2019 McGraw-Hill Education 25.1 Body Fluids Learning Objectives: 1. State the percentage of body fluid, and explain the significance of an individual’s percentage relative to fluid balance. 2. List the factors that influence the percentage of body fluid. 3. Descri...
1 Chapter 25 Lecture Outline © 2019 McGraw-Hill Education 25.1 Body Fluids Learning Objectives: 1. State the percentage of body fluid, and explain the significance of an individual’s percentage relative to fluid balance. 2. List the factors that influence the percentage of body fluid. 3. Describe the two major body fluid compartments, and compare their chemical compositions. 4. Explain how fluid moves between the major body fluid compartments. © 2019 McGraw-Hill Education 2 3 25.1a Percentage of Body Fluid 1 Human body is between 45% and 75% fluid Depends on age and amount of adipose tissue/muscle tissue Age • Infants—highest percentage of fluid • Elderly—lowest percentage • Children, young and middle-aged adults—in middle range • Body fluid decreases with age © 2019 McGraw-Hill Education 4 25.1a Percentage of Body Fluid 2 Relative amounts of adipose to skeletal muscle tissue • Adipose tissue is about 20% water • Skeletal muscle is about 75% water • Males have more skeletal muscle, so slightly higher % • % decreases with increased body fat Determines susceptibility to fluid imbalance • Individuals with lower percentage, at higher risk • E.g., elderly at more risk than young adults © 2019 McGraw-Hill Education 5 Fluid Compartments Figure 25.1 © 2019 McGraw-Hill Education 6 25.1b Fluid Compartments 3 Specific extracellular fluids • Cerebrospinal fluid • Synovial joint fluid • Aqueous and vitreous humor of the eye • Fluids of inner ear • Serous fluids within body cavity Not typically subject to significant daily gains and losses © 2019 McGraw-Hill Education 7 Percentages of Solutes in Body Fluids Figure 25.2 © 2019 McGraw-Hill Education 8 Fluid Movement Following Fluid Intake Figure 25.3a © 2019 McGraw-Hill Education 9 Fluid Movement if Dehydrated Figure 25.3b © 2019 McGraw-Hill Education Section 25.1 What did you learn? 1. If you have an increase in muscle mass as a result of weight training, will your percentage of body fluid increase, decrease, or stay the same? Explain. 2. Which ions are more prevalent in the intracellular fluid? Which are more prevalent in the extracellular fluid? 3. What is the major distinction in the chemical composition of blood plasma and interstitial fluid? 4. When you are dehydrated, is the net movement of fluid from the blood plasma into the cells or from the cells into the blood plasma? © 2019 McGraw-Hill Education 10 25.2 Fluid Balance Learning Objectives: 1 5. Define fluid balance. 6. List the sources of fluid intake. 7. Distinguish between the categories of water loss. 8. Name the different causes of fluid imbalance. 9. Compare and contrast the different types of fluid imbalances. © 2019 McGraw-Hill Education 11 25.2 Fluid Balance Learning Objectives: 2 10. Explain what is meant by fluid sequestration. 11. Describe the stimuli that increase fluid intake. 12. Explain the conditions and stimuli that decrease fluid intake. 13. Name the four hormones that are involved in regulating fluid output. © 2019 McGraw-Hill Education 12 13 25.2a Fluid Intake and Fluid Output Fluid balance • Fluid intake equal to fluid output • Normal distribution of water and solutes in both compartments • Influenced by multiple body systems • They help bring fluid into the body • Participate in fluid loss • Regulate the processes of fluid balance © 2019 McGraw-Hill Education 1 14 Fluid Intake and Fluid Output Figure 25.4 © 2019 McGraw-Hill Education 15 25.2a Fluid Intake and Fluid Output 4 • Sensible water loss • Measurable • Includes fluid lost through feces and urine • Insensible water loss • Not measurable • Fluid lost in expired air • Fluid lost from skin through sweat and cutaneous transpiration © 2019 McGraw-Hill Education 16 25.2a Fluid Intake and Fluid Output • Obligatory water loss • Loss of water that always occurs • Water lost through breathing and through the skin • Water lost through feces and minimal urine needed to eliminate waste • Facultative water loss • Controlled water loss • Dependent on hydration of body • Hormonally regulated in kidney nephrons • Only mechanism to control fluid output • Can decrease fluid loss if body is dehydrated © 2019 McGraw-Hill Education 5 17 25.2b Fluid Imbalance 1 Fluid imbalance occurs if fluid output does not equal fluid intake Organized into five categories • Volume depletion, volume excess, dehydration, hypotonic hydration, and fluid sequestration First four of these can be differentiated by two criteria: © 2019 McGraw-Hill Education 1. Does the fluid imbalance change the osmolarity of body fluid? 2. Is the fluid imbalance caused by excess or deficiency of body fluid? 18 25.2b Fluid Imbalance 2 Fluid imbalance with constant osmolarity • Occurs when isotonic fluid is lost or gained • Volume depletion • Occurs when isotonic fluid loss is greater than isotonic fluid gain • E.g., hemorrhage, severe burns, chronic vomiting, diarrhea, hyposecretion of aldosterone • Volume excess • Isotonic fluid gain is greater than isotonic fluid loss • Fluid intake normal but decreased fluid loss through kidneys • In both, no change in osmolarity • No net movement of water between compartments © 2019 McGraw-Hill Education 19 25.2b Fluid Imbalance 3 Fluid imbalance with changes in osmolarity • Dehydration: Water loss greater than loss of solutes • Results from profuse sweating, diabetes, intake of alcohol, hyposecretion of antidiuretic hormone (ADH), insufficient water intake, overexposure to cold weather • Blood plasma becomes hypertonic • Water shifts from cells into interstitial fluid and blood plasma • Possible dehydration of body cells © 2019 McGraw-Hill Education 20 25.2b Fluid Imbalance 4 Fluid imbalance with changes in osmolarity (continued) • Hypotonic hydration: water gain or retention that is greater than solute gain or retention • Water intoxication • Can result from ADH hypersecretion • Usually from drinking large amount of plain water • Both Na+ and water lost during sweating • Drinking water only replaces the water, not the solutes • Plasma becomes hypotonic • Fluid moving from blood plasma into interstitial fluid and into cells • Possible swelling of cells • Cerebral edema due to brain cells swelling • Convulsions, coma, and death in severe cases © 2019 McGraw-Hill Education 21 25.2b Fluid Imbalance 5 Fluid sequestration Total body fluid is normal, but distributed abnormally Examples: • Edema, puffiness with fluid accumulation in interstitial space • Ascites, accumulation of fluid within peritoneal cavity • Pericardial effusion, accumulation of fluid in pericardial cavity • Pleural effusion, accumulation of fluid in pleural cavity © 2019 McGraw-Hill Education 22 Edema Figure 25.5 © 2019 McGraw-Hill Education ©Bob Tapper/Medical Images 23 25.2c Regulation of Fluid Balance Means of regulating fluid balance • Monitor blood volume • Blood pressure • Blood plasma osmolarity Relationships between these variables: • Fluid intake increases blood volume • Increases blood pressure • Decreases blood osmolarity if water gain exceeds solute gain • Fluid output decreases blood volume • Decreases blood pressure • Increases blood osmolarity if more water is lost than solutes © 2019 McGraw-Hill Education 1 24 25.2c Regulation of Fluid Balance 2 Regulating fluid intake • Intake regulated by various stimuli that activate/inhibit the thirst center Stimuli to turn on the thirst center • Decreased blood volume and blood pressure • • Renin released from kidney, results in production of angiotensin II Angiotensin II stimulates thirst center • Increased blood osmolarity • • • Due to insufficient water intake Stimulates sensory receptors in thirst center directly Stimulates hypothalamus to trigger release of ADH • Decreased salivary secretions • © 2019 McGraw-Hill Education Sensory input relayed from receptors in mucous membranes 25 25.2c Regulation of Fluid Balance 3 Stimuli to turn off the thirst center • Fluid intake is greater than fluid output • Increased blood volume and blood pressure • Inhibits kidney from releasing renin • Decrease in angiotensin II results in reduced stimulation of thirst center • Decreased blood osmolarity • Thirst center is no longer stimulated directly • Decreased stimulation of ADH release • Increased salivary secretions • Decreased sensory input to thirst center • Distension of stomach • Stretch caused by fluid entering • Inhibitory sensory impulses relayed to thirst center © 2019 McGraw-Hill Education 26 25.2c Regulation of Fluid Balance 4 Regulating fluid output • Regulated through kidneys by controlling urine output • Four hormones involved in regulating urine output • Angiotensin II, antidiuretic hormone(ADH), and aldosterone • Decrease urine output to increase blood volume and pressure • Atrial natriuretic peptide (ANP) • Increases urine output to decrease blood volume and pressure © 2019 McGraw-Hill Education Section 25.2 What did you learn? 5. What are the two major sources of fluid intake? What are two ways that fluid output is categorized, and which one is based on the hydrated state of the body? 6. How would you distinguish fluid deficiency from dehydration in terms of changes in total body fluid, changes in blood osmolarity, and fluid movement between compartments? 7. What stimuli activate the thirst center? 8. Which of these four hormones—angiotensin II, antidiuretic hormone, aldosterone, and atrial natriuretic peptide—increases urine output? © 2019 McGraw-Hill Education 27 25.3 Electrolyte Balance Learning Objectives: 14. Describe the difference between a nonelectrolyte and an electrolyte. 15. Explain the general role of electrolytes in fluid balance. 16. List the six major electrolytes found in body fluids, other than H+ and HCO3–. 17. Explain why Na+ is a critical electrolyte in the body. 18. Describe the variables that influence K + distribution. 19. Identify the main location, functions, and means of regulation for each of the common electrolytes. © 2019 McGraw-Hill Education 28 29 25.3a Nonelectrolytes and Electrolytes Nonelectrolytes • Molecules that do not dissociate in solution • Most covalently bonded organic molecules Electrolytes • Dissociate in solution to form cations and anions • Ability of substances to conduct electrical current when dissolved • Each with unique function and osmotic functions • Concentration given as milliequivalents/L (mEq/L) • Electrical charges in 1 L solution © 2019 McGraw-Hill Education 25.3b Major Electrolytes: Location, Functions, and Regulation 1 Sodium ion (Na+) • 99% in ECF and 1% in ICF • Gradient maintained by Na+/K+ pumps • Principle cation in ECF • Exerts greatest osmotic pressure Sodium balance • Normal concentration 135–145 mEq/L • Daily requirement about 2 g/day • Intake may vary from 0.5 g to 20 g • Lost through urine, feces, and sweat • Amount lost in urine regulated by aldosterone, ADH, and ANP © 2019 McGraw-Hill Education 30 Sodium’s role in blood plasma osmolarity • Most important electrolyte in determining blood plasma osmolarity and regulating fluid balance • ECF hypertonic if Na+ concentration increased • © 2019 McGraw-Hill Education • Due to increased Na+ or decreased water content • Water moving from other compartments into blood plasma until normal concentration returned ECF hypotonic if Na+ concentration decreased • Due to decreased Na+ or increased water content • Water moving from plasma into cells until normal concentration reestablished 31 25.3b Major Electrolytes: Location, Functions, and Regulation 3 Sodium’s role in blood plasma osmolarity (continued) • Changes in plasma volume • Retention of Na+ and water causes increase in blood volume and blood pressure • Loss of Na+ and water with opposite effects • Individuals with high blood pressure are put on low-salt diet • Sodium imbalance • Most common type of electrolyte imbalance • Above normal level, hypernatremia • Below normal level, hyponatremia • Most changes resulting from changes in water content © 2019 McGraw-Hill Education 32 33 Sodium Balance Figure 25.6a © 2019 McGraw-Hill Education 25.3b Major Electrolytes: Location, Functions, and Regulation 4 Potassium ion (K+) • 98% in ICF, 2% in ECF • Maintained by Na+/K+ pump • Principle cation exerting intracellular osmotic pressure • Required for neuromuscular activities and controlling heart rhythm Potassium balance • Only the portion in ECF is regulated • Normal range, 3.5–5.0 mEq/L • Most lethal of electrolyte imbalances • Cardiac or respiratory arrest © 2019 McGraw-Hill Education 34 25.3b Major Electrolytes: Location, Functions, and Regulation 5 Potassium balance (continued) • Total body potassium • Regulated by K+ intake and output • Dietary requirement about 40 mEq/L from fruits and vegetables • Most K+ lost in the urine • Amount fluctuates • Greater amounts are lost if plasma K+ high, increased aldosterone secretion, and high blood pH • Potassium distribution • Dependent activity of Na+ /K+ pumps and K+ leak channels © 2019 McGraw-Hill Education 35 25.3b Major Electrolytes: Location, Functions, and Regulation 6 • Change in blood plasma K+ concentration cause shifts in K+ between ECF and ICF • With increase in plasma K+ (hyperkalemia), K+ moves from ECF into ICF • With decrease in plasma K+ (hypokalemia), K+ moves from ICF to ECF © 2019 McGraw-Hill Education 36 37 Potassium Balance Figure 25.7a © 2019 McGraw-Hill Education 25.3b Major Electrolytes: Location, Functions, and Regulation 8 Chloride ion (Cl–) • Associated with Na+ • Follows Na+ by electrostatic interactions • Amount lost in urine dependent upon blood plasma Na+ • Regulated by same mechanisms • Most abundant anion in ECF • Obtained in diet, lost in sweat, stomach secretions, and urine • Increased blood chloride levels: hyperchloremia • Decreased blood chloride levels: hypochloremia © 2019 McGraw-Hill Education 38 25.3b Major Electrolytes: Location, Functions, and Regulation 9 Calcium ion (Ca2+) • Most abundant electrolyte in bone and teeth • 99% stored here • Moved by pumps out of cells or into sarcoplasmic reticulum • Prevents binding phosphate within cells and hardening • Needed for muscle contraction and neurotransmitter release • Serves as a second messenger and participates in blood clotting • Obtained in diet, lost in urine, feces, and sweat • Increased blood calcium levels: hypercalcemia • Decreased blood calcium levels: hypocalcemia © 2019 McGraw-Hill Education 39 25.3b Major Electrolytes: Location, Functions, and Regulation 10 Phosphate ion (PO43-) • Most abundant anion in ICF • 85% stored in bone and teeth as calcium phosphate • Component of DNA, RNA, and phospholipids • Intracellular buffer and urine buffer • Most ionized (90%) in blood plasma, rest bound to proteins • Regulated by many of same mechanisms as Ca2+ © 2019 McGraw-Hill Education 40 25.3b Major Electrolytes: Location, Functions, and Regulation 11 Magnesium ion (Mg2+) • Primarily within bone or within cells • Cation in ICF • Participates in hundreds of enzymatic reactions • Important in muscle relaxation • Obtained from beans and peas, leafy green veggies • Lost from body in sweat and urine • Regulated through the kidney © 2019 McGraw-Hill Education 41 Section 25.3 What did you learn? 9. Why do electrolytes exert a greater osmotic pressure than nonelectrolytes? 10. What is the net direction of K+ movement in response to a decrease in pH? Explain. © 2019 McGraw-Hill Education 42 25.4 Hormonal Regulation Learning Objectives: 1 20. Explain the means by which angiotensin II formation can be triggered. 21. List the four primary effects of angiotensin II. 22. Explain how release of antidiuretic hormone (ADH) occurs from the posterior pituitary. 23. Describe the three actions of antidiuretic hormone. © 2019 McGraw-Hill Education 43 25.4 Hormonal Regulation Learning Objectives: 2 24. List three conditions that lead to aldosterone release. 25. Describe the changes that occur in response to binding of aldosterone by kidney cells. 26. Describe the stimulus for the release of atrial natriuretic peptide (ANP) and its three actions. 27. Explain the ways in which the effects of atrial natriuretic peptide differ from the effects of angiotensin II, ADH, and aldosterone. © 2019 McGraw-Hill Education 44 45 Renin-Angiotensin System Figure 25.8 © 2019 McGraw-Hill Education Actions and Effects of Antidiuretic Hormone Figure 25.9 © 2019 McGraw-Hill Education 46 47 Clinical View: Diabetes Insipidus Hyposecretion of ADH or inability of kidney to respond to ADH Decreased fluid retention Increased urine production Can loose up to 20 L of fluid daily © 2019 McGraw-Hill Education 48 Action and Effects of Aldosterone Figure 25.10 © 2019 McGraw-Hill Education Actions and Effects of Atrial Natriuretic Peptide Figure 25.11 © 2019 McGraw-Hill Education 49 Section 25.4 What did you learn? 11. How does angiotensin II alter fluid output and potentially alter fluid intake? 12. How does the homeostatic system involving ADH function? Include how it is released and its actions. 13. How does aldosterone influence the contents and volume of fluid output? 14. How does ANP influence fluid output, blood volume, and systemic blood pressure? © 2019 McGraw-Hill Education 50 25.5 Acid-Base Balance Learning Objectives: 1 28. Distinguish between the two categories of acids in the body. 29. Name the two buffering systems that regulate each category. 30. List the various sources of fixed acid. 31. Describe how the kidneys counteract increasing blood H+. 32. Explain how the kidneys function in response to decreasing blood H+. © 2019 McGraw-Hill Education 51 25.5 Acid-Base Balance Learning Objectives: 2 33. Explain the normal relationship between breathing rate and acid-base balance. 34. Describe the components of the protein buffering system and where and how they help prevent pH changes. 35. Explain the reactions of the phosphate buffering system within the ICF. 36. Discuss how the bicarbonate buffering system maintains acid-base balance in the ECF. © 2019 McGraw-Hill Education 52 53 25.5a Categories of Acid 1 Normal pH = 7.35 to 7.45, slightly alkaline Proper pH balance critical to body functions pH inversely related to H+ concentration • Adding an acid increases H+, base reduces it Two categories of acids in body—fixed and volatile Fixed acid • Wastes produced from metabolic processes • E.g., lactic acid from glycolysis • E.g., phosphoric acid from nucleic acid metabolism • E.g., ketoacids from metabolism of fat • Regulated by the kidney © 2019 McGraw-Hill Education 54 25.5a Categories of Acid 2 Volatile acid • Carbonic acid produced when carbon dioxide combines with water in the presence of the enzyme carbonic anhydrase • Referred to as “volatile” because it is produced from an expired or “evaporated” gas • Regulated by respiratory system © 2019 McGraw-Hill Education Increased Blood H+ Concentration and Adjustments by the Kidneys Figure 25.12a © 2019 McGraw-Hill Education 55 Decreased Blood H+ Concentration and Adjustments by the Kidneys Figure 25.12b © 2019 McGraw-Hill Education 56 25.5c Respiration and Regulation of Volatile Acid Respiratory system regulates level of carbonic acid When the body is at rest: • CO2 normally eliminated from lungs at same rate produced During exercise: • CO2, H+, and O2 levels detected by chemoreceptors • Relayed to respiration center to alter breathing rate • Changes in CO2—most important variable • Carbonic acid levels dependent on CO2 levels CO2 and H2CO3 do not usually have daily fluctuations and do not normally affect acid-base balance © 2019 McGraw-Hill Education 57 Respiration and Maintenance of Normal Blood CO2 Levels Figure 25.13 © 2019 McGraw-Hill Education 58 59 25.5d Chemical Buffers 1 Chemical buffering systems • Act in minutes to temporarily prevent pH changes • Composed of one or two types of molecules • Can bind and release H+ within a fraction of a second • Composed of a weak base and weak acid • Weak base can bind excess H+ • Weak acid can release H+ • Temporary and limited, until physiologic buffering systems can eliminate the excess acid or base • The three most important chemical buffering systems: 1. protein—within cells and the blood 2. phosphate—within cells 3. bicarbonate—within ECF, particularly bloods © 2019 McGraw-Hill Education 60 25.5d Chemical Buffers Protein buffering system • Proteins within cells and blood plasma • Accounts for 3/4 of chemical buffering in body fluids • Intracellular protein, plasma proteins, hemoglobin • Help minimize pH changes throughout body • Amine group • Acts as a weak base to buffer acid • Carboxylic acid • Acts as a weak acid to buffer base © 2019 McGraw-Hill Education 2 61 25.5d Chemical Buffers 3 Phosphate buffering system • Found in ICF • Effective in buffering metabolic acid produced by cells • Composed of a weak base and weak acid • Weak base, hydrogen phosphate (HPO42–) • Weak acid, dihydrogen phosphate (H2PO4–) © 2019 McGraw-Hill Education 62 25.5d Chemical Buffers 4 Bicarbonate buffer system • Most important buffering system in ECF • Composed of a weak base and weak acid • Weak base, bicarbonate (HCO3–) • Weak acid, carbonic acid (H2CO3) • Buffering capacity—limitation in amount of acid or base that the chemical buffering systems can buffer © 2019 McGraw-Hill Education Section 25.5 What did you learn? 15. What is meant by acid-base balance? 16. How are fixed acids distinguished from volatile acids? 17. How do the kidneys regulate fixed acids to help maintain blood pH in response to increased blood H+ concentration? 18. Explain why respiration does not normally influence acidbase balance. 19. What are the three chemical buffering systems, and where do they function? 20. What is the general amount of time required to maintain pH by (a) the chemical buffering systems, (b) the respiratory system, and (c) the kidneys? © 2019 McGraw-Hill Education 63 25.6 Disturbances to Acid-Base Balance Learning Objectives: 1 37. Explain acid-base disturbance, compensation, and acid-base imbalance. 38. Define respiratory acidosis, identify some of the causes of this type of acid-base disturbance, and explain how it occurs. 39. Explain why infants are more susceptible to respiratory acidosis. 40. Define respiratory alkalosis, identify some of the causes of this type of acid-base imbalance, and explain how it occurs. © 2019 McGraw-Hill Education 64 25.6 Disturbances to Acid-Base Balance Learning Objectives: 2 41. Explain how metabolic acid-base disturbances differ from respiratory acid-base disturbances. 42. Define both metabolic acidosis and metabolic alkalosis, identify some of the causes of each type of acid-base disturbance, and explain how each occurs. 43. Describe renal and respiratory compensation. © 2019 McGraw-Hill Education 65 66 25.6a Overview of Acid-Base Imbalances Conditions caused by acid-base disturbance • Acidosis: arterial blood pH below 7.35 • Alkalosis: arterial blood pH above 7.45 Acid-base disturbance • Occurs when buffering capacity is exceeded • Transient change in H+, resulting in a change in pH beyond normal range • Buffering systems respond to offset disturbance • Response of physiologic buffering systems is called compensation • If these system are not effective, the disturbance is called uncompensated © 2019 McGraw-Hill Education 1 67 25.6a Overview of Acid-Base Imbalances Acid-base disturbance (continued) • Acid-base imbalance • Persistent pH change • Life-threatening for any extended period of time • Types of disturbances classified by • Whether the primary disturbance is respiratory or metabolic • Whether the pH change is acidic or alkaline • Four categories of acid-base disturbances 1. 2. 3. 4. © 2019 McGraw-Hill Education Respiratory acidosis Respiratory alkalosis Metabolic acidosis Metabolic alkalosis 2 25.6b Respiratory-Induced Acid-Base Disturbances 1 Respiratory acidosis • Most common acid-base disturbance • Due to impaired elimination of CO2 by the respiratory system • PCO2 in arterial blood is above 45 mm Hg • Accumulation of CO2 and subsequent increase in H+ concentration • Infants more susceptible • Due to smaller lungs and lower residual volume © 2019 McGraw-Hill Education 68 25.6b Respiratory-Induced Acid-Base Disturbances 2 Respiratory acidosis (continued) • Possible causes • Hypoventilation (breathing too slow/shallow) due to • Injury to respiratory center by trauma or infection • Disorders of muscles or nerves involved with breathing • Decreased airflow due to • Airway obstruction • Bronchitis or asthma • Impaired alveolar gas exchange due to • Reduced respiratory membrane surface area (e.g., emphysema) • Thickened respiratory membrane (e.g., pneumonia) © 2019 McGraw-Hill Education 69 25.6b Respiratory-Induced Acid-Base Disturbances 3 Respiratory alkalosis • PCO2 below 35 mm Hg due to hyperventilation (increase in breathing rate/depth) • Decrease of CO2 and lower H+ concentration • Possible causes • Severe anxiety • Hypoxia (insufficient oxygen delivery to cells) • valtitude, congestive heart failure, severe anemia • Aspirin overdose (stimulates respiratory center) © 2019 McGraw-Hill Education 70 71 Abnormal Respiration Rate and Blood pH Figure 25.15 © 2019 McGraw-Hill Education 25.6c Metabolic-Induced Acid-Base Disturbances 1 Metabolic acidosis • May occur from loss of HCO3– or gain of H+ • More commonly due to gain of H+ • H+ binding to HCO3–, decreasing levels • Occurs when levels drop below 22 mEq/L • Infants particularly susceptible • They produce larger amounts of acidic metabolic wastes • Possible causes • Increased production of metabolic acids • E.g., ketoacidosis from diabetes, lactic acid from glycolysis, acetic acid from excessive alcohol intake • Decreased acid elimination due to renal dysfunction • Increased elimination of HCO3– due to severe diarrhea © 2019 McGraw-Hill Education 72 25.6c Metabolic-Induced Acid-Base Disturbances 2 Metabolic alkalosis • Arterial blood levels of HCO3– above 26 mEq/L • From loss of H+ or increase of HCO3– • Possible causes • Vomiting (most common) • Increased loss of acids by kidneys with diuretic overuse • Large amounts of antacids © 2019 McGraw-Hill Education 73 Clinical View: Lactic Acidosis and Ketoacidosis Lactic acidosis • Acid-base disturbance caused from large amounts of lactic acid • Occurs when insufficient oxygen is available Ketoacidosis • Acid-base disturbance due to large amounts of ketoacids • Ketoacids produced from fat metabolism • If insulin insufficient (diabetes type 1) cells forced to use fat • Insufficient cellular glucose uptake © 2019 McGraw-Hill Education 74 75 25.6d Compensation 1 Renal compensation: physiologic adjustments of the kidney to changes in pH Response to elevated blood H+ • Due to a cause other than renal dysfunction • Type A intercalated cells • Excrete H+ and reabsorb HCO3– • Occurs to a greater degree than normal during compensation • Blood levels HCO3– high in compensation • Urine pH lower than normal © 2019 McGraw-Hill Education 76 25.6d Compensation 2 Renal compensation (continued) Response to decreased blood H+ • Type B intercalated cells • Reabsorb H+ and excrete HCO3– • Occurs to a greater degree than normal during compensation • Blood levels HCO3– low in compensation • Urine pH higher than normal • Generally effective in neutralizing acid-base disturbances not due to renal dysfunction © 2019 McGraw-Hill Education 77 25.6d Compensation 3 Respiratory compensation occurs in response to metabolic acidosis or alkalosis Respiratory rate increases as a result of increased H+ concentration • Higher than normal amounts of CO2 expired • Lower than normal blood PCO2 value Respiratory rate decreases as a result of decreased H+ concentration • Lower than normal amounts of CO2 expired • Higher than normal blood CO2 value • Limited by development of hypoxia Less effective than renal compensation © 2019 McGraw-Hill Education Clinical View: Arterial Blood Gas (ABG) and Diagnosing Different Types of Acid-Base Disturbances 1 ABG • Used to diagnosis and monitor acid-base disturbance and compensation • Include pH, arterial PCO2 and HCO3– © 2019 McGraw-Hill Education 78 79 Clinical View: Respiratory acidosis with renal compensation • Respiratory rate decreased and blood CO2 increased • Drives increase in H2CO3 • Kidneys compensate • Increased secretion of H+ and reabsorption of HCO3– • With compensation, H2CO3 and HCO3– both greater than normal Respiratory alkalosis with renal compensation • Respiratory rate increased and blood CO2 decreased • Drives decrease in H2CO3 • Kidneys compensate • Increased secretion of HCO3– and reabsorption of H+ • © 2019 McGraw-Hill Education With compensation, H2CO3 and HCO3– both lower than normal 80 Clinical View: Metabolic acidosis with respiratory compensation • Blood H+ increased • Decrease of HCO3– • Compensates with increased respiratory rate and lower CO2 • With compensation, H2CO3 and HCO3– both lower than normal Metabolic alkalosis with respiratory compensation • Blood H+ decreased • Increase of HCO3– • Compensates with decreased respiratory rate and higher CO 2 • With compensation, H2CO3 and HCO3– both greater than normal © 2019 McGraw-Hill Education Section 25.6 What did you learn? 81 1 21. How does a compensated acid-base disturbance differ from an uncompensated acid-base imbalance? 22. Describe the change (increases, decreases, or stays the same) for each of the following variables if an individual hyperventilates: (a) blood CO2, (b) blood H+ concentration, and (c) blood pH. 23. What is the primary cause of metabolic alkalosis? 24. How does renal compensation affect blood plasma levels of HCO3– ? How does respiratory compensation affect blood PaCO2? © 2019 McGraw-Hill Education Section 25.6 What did you learn? 82 2 25. Using the normal arterial blood gas values (pH = 7.35-7.45; PaCO2 = 35-45 mm Hg; HCO3– = 22-26 mEq/L), identify both the primary disturbance and the degree of compensation for these three arterial blood gas readings: • (a) pH = 7.20, PaCO2 = 35 mm Hg, [HCO3– ] = 17 mEq/L • (b) pH = 7.20, PaCO2 = 25 mm Hg, [HCO3– ] = 17 mEq/L • (c) pH = 7.36, PaCO2 = 20 mm Hg, [HCO3– ] = 17 mEq/L © 2019 McGraw-Hill Education Section 25.6 What did you learn? 83 3 26. Using the normal arterial blood gas values (pH = 7.35-7.45; PaCO2 = 35-45 mm Hg; HCO3– = 22-26 mEq/L), identify both the primary disturbance and the degree of compensation for these three arterial blood gas readings: • (a) pH = 7.20, PaCO2 = 50 mm Hg, [HCO3– ] = 24 mEq/L • (b) pH = 7.20, PaCO2 = 50 mm Hg, [HCO3– ] = 29 mEq/L • (c) pH = 7.36, PaCO2 = 50 mm Hg, [HCO3– ] = 30 mEq/L © 2019 McGraw-Hill Education